ABSTRACT
Tobacco columns are extreme examples of heterogenous packed beds, which have various cut-filler shapes and a wide range of size distribution. The behavior of mechanical filtration through tobacco columns has been investigated by using polystyrene latex (PSL) standard particles to compare the actual filtration efficiency with the predicted filtration efficiency calculated by theoretical equations for spherical packed beds. The influence of cut-filler shape and the range of cut-filler size distribution on filtration efficiency have been examined. The effect of diffusion in tobacco columns was lower and the effect of interception and inertia were higher than in spherical packed beds. These results show that a partially faster flow could have occurred in tobacco columns. It means that it is difficult to utilize the theoretical equations for spherical packed beds to heterogenous packed beds as proposed. Filtration efficiency through tobacco columns had a relationship with the factor that shows cut-filler shape and size distribution (r = 0.894, p < 0.05) and the factor that shows cut-filler size distribution (r = 0.683, p < 0.15). The factor showing cut-filler shape and size distribution was expected to be an effective factor of filtration efficiency for heterogenous packed beds. From these experiments, empirical equations that can be applied to tobacco columns have been proposed, and the prediction accuracy during burning was validated. It has been found that the prediction accuracy was precise, revealing the importance of taking the influence of cut-filler shape and size distribution into account in the filtration equations.
Copyright © 2016 American Association for Aerosol Research
EDITOR:
Introduction
Tobacco smoke consists of thousands of chemical components (Dube and Green Citation1982) including aroma and flavor components. Recently, there have been numerous tobacco products that have featured aromas and tastes. It is becoming important to estimate the yield of not only tar and nicotine, but also semi-volatile components related to aromas and tastes. In general, since semi-volatile components are distributed in gas and particle phases, it is essential to understand the respective behaviors of gases and particles. Tobacco columns have a significant impact on smoke yields because there are various phenomena. Examples of such phenomena include filtration, volatilization, condensation, gas diffusion, etc. The most effective phenomenon on yields is filtration, which has been reported by numerous researchers in tobacco filter studies. Keith Citation(1978) proposed theoretical filtration equations of acetate filters in consideration of fiber diameter, weight, orientation, and agglomerating property. Inagaki et al. Citation(2003) claimed that the influences of fiber crimp angle and fiber cross-sectional shape have a large impact on mechanical filtration efficiency. There has been significant discussion about tobacco filters, on the other hand, there have been a few reports on tobacco columns. One of the reasons for the limited research on tobacco columns seems to be the difficulty of constructing theoretical equations and numerical simulations. The greatest features of tobacco columns are cut-filler shape heterogeneity and a wide range of cut-filler size distribution as shown in . The figure shows the difficulty of defining the shape and particle size, in other words, tobacco columns are one of the extreme examples of heterogenous packed beds. We suppose that effective and realistic ways to investigate mechanical filtration through heterogenous packed beds could be an experimental approach. Kaneki and Tokida Citation(1991) conducted measurements of the pressure drop and tar and nicotine filtration efficiency during burning so as to examine the shape factor of tobacco columns and the particle diameter of tobacco smoke. The tobacco smoke diameter was discussed in the article; however, the relationship between the shape factor and filtration efficiency was not researched. Byckling Citation(1976) examined the effects of column length and packing density on the mechanical filtration of tar and nicotine through tobacco columns during burning. Theoretically, since tar and nicotine are condensable, it is not appropriate to use these components for the study of mechanical filtration. Therefore, the previous studies on tobacco column filtration have mixed with mechanical filtration and condensation on cut-fillers. There are no reports on purely mechanical filtration through tobacco columns.
Figure 1. A scanned image of the cut-filler shape and size in a tobacco column used in this study. The cut-fillers were scanned by a printer (Hewlett Packard, Palo Alto, CA, USA, C5280) and analyzed by an image processing software (MathWorks Inc., Natick, MA, USA, Matlab).
