Abstract
Despite the use of cerium oxide nanoparticles (nCe) in some regions as a diesel fuel additive, the physicochemical properties of the resulting exhaust particles in the ambient atmosphere are not well known. The mixing state of ceria with other exhaust particles is one such physicochemical property that has been shown to potentially affect ecosystem/human health. In this study, cerium-containing particles associated with an nCe additive were collected in the laboratory and in Newcastle-upon-Tyne, UK where the local bus fleet uses the Envirox nCe additive. Electron microscopy of laboratory-generated exhaust samples indicated both individual ceria and soot particles (external mixture) and ceria contained within soot agglomerations (internal mixture). Low ambient concentrations prevented quantification of the ceria particle mixing state in the atmosphere; therefore, a multicomponent sectional aerosol dynamic model was used to predict the size, chemical composition, and mixing state of ceria particles as a function of distance from an idealized roadway. Model simulations predicted that most ceria particles remain nonmixed in the ambient atmosphere (300 m downwind from the roadway) due to slow coagulation, with the mixing rate most sensitive to the ceria content of emitted nuclei-mode particles and the particle concentration upwind of the road. Although microscopy analysis showed both external and internal mixtures of ceria and soot in freshly emitted particles, the ambient mass concentration, and size distribution of ceria particles predicted by the model was insensitive to the emitted mixing state.
Copyright 2015 American Association for Aerosol Research
INTRODUCTION
The use of engineered nanomaterials (ENMs) in consumer products highlights the need for quantification of their fate in the environment and the potential exposure of humans and ecological receptors (Majestic et al. Citation2010; Peralta-Videa et al. Citation2011). While the global production of cerium oxide (ceria) nanoparticles (nCe) is small relative to other ENMs like SiO2 and TiO2, its commercial use as a diesel fuel additive has led to concerns of near-road exposure. While reducing fuel consumption, soot, and CO2 emissions, nCe-based diesel fuel additives (which are commercially available under the brand names Eolys, Envirox, and Platinum Plus) change the physicochemical characteristics of exhaust particles and substantially increase the emission of cerium-containing particles to the atmosphere (Czerwinski et al. Citation2000; Skillas et al. Citation2000; Jung et al. Citation2005; Okuda et al. Citation2009; Zhang et al. Citation2013). The introduction in 2005 of an nCe additive to diesel fuel used by Stagecoach buses (Stagecoach Group plc) operating in Newcastle-upon-Tyne, UK led to a fivefold increase in ambient cerium mass concentrations (Park et al. Citation2008; Cassee et al. Citation2011). nCe-containing additives are not registered for on-road use in the U.S.; however, model predictions of a hypothetical scenario in which all on-road and nonroad diesel vehicles in the eastern U.S. began using nCe additives without diesel particulate filters show a 25-fold increase fine-particulate cerium emissions and ambient cerium mass concentrations as high as 22 ng m−3 (Erdakos et al. Citation2014). Although future changes in the usage and technology of diesel particulate filters may affect the magnitude of emissions associated with nCe additives, improved understanding their potential environmental/human health impacts is needed.
Reviews of the human and ecological impacts of cerium-containing nanoparticles (HEI Citation2001; EPA Citation2009; Batley et al. Citation2013; Perullini et al. Citation2013) find that the lack of consistent particle size and dosage amounts make comparison between studies difficult, but indicate that environmental concentrations are below toxic levels. Many of these reviews also state that the understanding of the surface reactivity, fate, transport, bioavailability, and toxicity of these particles is still in its infancy. A consistent observation among these studies is that the size of the ceria particle is inversely related to its potential toxicity due to smaller particles having a greater surface area to volume ratio (Yokel et al. Citation2014) and the ability to deposit deep within the lungs and to penetrate cells (see Perullini et al. Citation2013 and references therein). Limbach et al. (Citation2005) finds that uptake of ceria particles in human lung fibroblasts is directly related to size, with rapid agglomeration of smaller particles in biological systems limiting uptake. While no chronic inhalation studies of nCe toxicity in humans are available, EPA (Citation2009) summarizes an inhalation study using rats and ∼2000 nm diameter ceria particles which found that the inhalation reference concentration (defined as an estimate of a continuous inhalation exposure to the human population that is likely to be without an appreciable risk of deleterious effects during a lifetime) to be 900 ng m−3. In terms of ecosystem impacts, the expected ambient concentrations of ceria in freshwater environments (<1 μg L−1) are several orders of magnitude less than the concentration required for ecological risk (∼1 mg L−1) (Batley et al. Citation2013).
In addition to the particle size, ceria toxicity to human health and the environment is also related to its aggregation/interaction with soot particles (Batley et al. Citation2013). Ma et al. (Citation2014) finds that exposure to a mixture of nCe and diesel exhaust particles leads to increased lung injury in rats relative to exposure of diesel exhaust particles alone. Similarly, exhaust particles from nCe-doped diesel fuel has more adverse pulmonary effects on a mass basis than diesel exhaust particles without nCe additive usage (Snow et al. Citation2014). Jung et al. (Citation2005) observes that cerium-containing particles at the time of emission consist of cerium oxide nanoparticle aggregates that either decorate the surface of larger soot particles or exist as individual particles. Cassee et al. (Citation2012) and Batley et al. (Citation2013), on the other hand, characterize the ceria nanoparticle agglomerations as being within the soot matrix/clusters. Using an iron (Fe) fuel borne catalyst similar to the nCe-based additives, Nash et al. (Citation2013) reports that the fraction of Fe-containing exhaust particles unassociated with soot increases as the particle size decreases. A review of atmospheric metallic nanoparticles (Sanderson et al. Citation2014) suggests that the particle morphology/agglomeration of diesel fuel exhaust is affected by engine speed/load and additive doping levels.