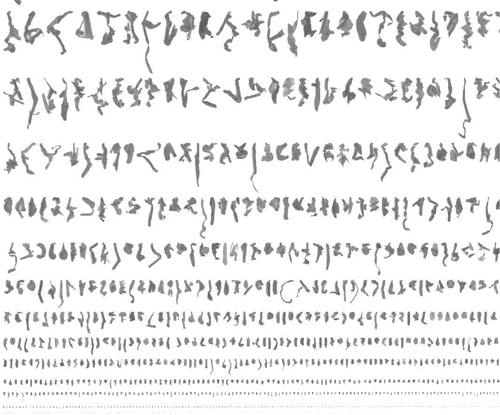
Meanwhile, studies on mechanical filtration through granular packed beds have been investigated for industrial applications. Numerous theoretical and empirical equations of filtration efficiency have been proposed (George and Poehkein Citation1974; Lee and Gleseke Citation1979; D'Ottavio and Goren Citation1983; Kimura et al. Citation1983a; Mann and Goren Citation1984; Mori et al. Citation1985; Otani et al. Citation1989). These studies targeted gravel, silica sand, grass beads, and several other granules with a spherical shape, and the experiments were conducted with uniform particle sizes. There are a few reports on the influence of the particle size distribution of aerosols on the filtration efficiency (Jung and Tien Citation1992; Veerapaneni and Wiesner Citation1993), on the other hand, there have been no reports on the simultaneous influence of granular shape and size distribution. Previously, the influence of granular shape on filtration efficiency has been reported (Kimura et al. Citation1983b). They found that filtration efficiency was increased with the degree of sphericity. Choi et al. Citation(2014) investigated the influence of the granular size and its distribution on filtration efficiency and pressure drop. However, their approaches could not focus on the relationship between heterogeneity and filtration efficiency. Since the cut-fillers in tobacco columns are crushed in the manufacturing process, the cut-filler shape and size are not uniform. Hence, there is a possibility that tobacco columns have a different filtration behavior from other uniform packed beds.
In this study, the applicability of filtration equations for spherical packed beds to tobacco columns has been investigated by comparing filtration efficiency between the experimental results and the theoretical equations for spherical packed beds. Furthermore, the simultaneous influence of the cut-filler shape and size distribution on filtration efficiency has been examined. From these results, the modified filtration equations for tobacco columns have been proposed and the validity of the filtration equations during burning has been investigated to compare with the experimental results and the predicted filtration efficiency. Although previous research on mechanical filtration used model particles in their experiments, information on the applicability of constructed equations to aerosols generated from combustion is rare. One of the purposes in this study is to check the usefulness of model experiments and constructed filtration equations to commercial tobacco products.
Experimental
Sample information
Six cigarette samples were selected from the Japanese market with the physical parameters listed in . The samples were weighed and the pressure drop was measured so as to set the average target after storing for 48 h under a temperature of 22 ± 1°C and a humidity of 60 ± 5%. They were sorted toward the target within a range of ±10 mg (weight) and ± 50 Pa (pressure drop), which is likely equal to the standard deviation. The cigarette paper was sealed with cellophane tape to eliminate the effect of air dilution through the cigarette paper except for puff-by-puff measurements. The packing density was estimated by net tobacco weight, the superficial volume inside the cigarette, and apparent density determined through the mercury intrusion technique (Quantachrome, Kanagawa, Japan, Poremaster).
Table 1. The physical properties of sample cigarettes.
Experimental apparatus and procedure
shows the experimental setup for mechanical filtration efficiency in this study. Polystyrene latex (PSL, JSR, Ibaraki, Japan, size standard particles 55, 101, 202, 300, 402, 505, and 602 nm) particle generation as monodispersed aerosols was carried out by an atomizer (TSI, Model 3079), and the particles were passed through a diffusion dryer (TSI, St. Paul, MN, USA, Model 3062) and a neutralizer (Kanomax, Osaka, Japan, Am-241) in an equilibrium charge state. The air volume was considered to be tobacco smoking conditions in a range of 0.4–2.5 L/min. The particle concentration was measured with SMPS (scanning mobility particle sizer, TSI, CPC: Model 3022A, DMA: Model 3080) and the filtration efficiency was determined by the concentration gap between with or without tobacco columns. After drying the particles in the diffusion dryer, the particle size distribution had a small peak around 50 nm as a residue of moisture evaporation. However, the influence on filtration efficiency was considered negligible because the concentration was an order of magnitude lower than PSL concentration. PSL particles were passed through tobacco columns after 20 min of generation so as to maintain a steady state. SMPS measurements were carried out after a minute of passing through tobacco columns, and the scanning time was 6 min (up-scan; 2 min, down-scan; 1 min, and interval; 3 min). Measurements were conducted three or four times per cigarette, and filtration efficiency was determined for four or five cigarettes. The error bars in the figures express the standard deviation of the measurement value in this study.
Method for measuring filtration efficiency during burning
The measurements of filtration efficiency during burning were calculated from the solanesol decay rate between a short cigarette (butt length: 10 mm) and a cigarette with a particular butt length in puff-by-puff measurements. SMPS measurements were not implemented due to the high concentration of tobacco smoke. A schematic drawing of the experiments is shown in and the equation for filtration efficiency is shown in the following:
It has been recognized that solanesol is one of the components that distribute mostly in the particle phase in tobacco smoke because of the low vapor pressure. Tang et al. Citation(1990) reported that solanesol is a suitable tracer component even in environmental tobacco smoke. Therefore, solanesol in the particles is expected to be generated immediately by homogenous and/or heterogenous nucleation within a short distance (<10 mm) at the top of the cigarette after burning. In addition, since the solanesol amount is sufficient to quantify in the tobacco smoke and the quantitative process is not complicated, it is suitable as being representative of particle-phase components. It should be noted that the calculation of filtration efficiency derived from solanesol yield corresponds to the efficiency of the mass mean particle diameter. Our samples have the peak diameter of smoke at 150–200 nm on a number basis, which correspond to 300–400 nm on a mass basis. Therefore, we examined in advance filtration efficiencies between PSL particles (200 nm and 400 nm) and solanesol with respect to filtration length.
The puff-by-puff measurements were performed by a smoking machine (Borgwaldt, Hamburg, Germany, RM20D), and five cigarettes were used each time. Before the measurements, the tobacco filters were removed from the cigarette in order to evaluate the filtration efficiency of tobacco columns. The generated smoke was collected on a Cambridge pad (Borgwaldt, 44 mmφ), and the number of repetitions was three. Three types of smoking conditions were carried out: (1)14 mL/2 s (interval: 58 s); (2) ISO condition: 35 mL/2 s (interval: 58 s); and (3) Health Canada Intensive condition (HCI): 55 mL/2 s (interval: 28 s). In the puff-by-puff measurements, the filtration lengths after puffing were captured on video (SONY, Tokyo, Japan, CX550V) and the positions were analyzed by the application of video image analysis (MITANI, Tokyo, Japan, Win-roof Version 6. 3.1). This method improved the prediction accuracy because the effective filtration length was more precise than previously. In the smoking experiments, since the cigarette paper was not sealed with cellophane tape, the combustion weight at the top of the cigarettes was decreased due to ventilation through the cigarette paper. In cases where the cigarette has a long butt length and high porosity of the cigarette paper, the ventilation ratio at first puff through the cigarette paper could exceed 15% calculated by equations from Futamura et al. Citation(1991). If the ventilation ratio through the cigarette paper exceeded 5%, the influence on the combustion yield was not negligible. In such a case, the solanesol yield from the combustion was corrected by the relationship examined in advance between the solanesol generation ratio and the air ventilation ratio.
Solanesol, nicotine, and tar analysis
The analysis method for solanesol was referred from a method (CORESTA Citation2002) from one of the academic communities: CORESTA. In the analysis, the collected particles on Cambridge filters were extracted in methanol solvent with 60 min of shaking, and the extraction liquid was filtered by syringe filters. The quantitative analysis was performed by HPLC (high performance liquid chromatograph, Agilent, California, USA, 1100 series) equipped with a Delta bond C18 ODS (Octadecylsilane) column (Keystone Scientific, Tokyo, Japan; 250 mm × 3.0 mm id, pore size 300 Å, and particle size 5 μm). The mobile phase composition consisted of acetonitrile and methanol (95:5) under the flow condition of 0.5 mL/min. The detector was PDA (Photodiode Array Detector, Agilent, 1100 series, G1315B) and the wavelength for solanesol was set at 205 nm. The reagent-grade solanesol (Tokyo Chemical Industry, Tokyo, Japan, S0525) was used in this experiment.
Nicotine and water were analyzed by GC (gas chromatography, Agilent, 7890A) coupled with a flame ionization detector and a thermal conductivity detector after the extraction of Cambridge filters in methanol solvent with 40 min of shaking. DB-WAX (Agilent, length: 30 m, inner diameter: 0.53 mm, thickness: 1 μm) and Porapak Q (Agilent, length: 1.83 m, mesh: 80 to 100) columns were equipped with the detectors, respectively. The carrier gas was helium at the flow volume of 3 mL/min. The injector and detector temperatures were set at 250°C and the oven temperature was set at 160°C. Generally, tar is defined as the portion of TPM (total particulate matter) retained on a Cambridge filter minus water and nicotine.
Pressure drop measurements and sieve measurements
Pressure drop measurements were carried out by an instrument (TAMAOKI works Ltd., Kanagawa, Japan, Air flow resistance meter), changing the air volume from 0.4 L/min to 2.5 L/min. Sieve measurements were conducted by JEL-200 (Borgwaldt, JEL-200). The sieve sizes were separated into seven categories (3.35 mm, 2.86 mm, 2.03 mm, 1.55 mm, 1.29 mm, 0.95 mm, and 0.71 mm). In the measurements, the cut-filler weight was 25 g each time, and the operating time was set at 2 min at 150 rpm. The particle size distribution and the average granule size were analyzed from the cut-filler weight for each sieve size.