Although several studies have examined the physicochemical properties of freshly emitted ceria particles from nCe additive use (Czerwinski et al. Citation2000; Skillas et al. Citation2000; Jung et al. Citation2005; Okuda et al. Citation2009; Zhang et al. Citation2013), the atmospheric evolution, transport, and fate of these particles have not been examined in detail. The properties of ambient ceria particles, rather than those of the nCe additive or freshly emitted particles, ultimately determine their potential to impact human health and the environment. In a companion paper, Gantt et al. (Citation2014) describes how the use of nCe additives modifies the size distribution and composition of exhaust particles in the near-road environment of Newcastle-upon-Tyne, UK. This study expands upon Gantt et al. (Citation2014) by examining multiple factors that affect the ambient physicochemical properties and atmospheric evolution of cerium-containing particles generated from the use of nCe additives. By supplementing laboratory- and field-based electron microscopy of cerium-containing particles with sensitivity simulations from a multicomponent sectional aerosol dynamic model, we were able to provide an indication of the atmospheric mixing state of ceria and how that mixing is affected by the emission intensity, meteorology, background particle concentration, and properties of the emitted particles.
METHODS
Laboratory and Ambient Observations
Gantt et al. (Citation2014) describes the Newcastle-upon-Tyne, UK measurement campaign which took place in August 2012 at the Automatic Urban and Rural Network (AURN) site near the Civic Center on St. Mary's Place (54.98°N, -1.61°W). Observations from that campaign include hourly black carbon (BC) concentrations, 12-h average chemically resolved PM10 (which contrast high-traffic periods from 8 AM to 8 PM with low-traffic periods from 8 PM to 8 AM), 7-day average size-resolved and chemically resolved PM (12 size bins from <10 to 18,000 nm in aerodynamic diameter), and hourly PM10 and PM2.5 mass concentrations. For all PM10 and size-resolved particle samples, 47 mm MTL (PT47P) and Zefluor (P5PJ037) PTFE filters were analyzed for total mass (gravimetric) and trace-metals concentration via inductively coupled plasma mass spectrometry (ICP-MS).
Concurrent with the ambient mass concentration measurements, electron microscopy of nCe-doped diesel exhaust particles from both laboratory and field settings was performed to characterize the presence and mixing state of nonvolatile particles such as ceria and soot. In the laboratory, exhaust particles from a diesel-powered electric generator (Yanmar Corp., model L70V) doped with 10 ppm Ce (by weight) from Envirox were sampled on polished silicon wafers that were 25.4 mm in diameter and 50 μm thick in a nanoMOUDI impactor and analyzed by scanning electron microscopy (SEM) using FEI Nova NanoLab 600 Focused Ion-Beam SEM (FEI, Hillsboro, OR, USA). The instrument was operated as a field-emission SEM with the beam energy at 5 keV and current at 0.4 nA. Secondary electron imaging (a and b) was performed with a through-the-lens scintillator detector, while the backscatter electron imaging (c and d) used a concentric backscatter detector configured to optimize compositional contrast. In the field, exhaust particles from the tailpipe of an idling Stagecoach bus using Envirox were collected with a passive sampler on a copper TEM grid and analyzed by scanning transmission electron microscopy (STEM) using a Hitachi HD-2300 (Hitachi Ltd., Japan). These tailpipe samples were intended to be representative of emissions from the Haymarket and Eldon Square bus stations within 0.5 km of the Newcastle AURN site. Additionally, holey carbon TEM grids were used to collect particles from five of the six smallest nanoMOUDI stage (particles between 56 and 320 nm aerodynamic diameter) from a sampler adjacent to the Newcastle AURN site. These grids were examined with a JEOL JEM-3011 high resolution TEM (JEOL Ltd., Japan).
FIG. 1. SEM (a, b) and associated backscattered SEM (c, d) images at 12,000× magnification of laboratory-generated soot and CeO2 particles and a single large soot particle with embedded CeO2 particles. The white box on the upper right of (a) is zoomed to 250,000× magnification in (b) and (c). White areas of the backscattered SEM images (c, d) represent CeO2. The sample was collected on a polished silicon wafer and analyzed with an FEI Nova NanoLab 600 Focused Ion-Beam SEM.