Results and discussion
Model particles of tobacco smoke
It is essential that the model particles simulate tobacco smoke behavior so as to investigate mechanical filtration through tobacco columns. In this study, PSL particles were selected as the model particles; however, there has been little investigation on the model particles of tobacco smoke. Hence, a comparison of filtration efficiency along with the tobacco column length between tobacco smoke (smoking condition; 35 mL/2 s (interval: 58 s)) and PSL particles (flow condition: 1.05 L/min that is related to 35 mL/2 s smoking condition) was performed to verify the validity of using PSL particles. shows the logarithmic penetration ratio along with the tobacco column length with commercial brand A. The first puff of the smoking was removed from the results owing to an ignition effect on the puff. The slope between points shows the strength of the filtration. The high slope means that the strength of filtration efficiency is high.
Figure 4. Comparison results between the PSL logarithmic penetration ratio and the smoke components logarithmic penetration ratio.
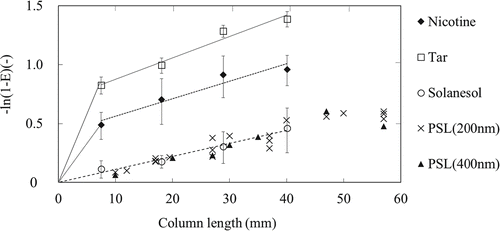
As shown in , the filtration behavior of PSL 200 nm has resulted in the same level of solanesol. Meanwhile tar and nicotine have indicated a high filtration efficiency, suggesting tar and nicotine are condensable lengthways in comparison with solanesol. Therefore, it is difficult to investigate mechanical filtration with tar and nicotine because they are highly condensated on cut-fillers. Using the standard particles or stable components like solanesol, which immediately condense and mainly distribute to the particle phase must be important to examine mechanical filtration. It should be noted that PSL filtration efficiency is consistent with solanesol in this experiment. Since there was no significant difference in filtration efficiency between PSL particles (200 nm and 400 nm) and solanesol under the ISO condition, the filtration efficiency derived from solanesol can be applicable to that of PSL particles (200–400 nm).
Filtration equations for tobacco columns
Comparison of previous research on filtration models for spherical packed beds
In order to investigate the suitability of the filtration equations for spherical packed beds reported by previous research (Lee and Gieseke Citation1979; Kimura et al. 1983; Mann and Goren Citation1984; Mori, et al. Citation1985; Otani et al. Citation1989) to tobacco columns, a comparison study on filtration efficiency between the predicted value by the research and the experimental value was carried out. It is assumed that the collection mechanism through tobacco columns would be dominated by diffusion and interception because a number of small cut-fillers are included in tobacco columns due to fragmentation in the manufacturing process and the low linear velocity through tobacco columns under smoking conditions. In addition, it is well known in the filter research of tobacco smoke that diffusion and interception are the dominant collection mechanism (Du et al. Citation2015). Hence, only the research that focused on diffusion and interception was selected in the comparison. Mann and Goren Citation(1984) investigated the effect of diffusion and gravity on the filtration efficiency by use of DOP (dioctyl phthalate) particles (0.15–4.7 μm) in the range of superficial velocity from 2.5 to 80 mm/s with glass beads (2.13, 3.69, and 15.6 mm) and crushed shale rocks (2.2 and 16.6 mm). The effect of interception was not focused on in the research; however, the results make good use of indicating the only effect of diffusion. Otani et al. Citation(1989) conducted the experiments of mechanical filtration as a function of Re number with lead spheres (1.2 and 2 mm) and alumina particles (0.5 and 1 mm). The measurements were carried out by using NaCl (0.02–0.3 μm) and PSL (0.5–2.02 μm) particles under the condition of superficial velocity from 0.4 to 120 cm/s. They reported that the constructed equations can be applied in a wide range of experimental conditions. Kimura et al. (Citation1983a) and Mori et al. Citation(1985) proposed the prediction equations in diffusion and interception dominated regimes and they implemented the same experiments. The differences in the research were the models of collection and air flow. PSL and stearic acid particles and 13 types of granules (88–4340 μm) were used in the measurements. It has been reported in the research that the prediction efficiency agreed with the experimental results within the range of superficial velocity 0.2–20 cm/s, aerosol particle diameter 0.31–2.0 μm, and granule diameter 88–4300 μm. Lee and Gieseke Citation(1979) reported the prediction equations for diffusion and interception collection by using the Kuwabara flow model. They utilized the experimental data of previous research and found that the prediction accuracy was precise. shows the results of the comparison between experimental results and prediction results calculated by previous research with commercial brand A. In this calculation, we have set 160 μm as the granular particle diameter of tobacco columns at which diameter the predicted filtration efficiency calculated by Otani et al. Citation(1989), Mori et al. Citation(1985), and Lee and Gieseke Citation(1979) agreed closely with the experimental results.
Figure 5. The actual filtration efficiency and the predicted filtration efficiency of each reference through a tobacco column. (a) Flow velocity vs. filtration efficiency (PSL: 200 nm) and (b) PSL particle size vs. filtration efficiency (flow volume: 1.05 L/min).
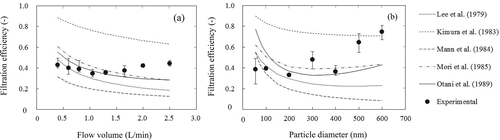
As can be seen from , there was a gap between the predicted filtration efficiency and the experimental filtration efficiency. It should be noted that while diffusion was lower, interception and inertia were higher compared to the spherical packed models. The results suggest that the flow inside tobacco columns is heterogenous, being partially faster than expected since some flow paths are filled up with the small cut-fillers and cut-filler overlaps. Kimura et al. (1983) examined the influence of granular shape on filtration efficiency in the diffusion and interception dominated regions. It was reported that filtration efficiency was increased with particle surface area in comparison to spherical shape. Since filtration efficiency in diffusion region was reduced in our results, we can deduce that the flow speed effect on filtration efficiency must be significant in heterogenous packed beds. Tobacco columns are expected to have heterogeneity in the circumferential direction and long axis direction, which leads to the specific flow inside the columns. Hence, filtration efficiency through tobacco columns disaccords with spherical packed beds, revealing that each collection mechanism of mechanical filtration should be modified from the experimental results to fit filtration efficiency. It means that it is difficult to utilize the theoretical equations to heterogenous packed beds because the heterogeneity changes the flow speed inside the columns.
Filtration equations for tobacco columns
It can be seen from that the filtration efficiencies calculated by the equations of Otani et al. Citation(1989) and Mori et al. Citation(1985) were close to the experimental results. Otani et al. Citation(1989) classified the collection mechanism of mechanical filtration into diffusion, interception, inertia, and gravity, and the sum of each mechanism shows overall filtration efficiency. From the perspective of convenience, the filtration equations reported by Otani et al. Citation(1989) were selected and modified so as to fit the experimental data. A fitting approach has been carried out to modify each collection mechanism of mechanical filtration equation through changing the empirical constants. PSL particle diameter (55–602 nm) and air volume (0.4–2.5 L/min) have been changed in this experiment. The dominant collection mechanisms of mechanical filtration were diffusion, interception, and inertia independently or simultaneously according to the theoretical model of Otani et al. Citation(1989). These can be separated into four categories as follows: (a) diffusion, (b) diffusion and interception, (c) interception, (d) interception and inertia.
Extracting the experimental conditions that are allocated with (a)–(d) as shown in , and modifying each empirical constant of the filtration equations to fit the experiment date, bridges the gap between the predicted filtration efficiency and the experimental filtration efficiency. The interaction of each collection mechanism was not considered because the modifications were done independently in this method. Sorting the experimental conditions into four categories was carried out based on whether each filtration efficiency exceeded 0.1. The modification was implemented on the basis of the determination coefficient and slope of linear regression analysis. The modified filtration equations in this method for tobacco columns are shown as follows:
Table 2. The dominant collection mechanisms under the experimental conditions.
[3]
[4]
[5]
[6] The single collection efficiency of diffusion ηD was proposed in Equations Equation(2) and (3). The single collection efficiency is a function of Sc and Re and their power of number depends on the Re number. The empirical constants of f1 (Re) and f2 (Re) (Re < 30) have been changed from −2/3 to −7/10 and from −2/3 to −0.80, respectively. The predicted equation of the single collection efficiency of interception ηR is shown in Equation Equation(4)
[4] . It has been well known that the effect of interception on filtration efficiency is proportional to R2 for low flow condition. In this study, the power of number of (Re1/3 + 1) has been changed from 3 to 2.6. The single collection efficiency of inertia ηI was written in Equation Equation(5)
[5] . The single collection efficiency was directly influenced by Stkeff. The empirical constant of ηI has been modified from 1.4 to 0.5. In Equation Equation(6)
[6] , nothing has been changed because of the effect of gravity on filtration.
shows a comparison between the experimental filtration efficiency and the predicted filtration efficiency calculated by the modified filtration equations. As indicated in the figures, the predicted filtration efficiency of the modified equations is close to the experimental results. There was a small gap at 55 nm because it is inferred from the results that the influence of the flow speed inside tobacco columns has a great impact on the diffusion dominated region. Provided that the linear velocity through tobacco columns was twice as fast as expected, the predicted filtration efficiency at 55 nm can be in good agreement with the experimental filtration efficiency. It also suggests there is some possibility that a partially faster flow inside tobacco columns could be twice as fast as expected. In this modification, the intentional reduction to fill the gap at a particle diameter of 55 nm has not been implemented because the influence on filtration efficiency at other particle diameters was significant. In addition, since the particle diameter of 55 nm has a small impact on particle weight, we have considered the influence of this gap on the tobacco smoke yield as negligible.