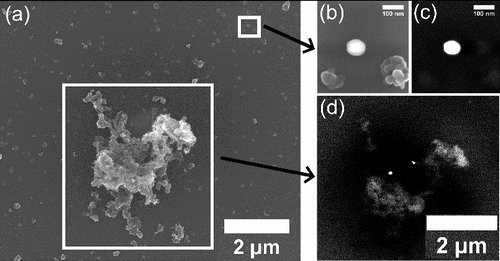
Model Configuration
An extension of a multicomponent sectional aerosol dynamic model for the numerical simulation of particle dynamics near roadways (Zhang and Wexler Citation2004; Zhang et al. Citation2004) was used to simulate the atmospheric evolution of cerium-containing particles. This model incorporated the physical processes of dilution, coagulation, condensation/evaporation, and deposition for particles emitted near an idealized roadway with uniform winds blowing perpendicular to the flow of traffic. The model explicitly tracked (as a function of distance from the roadway) the number concentration of particles with Stokes diameters ranging from 1 to 10,000 nm for five particle types: upwind background, nonceria exhaust, pure-ceria exhaust, a mixture of ceria/nonceria exhaust, and a coagulated mixture of ceria/nonceria exhaust with background particles. The ceria/nonceria exhaust particle type was directly emitted or formed via coagulation in the atmosphere, while the ceria/non-ceria exhaust/background particle type only existed as a result of coagulation in the atmosphere. Within each of these particle types, the model calculated the size-resolved mass concentration of semi-, low-, and nonvolatile organic material (OM), elemental carbon (EC; which also includes the “Other” category of Kleeman et al. [Citation2000]), and ceria. The ceria particle type, for example, contained both ceria and the semi- and low-volatile OM that condensed onto the ceria particle. See the supplemental material from Gantt et al. (Citation2014) for details.
Gantt et al. (Citation2014) describes the emission inputs to the model, which consist of size-resolved and chemically resolved mass and number concentrations of diesel exhaust emitted from vehicles with and without nCe additive usage. These inputs were generated from mean values of available laboratory measurements (Czerwinski et al. Citation2000; Skillas et al. Citation2000; Kleeman et al. Citation2000; Jung et al. Citation2005; Okuda et al. Citation2009) normalized to total roadside concentrations of 10.0 and 8.3 μg m−3 for the simulations with and without nCe additive usage, respectively. This 17% decrease in total particle mass emissions from nCe additive usage was based on an average of laboratory studies (Erdakos et al. Citation2014). Two model simulations (hereafter, “Default” and “CerAdd”) were performed in which the emitted particle mixing state, meteorological conditions, background particle size distribution, and amount of condensable organic gases in the exhaust and background atmosphere were each fixed to an assumed value. Those two simulations were designed to represent conditions dominated by exhaust from diesel vehicles without (Default) and with (CerAdd) the use of an nCe additive. Additional differences in the size distribution and chemical composition of exhaust particles were related to the impact of the nCe additive on diesel fuel combustion (see Gantt et al. Citation2014 for details).
In this study, we performed a series of sensitivity simulations to examine how the atmospheric evolution of exhaust particles responds to changes in extrinsic and intrinsic properties. The extrinsic sensitivity simulations included changes in the wind speed (“CerAdd-WS”), total mass concentration (a surrogate for emission intensity or traffic volume) at the roadside (“CerAdd-MConc”), background particle concentration upwind of the road (“CerAdd-BConc”). Uncertainties in the composition, size distribution, and mixing state of emitted exhaust particles associated with the use of an nCe additive in laboratory-based studies necessitated a set of intrinsic sensitivity simulations to determine the impact on ambient particles. These included a reduction of emitted nuclei mode ceria particles (“CerAdd-Nuc”), downward shift in the emitted exhaust particle size distribution through a reduction in supermicron particles (“CerAdd-Sup”), and the inclusion of internally mixed ceria particles at the time of emission (“CerAdd-Mix”). A summary of the variables of interest in each of the simulations is given in Table S1.
RESULTS AND DISCUSSION
Electron Microscopy
An analysis of laboratory-generated particles from nCe-doped diesel fuel exhaust in showed that ceria particles are emitted both as smaller particles composed mainly of CeO2 (c) and as aggregations of ceria on larger soot particles (d). is one of many SEM samples which found that the fraction of CeO2 associated with soot particles averaged ∼40%. c and d also indicate that the ceria particles were similar in size (∼75 nm diameter) whether emitted as individual particles or associated with larger soot particles; this particle size was similar to the peak (65 nm Stokes diameter) in the emitted ceria particle mass size distribution from the sectional aerosol model (Gantt et al. Citation2014). Furthermore, our tailpipe sample collected from an idling Stagecoach bus in Newcastle confirmed the emission of ceria particles from vehicles using the Envirox diesel additive (Figure S1). Ceria particles sampled from the tailpipe ranged from 5–100 nm in diameter, had both cubic (similar to CeO2 in the additive) and spherical morphologies, and were typically mixed with soot.
The cerium-containing particle mass at Newcastle has the following characteristics: (1) an average total (crustal + noncrustal) cerium concentration of ∼0.5 ng m−3, (2) a diurnal cycle matching the intensity of bus traffic in the region, and (3) a bimodal size distribution (peaks in the submicron and supermicron size ranges) with noncrustal cerium largely contained within a single submicron peak (Gantt et al. Citation2014). Samples from ambient nanoMOUDI stages with the highest estimated mass concentrations of noncrustal cerium as estimated by Gantt et al. (Citation2014) were subjected to TEM analysis in an effort to determine the composition and mixing state of cerium-rich particles in the atmosphere. Despite a meticulous search, few cerium-containing particles were identified in the ambient samples. The TEM image in a shows an elongated ceria particle with a diameter of about 12 nm, with the higher magnification in b revealing the particle's crystalline structure as indicated by the lattice fringes measuring 1.94 Å (consistent with the fluorite structure of ceria). Energy-dispersive x-ray spectroscopy (EDS) confirmed the particle to be ceria. Graphitic fringes were seen around the particle but not adjoining it, suggesting that it had not acted as a nucleation center for carbon deposition.