Introduction of cut-filler shape and size distribution effects into filtration equations
The granular particle diameter of packed beds is important for the calculation of filtration efficiency because it has a significant impact on filtration efficiency. In this study, the diameter (160 μm) that is the closest to the experimental results has been selected as being representative of tobacco columns. We have confirmed in advance that the granular particle diameter (sphere equivalent diameter) that was determined through measurements of the pressure drop (653 μm, calculated by equations of Ergun) and the projection cross-sectional area determined through Camsizer (Retsch, Haan, Germany, AS200) (450 μm: median diameter on a number basis and 267 μm: geometric average diameter on a number basis) were different from 160 μm with commercial brand A. The difficulty lies in estimating the granular particle diameter of heterogenous packed beds because the effects of the granular shape, size distribution, and orientation on mechanical filtration are different from the sphere equivalent diameter. However, previous research on filtration equations is helpful to obtain the approximate sphere equivalent diameter of mechanical filtration for heterogenous packed beds. There are numerous commercial cigarettes on the market and the physical properties of their cut-fillers differ from each other, which is to say that their sphere equivalent diameters are different. Therefore, it is industrially beneficial that inputting a new factor into filtration equations with the same sphere equivalent diameter enables the prediction of filtration efficiency through tobacco columns among several commercial products. We believe that the new factor is also effective as an indicator of mechanical filtration through other heterogenous packed beds.
The factors that have an influence on filtration efficiency include cut-filler shape, size distribution, and orientation. Several samples and experiments that are intentionally controlled to change the factors are needed in order to understand the effects of the factors on filtration efficiency. However, it is difficult to control the factors at the current technical level. For this reason, six commercial products that have different physical properties of cut-fillers have been exploited and investigated for the relationship between filtration efficiency and the cut-filler shape and the size distribution so as to find effective factors.
The shape-size factor as an indicator of cut-filler shape and size distribution was determined through pressure drop measurements by changing the air flow volume. Kaneki and Tokida Citation(1991) utilized a method of shape factor for wood fiber reported by Takahashi et al. Citation(1972). We have implemented the same analysis so as to investigate the simultaneous effect of the cut-filler shape and size distribution on filtration efficiency. The equation was as follows:[7]
Since (1 − ϵ), DG, and L are constant. K is defined as shape-size factor in this study, Equation Equation(7)[7] can be transformed as below:
[8]
[9]
[10]
Equation Equation(8)[8] shows that C1 and C2 are derived from the relationship between linear flow v and ΔP/v, and the shape-size factor can be calculated by C1, C2 and a solution to simultaneous Equations Equation(9) and (10). These equations are appropriate because tobacco columns have a linear relationship between linear flow v and ΔP/v. shows the measurement results of the granular particle diameter, and the shape-size factor calculated from Equations Equation(7)–(10). The shape size factor is relevant to the surface area of the granules because the factor is obtained from the pressure drop measurement. Accordingly, if the cigarette has a high fraction of small cut-fillers, the factor has a larger value due to an increase in pressure drop. In addition, if the cut-fillers have complicated shapes, the factor increases with the pressure drop.
Table 3. The shape-size factor and the distribution factor determined through each of the measurements.
The distribution factor as an indicator of cut-filler size distribution was determined through the sieve measurements. The inverse standard normal cumulative distribution function (NORMSINV) was calculated from the weight cumulative ratio on each sieve size (minus sieve). The slope of approximation straight line between log-minus sieve size and NORMSINV was defined as the distribution factor in Equation Equation(11)[11] :
[11] where D is the distribution factor, Di is sieve size, Dm is average of sieve size, fi is normal cumulative distribution ratio, and fm is average of normal cumulative distribution ratio. The larger value of the distribution factor means the distribution could be sharp and reflect a low fraction of small cut-fillers. Generally, the factor has been used as an indicator of sharpness of size distribution. shows the results of sieve measurements and the relationship between log-minus sieve size and NORMSINV. As can been seen from , the relationship can be approximated by a linear equation.
Figure 7. Experimental results of sieve measurements with the commercial brands. (a) Weight frequency of log-normal size distribution and (b) inverse standard normal cumulative distribution (NORMSINV) vs. log minus sieve size.
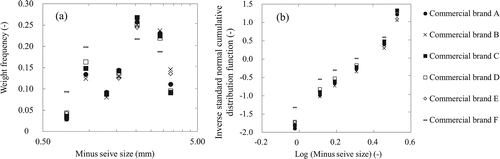
and show the relationship between the shape-size factor, the distribution factor, and the average granule size and filtration efficiency of PSL 200 nm at 1.05 L/min and 1.65 L/min, which are conditions equal to the flow speed of ISO: 35 mL/2 s and 55 mL/2 s smoking condition, respectively. Filtration efficiency was corrected by the filtration Equation Equation(1)[1] with the same packing ratio (α = 0.4) because each commercial product has a different packing ratio. The results in the figures show that the shape-size factor (r = 0.894, p < 0.05) has a high correlation and the distribution factor (r = 0.683, p < 0.15) has a moderate correlation with filtration efficiency. The shape-size factor has a higher correlation with filtration efficiency. It can be seen that the higher the shape-size factor, the higher the filtration efficiency, because the complication of flow lines and the fraction of small cut-fillers increased filtration efficiency. In contrast, it seems that the low distribution factor causes the high filtration efficiency, because reducing the granular particle diameter increases the interception effect. The average granule size has a low correlation with filtration efficiency, revealing the difficulties of representing the cut-filler shape and the size distribution from the perspective of the average granule size. These results indicate that cut-filler size distribution has an impact on filtration, and what is more, cut-filler shape also should be taken into account in filtration. It is reasonable to conclude that the indicator of expressing both the effect of the cut-filler shape and the size distribution of tobacco columns is the shape-size factor since the factor was derived against the surface area. In other words, air permeability methods are useful to evaluate the granular shape and size distribution simultaneously. The prediction accuracy of filtration efficiency among different commercial products is expected to improve by adding the shape-size factor into the filtration equation. The applied equation using the shape-size factor is noted below:
Figure 8. Relationships between the filtration efficiency under the condition of PSL: 200 nm and flow volume: 1.05 L/min and (a) the shape-size factor, (b) the distribution factor, and (c) the average granule size.
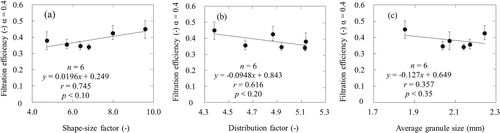
Figure 9. Relationships between the filtration efficiency under the condition of PSL: 200 nm and flow volume: 1.65 L/min and (a) the shape-size factor, (b) the distribution factor, and (c) the average granule size.
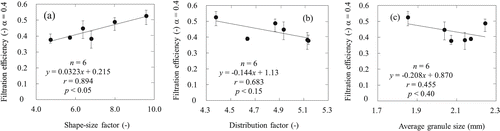
Prediction accuracy of filtration efficiency during burning
The applicability test to burning cigarettes was implemented on the basis of the smoking experiments by using the filtration Equations Equation(2)–(6) and (12). Since the particle concentration during burning is different by one or two orders of magnitude from that of the model experiments, the purpose of this experiment was the validation of prediction accuracy and the effectiveness of the shape-size factor on burning cigarettes.
shows the results of the comparison between the actual filtration efficiency during burning under the different smoking conditions and the predicted filtration efficiency (smoke particle diameter: 200 nm reported by Katayama et al. Citation(2005)) with commercial brand A. The density of tobacco smoke particles has been set 1.18 kg/m3 as reported from Johnson et al. Citation(2014). The differences in filtration efficiency in under the same smoking condition show the difference of puffing positions (butt length) except for the first puff due to the influence of the ignition. The predicted filtration efficiency was in good agreement with the actual filtration efficiency (R2 = 0.915, p < 0.01) under all smoking conditions (14 mL/2 s, 35 mL/2 s, and 55 mL/2 s). These results show that the filtration equations are applicable to burning cigarettes, and the model experiments using PSL particles must be useful in understanding mechanical filtration through tobacco columns even though the cigarettes are not burned. That is to say, filtration experiments that use solid particles are effective, whereas the experiments that use tar and nicotine have the possibility of making an erroneous interpretation regarding mechanical filtration.
Figure 10. Comparison results of the filtration efficiency during burning with commercial product A under three types of smoking conditions.