FIG. 2. TEM images of an elongated 12 nm CeO2 particle at 300,000× (a) and 800,000× (b) magnification sampled at Newcastle, UK on the 180–320 nm impaction stage of a nanoMOUDI with the circle denoting the approximate location of the EDS spectrum shown below. The sample was collected on a holey carbon film supported on a 300 mesh copper TEM grid and analyzed with a JEOL JEM-3011 high-resolution TEM operating at 300 kV. The source of the silicon peak in the TEM image is not known.
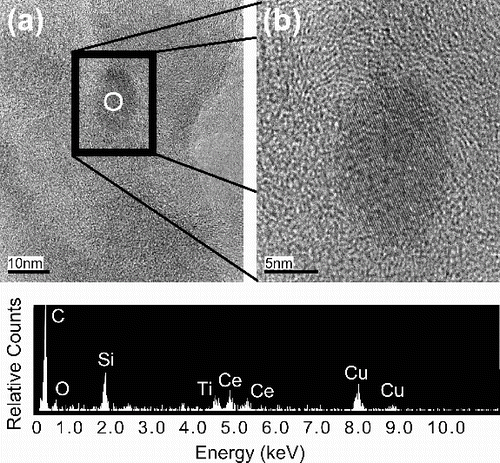
Based on the EDS spectrum, the particle shown in was noncrustal and likely generated by nCe additive use. It exhibited an elongated morphology, potentially related to the use of surfactants in the production of the nCe additive (Gnanam and Rajendran Citation2013). Scarcity of ceria particles in the ambient samples was expected, as their estimated number concentration during the Newcastle campaign (assuming CeO2 spheres) was ≪ 1 cm−3 (approx. 50 ppm). One cerium-containing particle (not shown), thought to be of crustal origin due to the copresence of iron and lanthanum, was identified by STEM analysis in contrast to the lack of submicron crustal particles modeled by Gantt et al. (Citation2014). It is worth noting that semivolatile components of the laboratory, tailpipe, and ambient particles were not observed by electron microscopy due to evaporation within the SEM/TEM vacuum. While the laboratory-generated and tailpipe images indicated some mixing with other particles types, the single ambient sample in did not give a quantitative determination of the mixing state of nonvolatile ceria and soot particles in the atmosphere; therefore, we used the near-roadway sectional aerosol model to identify potential factors affecting the mixing state.
Model Predictions
The sectional aerosol dynamic model results using emission inputs without (Default) and with (CerAdd) the nCe additive showed that the ambient (at 300 m) number concentration of exhaust particles was 80% lower than the roadside (at 30 m) concentration, mainly due to dilution and deposition (). The mass concentration of exhaust particles decreased <20% by 300 m from the roadway because condensational growth offset the initially rapid losses from dilution and to a lesser degree deposition (a and ). Predictions from the model suggested that the ambient ceria mass size distribution peaked at ∼150 nm in aerodynamic diameter, similar to the 200 nm peak observed in Newcastle for cerium-containing particles. Although the exhaust particle mass concentration gradually increased due to condensation of organic gases following the rapid dilution phase of the first 60 m, the mass concentration of nonvolatile ceria in the exhaust particles only decreased due to dilution and deposition (b).
TABLE 1 Summary of sensitivity simulation results from the sectional aerosol dynamics model as a function of distance from a roadway
FIG. 3. Mass concentration by distance of all exhaust particles (a) and ceria within the exhaust particles (b) for sensitivity simulations in which the roadside concentration is 8.3 μg m−3. Note that the CerAdd simulation described in Gantt et al. (Citation2014) is in bold and some of the sensitivity simulations are not visible because they have identical values to the CerAdd simulation.
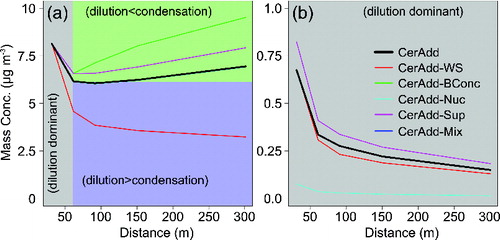
In addition to predicting the number and mass size distribution of ceria, the aerosol model also predicted the mixing state of ceria with both background and/or nonceria exhaust particles based on coagulation rates. Ceria particles in the CerAdd simulation were externally mixed with other exhaust particles at the time of emission, although observational evidence suggests that the ceria is neither completely externally or internally mixed with other exhaust particles (Jung et al. Citation2005). The coagulation timescale in the model was substantially larger than the dilution and condensation timescales; therefore, coagulation played a minor role in determining the ambient particle size distribution. Similarly, the slow rate of coagulation between ceria and other particles resulted in a slight decrease in the ceria particle type (nonmixed) fraction from 100% at 30 m to 88% at 300 m. Most of the coagulation involving ceria occurred within 60 m of the roadway because of the higher concentrations prior to dilution (). Condensation of organic gases also occurred on the non-mixed ceria particles in the model (only coagulation changes the particle type), leading to particle growth (see Figure 5 in Gantt et al. Citation2014).
Effect of Meteorology
The impact of wind speed in the aerosol model was tested in CerAdd-WS by doubling the wind speed from 1 to 2 m s−1 without changing other model parameters from CerAdd. In general, the faster dilution rates associated with higher wind speeds exceeded condensation rates and resulted in lower concentrations at all distances (a). Despite the decrease in ambient particle number concentration from faster dilution, doubling of the wind speed led to a much larger relative (to CerAdd) decrease in the exhaust particle mass concentration from 30 to 300 m by limiting condensational growth. Because the condensation rate was relatively constant (unlike dilution which occurs fastest within 60 m), exhaust particle mass concentration changes were more sensitive to wind speed than changes in the number concentration. In terms of ceria particles, doubling the wind speed to 2 m s−1 resulted in slightly lower mass concentrations at 300 m (0.13 μg m−3 compared to 0.15 μg m−3 for winds of 1 m s−1) due to more rapid dilution associated with higher wind speeds. Higher wind speeds also decreased the amount of mixing between ceria and other particle types, with 92% of the ceria at 300 m remaining nonmixed with winds of 2 m s−1 as compared to 88% for winds of 1.0 m s−1. Changing the meteorological/surface inputs in the model to resemble wintertime conditions with snow cover and an air temperature of 0°C had a minimal impact on the total exhaust and ceria particle mass concentrations and mixing rates.
Effect of Emitted Mass
To determine the changes that may occur due to higher vehicular emissions, the total roadside mass concentration CerAdd-MConc was doubled to a value of 16.6 μg m−3 without changing other model parameters such as emitted condensable organic gases. Changes in the total number concentration with distance for this case were similar to that of CerAdd and most other sensitivity simulations (with the exception of CerAdd-WS) where the percentage decrease from 30 to 300 m is 80%. Increased exhaust particle number concentration in CerAdd-MConc lead to greater competition for condensable gases, resulting in a proportionally larger decrease (19%) in total mass from 30 to 300 m than that of the CerAdd (14%) simulation. Doubling of the total exhaust particle mass lead to a proportional doubling of the ceria mass concentration at 30 and 300 m from the roadway, with an ambient mass concentration of 0.31 μg m−3. Although the concentration of non-mixed ceria in the CerAdd-MConc simulation was roughly double that of CerAdd, mixing processes were insensitive to the amount of ceria by the roadway as indicated by the similar relative fractions of ceria remaining nonmixed at 300 m (88%) in the two simulations.
Effect of Background Particle Concentration
Decreasing the background particle concentration from urban (1.4 × 105 cm−3) to rural (8.8 × 103 cm−3) values (Seinfeld and Pandis Citation2007) in CerAdd-BConc had a noticeable effect on the condensation and mixing of the exhaust particles. While the exhaust particle number concentrations were similar at all distances from the roadway for the urban and rural cases, the lower background particle concentrations allowed more of the organic gases (both emitted and background) to condense on the exhaust particles rather than the background particles. Instead of the 14% decrease in the predicted total particle mass by 300 m from the roadway with an urban background (CerAdd), the predicted mass in CerAdd-BConc increased by 17% when a rural background was used ( and a).
Like other exhaust particles, ceria particles were affected by the background particle concentration. Because fewer particles were available to coagulate with ceria when a rural background particle concentration was used, 99% (up from 88% in CerAdd) of the ceria particle type remained nonmixed at 300 m in CerAdd-BConc (). Reducing the background particle number concentration resulted in greater condensation of organic gases and increased OM mass onto the ceria particle type, shifting the geometric mean diameter these particles from 104 to 117 nm Stokes diameter (Figure S2). Using the Shen and Ring (Citation1986) method for distinguishing between two aerosol size distributions, we found that differences in the ceria mass size distributions from the CerAdd and CerAdd-BConc simulations were statistically significant with a p value <0.05. Despite the increase in ceria particle size, the ceria mass concentration within exhaust particles at 300 m in CerAdd-BConc was unchanged from the CerAdd simulation ( and b where CerAdd-BConc is hidden behind CerAdd). The introduction of sulfur-free diesel fuel and other air pollution controls has led to reductions in background particle number concentrations in many urban areas (Jones et al. Citation2012; Kumar et al. Citation2014a), suggesting that recent ceria particle mixing in the atmosphere may be better represented by CerAdd-BConc than CerAdd.
Effect of Intrinsic Particle Properties
In addition to environmental/meteorological conditions, the emitted physicochemical properties of exhaust particles from nCe-doped diesel fuel can affect their ambient properties. One such property with large uncertainties is the chemical composition of the smallest particles. In the CerAdd-Nuc simulation, the nuclei mode ceria particles <50 nm in diameter were mostly removed by assuming that these particles were 1.2% ceria by mass (down from the 100% value used in CerAdd and all other nCe simulations) as suggested by Okuda et al. (Citation2009). This large reduction of nuclei mode ceria particles lead to an 88% decrease in the bulk ceria content of emissions. Like the CerAdd simulation, ceria particles in the CerAdd-Nuc simulation were externally-mixed with other exhaust particles but with ∼99% of the nuclei mode mass composition changed from ceria to either EC, OM, or Other according to the Kleeman et al. (Citation2000) ratios (Figure S3). The particles formerly composed of ceria were also reclassified to the nonceria exhaust particle type in the CerAdd-Nuc simulation. The large reduction in the ceria content of the smallest particle sizes lead (not surprisingly) to a decrease in the roadside and ambient ceria mass concentration by nearly an order of magnitude (0.08 and 0.02 μg m−3 for roadside and ambient conditions, respectively) relative to CerAdd (0.68 and 0.15 μg m−3). Although the ceria that remained in the CerAdd-Nuc simulation is not mixed with other exhaust particles at the time of emission, the large decrease nuclei mode ceria particles and corresponding increase in nonceria particles enhanced the coagulation efficiency of the ceria particle type such that only 11% of these particles remained nonmixed at 300 m ().
The supermicron size distribution of nCe-doped diesel fuel exhaust particles used in the model was also uncertain, as few of the published laboratory studies measure particles in this size range. The mass reduction of supermicron exhaust particles in CerAdd-Sup to match that of the Default simulation (see in Gantt et al. Citation2014) lead to a shift in the size distribution such that the peak mass concentration of accumulation mode particles increased by >40%. Despite having the same total mass concentration as CerAdd (8.3 μg m−3), the CerAdd-Sup simulation had a higher total particle number concentration (49480 vs. 42240 cm−3 in CerAdd) due to the increase in particles ∼100 nm in diameter. This sensitivity study captured the higher particle number and lower mass emissions observed in laboratory studies when an nCe additive is used (Skillas et al. Citation2000; Zhang et al. Citation2013). The modified particle size distribution in CerAdd-Sup resulted in a slightly different atmospheric evolution of the exhaust particles; more numerous 50–100 nm diameter particles and fewer supermicron particles enhanced condensation and to a lesser degree reduced deposition such that mass concentrations at 300 m from the roadway were higher than CerAdd (a). Despite the large size distribution change in CerAdd-Sup, the impact on the mass concentration at 300 m was smaller than that of environmental changes in the CerAdd-WS and CerAdd-BConc simulations. Higher submicron exhaust particle concentrations in CerAdd-Sup also lead to increased ceria mass concentrations at 30 (0.83 μg m−3) and 300 (0.19 μg m−3) meters from the roadside, although the ratios of roadside and ambient concentrations are similar for the two cases. The fraction of non-mixed ceria was the same in CerAdd and CerAdd-Sup, indicating that the mixing of ceria and other exhaust/background aerosols was insensitive to the size distribution of supermicron exhaust particles.
Due to observational evidence ( and ) that ceria can be associated with soot particles at the time of emission, a sensitivity simulation was conducted in which ceria and other exhaust particles were internally-mixed at the time of emission (CerAdd-Mix). This assumption of an internal mixture of emitted ceria and other exhaust particles had little effect on the mass concentration of total exhaust particles and ceria at 30 and 300 m relative to CerAdd (see b where CerAdd-Mix is hidden behind CerAdd). The emitted mixing state did affect the ambient mixing state; however, as none of the ceria particles in CerAdd-Mix were nonmixed at 30 and 300 m. The CerAdd-BConc and CerAdd-Mix simulations represented the upper and lower limit of ceria particle mixing rates, and comparing the two () showed that the emitted mixing state had a large impact on the ambient mixing state but did not impact the ambient mass concentration of ceria-containing particles.
CONCLUSIONS
The physicochemical properties (such as the size and mixing state) of cerium-containing particles have been shown to affect their potential reactivity and toxicity to the environment and human health (Yokel et al. Citation2014; Kumar et al. Citation2014b). Low ambient concentrations at Newcastle-upon-Tyne, UK inhibited a complete characterization of the mixing state of nonvolatile particles such as ceria by electron microscopy; therefore, a near-roadway sectional model was used to determine the factors that affect the mixing state of cerium-containing particles associated with nCe additive usage. Sensitivity simulations indicate that the concentration of particles immediately upwind of an idealized roadway and the emitted composition of the nuclei-mode particles (<50 nm in diameter) had the largest effect on the mixing state of ambient ceria particles. Specifically, ambient ceria particles were predicted to be mixed to a greater degree with other exhaust and background particles when the emissions were injected into an atmosphere with high background particle concentrations (e.g., urban or industrialized area) or when the emissions of nuclei mode ceria particles were mostly removed. A reduction of the background aerosol concentration enhanced condensation of organic gases onto ceria particles, resulting in particle growth in the atmosphere. The dependence of ceria particle size and mixing state on the background aerosol concentration suggests that the location where nCe additives are used can strongly affect the ambient properties of the cerium-containing particles.
Due to the large fraction of ceria emitted in the nuclei mode and slow coagulation with exhaust and background particles within 300 m in most simulations, the potentially high ambient number concentration of nonmixed ceria particles may have a greater human health and ecosystem impact than the observed low mass concentrations (∼0.5 ng m−3 in Newcastle, UK) would indicate. Ambient ceria particles may have lower human health/ecosystem impacts than freshly emitted particles due to particle growth and mixing in the atmosphere. Model-predicted condensation of organic gases onto non-mixed ceria particles in the ambient atmosphere also suggests that their chemical properties may differ from those of freshly combusted particles (Lee and Donahue Citation2011). The low ambient number concentration of ceria particles and associated challenges with TEM and STEM analyses may necessitate instrumentation such as the single particle ICP-MS (Laborda et al. Citation2014), which can detect cerium nanoparticles as small as 10 nm in diameter (Lee et al. Citation2014), to better quantify their physicochemical properties.
SUPPLEMENTAL MATERIAL
Supplemental data for this article can be accessed on the publisher's website.
UAST_1027809_Supplemental_Files.zip
Download Zip (629.2 KB)ACKNOWLEDGMENTS
The authors gratefully acknowledge the assistance of Charly King and Todd Krantz of the United States Environmental Protection Agency (US EPA) and David Nash of Arcadis, Inc., for assistance with the laboratory generation and collection of cerium-doped diesel exhaust samples. We also acknowledge Richard Billings and Joe Fanjoy from ERG, Geoff Dollard and Steve Telling from AEA Technologies, and Michele Conlon, Gary Norris, and William Benjey from EPA for their administrative input.
FUNDING
The US EPA through its Office of Research and Development funded and managed the research described here under Contracts EP-12-D-000128 to RJ Lee Group, Inc., EP-D-11-006 to Eastern Research Group, Inc. (ERG), and a U.S. government interagency agreement DW-13-92339401-0. This article has been subjected to the Agency's administrative review and approved for publication. Brett Gantt and Shamia Hoque are supported by an appointment to the Research Participation Program at the Office of Research and Development, US EPA, administered by ORISE.
REFERENCES
- Batley, G. E., Halliburton, B., Kirby, J. K., Doolette, C. L., Navarro, D., McLaughlin, M. J., et al. (2013). Characterization and Ecological Risk Assessment of Nanoparticulate CeO2 as a Diesel Fuel Catalyst. Environ. Tox. Chem., 32:1896–1905.
- Cassee, F. R., van Balen, E. C., Singh, C., Green, D., Muijser, H., Weinstein, J., et al. (2011). Exposure, Health and Ecological Effects Review of Engineered Nanoscale Cerium and Cerium Oxide Associated With Its Use as a Fuel Additive. Crit. Rev. Tox., 41:213–229.
- Cassee, F. R., Campbell, A., Boere, A. J. F., McLean, S. J., Duffin, R., Krystek, P., et al. (2012). The Biological Effects of Subacute Inhalation of Diesel Exhaust Following Addition of Cerium Oxide Nanoparticles in Atherosclerosis-Prone Mice. Environ. Res., 115:1–10.
- Czerwinski, J., Napoli, S., Comte, P., Matter, U., and Mosimann, T. (2000). Investigations with the CORNING - Diesel Particulate Filter and Cerium- Additive Eolys DPX 9 on the Liebherr D914T Engine, Technik Thermische Maschinen, Neiderrohrdorf/Schweiz. VERT report: 1–13.
- Erdakos, G. B., Bhave, P. V., Pouliot, G. A., Simon, H., and Mathur, R. (2014). Predicting the Effects of Nanoscale Cerium Additives in Diesel Fuel on Regional-Scale Air Quality. Environ. Sci. Technol., 48:12775–12782.
- Gantt, B., Hoque, S., Willis, R. D., Fahey, K. M., Delgado-Saborit, J. M., Harrison, R., et al. (2014). Near-Road Modeling and Measurement of Cerium-Containing Particles Generated by Nanoparticle Diesel Fuel Additive Use. Environ. Sci. Technol., 48(21):10607–10613.
- Gnanam, S. and Rajendran V. (2013). Influence of Various Surfactants on Size, Morphology, and Optical Properties of CeO2 Nanostructures via Facile Hydrothermal Route. J. Nanopart., 839391.
- HEI (Health Effects Institute). (2001). Evaluation of Human Health Risk from Cerium Added to Diesel Fuel. HEI Communication 9. Flagship Press, North Andover, MA. (available online at http://pubs.healtheffects.org), pp. 1–64.
- Jones, A. M., Harrison, R. M., Barratt, B., and Fuller, G. (2012). A Large Reduction in Airborne Particle Number Concentrations at the Time of the Introduction of “Sulphur Free” Diesel and the London Low Emission Zone. Atmos. Environ., 50:129–138.
- Jung, H. J., Kittelson, D. B., and Zachariah, M. R. (2005). The Influence of a Cerium Additive on Ultrafine Diesel Particle Emissions and Kinetics of Oxidation. Combust. Flame, 142:276–288.
- Kleeman, M. J., Schauer, J. J., and Cass, G. R. (2000). Size and Composition Distribution of Fine Particulate Matter Emitted From Motor Vehicles. Environ. Sci. Technol., 34:1132–1142.
- Kumar, P., Morawska, L., Birmili, W., Paasonen, P., Hu, M., Kulmala, M., et al. (2014a). Ultrafine Particles in Cities. Environ. Intl., 66:1–10.
- Kumar, A., Das, S., Munusamy, P., Self, W., Baer, D. R., Saylee, D. C., et al. (2014b). Behavior of Nanoceria in Biologically-Relevant Environments. Environ. Sci.: Nano. 1:516–532.
- Laborda, F., Bolea, E., and Jimenez-Lamana, J. (2014). Single Particle ICPMS: A Powerful Tool for Nano-Analysis. Anal. Chem., 86:2270–2278.
- Lee, J. and Donahue, N. M. (2011). Secondary Organic Aerosol Coating of Synthetic Metal-Oxide Nanoparticles. Environ. Sci. Technol., 45:4689–4695.
- Lee, S., Bi, X., Reed, R. B., Ranville, J. F., Herckes, P., and Westeroff, P. (2014). Nanoparticle Size Detection Limits by Single Particle ICP-MS for 40 Elements. Environ. Sci. Technol., 48:10291–10300.
- Limbach, L. K., Li, Y. C., Grass, R. N., Brunner, T. J., Hintermann, M. A., Muller, M., et al. (2005). Oxide Nanoparticles Uptake in Human Lung Fibroblasts: Effects of Particle Size, Agglomeration, and Diffusion at Low Concentrations. Environ. Sci. Technol., 39:9370–9376.
- Ma, J. Y., Young, S. H., Mercer, R. R., Barger, M., Schwegler-Berry, D., Ma, J. K., et al. (2014). Interactive Effects of Cerium Oxide and Diesel Exhaust Nanoparticles on Inducing Pulmonary Fibrosis. Toxicol. Appl. Pharmacol., 278:135–147.
- Majestic, B. J., Erdakos, G. B., Lewandowski, M., Oliver, K. D., Willis, R. D., Kleindienst, T. E., et al. (2010). A Review of Selected Engineered Nanoparticles in the Atmosphere, Sources, Transformations, and Techniques for Sampling and Analysis. Intern. J. Occu. Environ. Health, 16:488–507.
- Nash, D. G., Swanson, N. B., Preston, W. T., Yelverton, T. L. B., Roberts, W. L., and Wendt, J. O. L. (2013). Environmental Implications of Iron Fuel Borne Catalysts and Their Effects on Diesel Particulate Formation and Composition. J. Aerosol. Sci., 58:50–61.
- Okuda, T., Schauer, J. J., Olson, M. R., Shafer, M. M., Rutter, A. P., Walz, K. A., et al. (2009). Effects of a Platinum-Cerium Bimetallic Fuel Additive on the Chemical Composition of Diesel Engine Exhaust Particles. Energy Fuels, 23:4974–4980.
- Park, B., Donaldson, K., Duffin, R., Tran, L., Kelly, F., Mudway I., et al. (2008). Hazard and Risk Assessment of a Nanoparticulate Cerium Oxide-Based Diesel Fuel Additive – A Case Study. Inhal. Tox. 20:547–566.
- Peralta-Videa, J. R., Zhao, L., Lopez-Moreno, M. L., de la Rosa, G., Hong, J., and Gardea-Torresdey, J. L. (2011). Nanomaterials and the Environment: A Review for the Biennium 2008–2010. J. Haz. Mater., 186:1–15.
- Perullini, M., Aldabe Bilmes, S. A., and Jobbágy, M. (2013). Cerium Oxide Nanoparticles: Structure, Applications, Reactivity, and Eco-Toxicology Nanomaterials: A Danger or a Promise? Springer, London.
- Sanderson, P., Delgado-Saborit, J. M., and Harrison, R. M. (2014). A Review of Chemical and Physical Characterisation of Atmospheric Metallic Nanoparticles. Atmos. Environ., 94:353–365.
- Seinfeld, J. H. and Pandis, S. N. (2007). Atmospheric Chemistry and Physics: From Air Pollution to Climate Change. Wiley, New York.
- Shen, A,-T. and Ring, T. A. (1986). Distinguishing Between Two Aerosol Size Distributions. Aerosol Sci. Technol., 5:477–482.
- Skillas, G., Qian, Z., Baltensperger, U., Matter, U., and Burtscher, H. (2000). The Influence of Additives on the Size Distribution and Composition of Particles Produced by Diesel Engines. Combust. Sci. Technol., 154:259–273.
- Snow, S. J., McGee, J., Miller, D. B., Bass, V., Schladweiler, M. C., Thomas, R. F., et al. (2014). Inhaled Diesel Emissions Generated With Cerium Oxide Nanoparticle Fuel Additive Induce Adverse Pulmonary and Systemic Effects. Toxicol. Sci., 2014:1–15.
- US EPA (U.S. Environmental Protection Agency). (2009). Toxicological Review of Cerium Oxide and Cerium Compounds. Available online at http://www.epa.gov/iris/toxreviews/1018tr.pdf.
- Yokel, R. A., Hussain, S., Garantziotis, S., Demokritou, P., Castranova, V., and Cassee, F. R. (2014). The Yin: An Adverse Health Perspective of Nanoceria: Uptake, Distribution, Accumulation, and Mechanisms of its Toxicity. Environ. Sci.: Nano., 1:406–428.
- Zhang, K. M., and Wexler, A. S. (2004). Evolution of Particle Number Distribution Near Roadways—Part I: Analysis of Aerosol Dynamics and Its Implications for Engine Emission Measurement. Atmos. Environ., 38:6643–6653.
- Zhang, K. M., Wexler, A. S., Zhu, Y. F., Hinds, W. C., and Sioutas, C. (2004). Evolution of Particle Number Distribution Near Roadways. Part II: The ‘Road-to-Ambient’ Process. Atmos. Environ., 38:6655–6665.
- Zhang, J., Nazarenko, Y., Zhang, L., Calderon, L., Lee K. B., Garfunkel, E., et al. (2013). Impacts of a Nanosized Ceria Additive on Diesel Engine Emissions of Particulate and Gaseous Pollutants. Environ. Sci. Technol., 47:13077–13085.