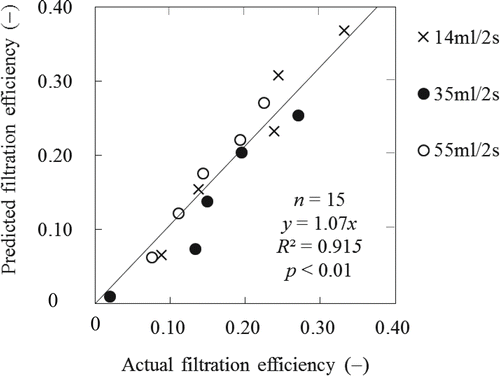
The applicability test of the shape-size factor to the burning commercial products was carried out. shows the comparison results between the actual filtration efficiency during burning under the ISO smoking condition and the predicted filtration efficiency with: (a) and without: (b) the shape-size factor among commercial products. In this calculation, we have set 160 μm as the granular particle diameter for all products so as to confirm the effect of shape-size factor. It can be seen from that the commercial brands that have a small or large shape-size factor had a gap between the actual filtration efficiency and predicted filtration efficiency. In contrast, the filtration equations that took the shape-size factor into account have improved the correlation (R2 = 0.880, p < 0.01) compared to the result without the shape-size factor (R2 = 0.749, p < 0.01), suggesting the shape-size factor is useful in predicting filtration efficiency even during burning.
Conclusion
Tobacco columns have different filtration characteristics compared to spherical packed beds with uniformed granular sizes. The diffusion effect was lower and the inertia and interception effect was higher than the spherical packed beds, implying a partially faster flow could occur in tobacco columns. That means heterogenous packed beds show the different filtration behavior compared to homogenous packed beds. It is necessary to modify the filtration equations so as to fit the filtration efficiency for heterogenous packed beds.
The influence of cut-filler size distribution was significant on filtration efficiency; however, the influence of cut-filler shape should also be taken into consideration. On the basis of the equations reported by Otani et al. Citation(1989), modified filtration equations that take the shape-size factor into account have been obtained and the validation of prediction accuracy during burning was performed. It has been confirmed that the predicted filtration efficiency was in good agreement with the actual filtration efficiency. Accordingly, we would like to emphasize that introducing the simple and effective shape-size factor that enables the showing of the granular shape and size distribution into filtration equations is useful to heterogenous packed beds.
Nomenclature
Cm | = | slip correction efficiency (−) |
D | = | distribution factor (−) |
DG | = | granular particle diameter (μm) |
Di | = | sieve size i (mm) |
Dm | = | average of sieve size (mm) |
DP | = | aerosol particle diameter (μm) |
Ε | = | filtration efficiency (−) |
fi | = | normal cumulative distribution ratio as i sieve size (−) |
fm | = | average of normal cumulative distribution ratio (−) |
G | = | gravity parameter (−) |
K | = | shape-size factor (−) |
L | = | length of packed bed (mm) |
R | = | interception parameter = DP /DG (−) |
Re | = | Reynolds number (−) |
Sc | = | Schmidt number (−) |
Stk | = | Stokes number (−) |
Stkeff | = | modified Stokes number (−) |
v | = | liner velocity (m/s) |
α | = | packing ratio (−) |
ϵ | = | void ratio (−) |
η | = | single collection efficiency (−) |
ηD | = | single collection efficiency of diffusion (−) |
ηG | = | single collection efficiency of gravity (−) |
ηI | = | single collection efficiency of inertia (−) |
ηR | = | single collection efficiency of interception (−) |
μ | = | air viscosity (Pa.s) |
ρa | = | air density (10−3 g/cm3) |
ρP | = | aerosol particle density (g/cm3) |
ΔP | = | pressure drop through packed beds (Pa) |
Reference
- Byckling, E. (1976). Investigation into the Filter Efficiency of the Tobacco Rod in Cigarettes of Differing Density in Dependence on the Smoked Length. Beitr. Tabakforsch., 8(6):382–391.
- Choi, H. J., Kim, J. U., Kim, S. H., and Lee, M. H. (2014). Preparation of Granular Ceramic Filter and Prediction of Its Efficiency. Aerosol Sci. Technol., 48:1070–1079.
- CORESTA Association. (2002). Environmental Tobacco Smoke - Estimation of its Contribution to Respirable Suspended Particles - Method based on Solanesol Determination. CORESTA Recommended Method no. 52. Available at http://www.coresta.org/Recommended_Methods/CRM_52.pdf, Accessed on August 8, 2015.
- D'Ottavio, T., and Goren, S. L. (1983). Aerosol Capture in Granular Beds in the Impaction Dominated Regime. Aerosol Sci. Technol., 2:91–108.
- Du, W., Wen, J., Peng, B., Zhang, X., Xie, F., Liu, H., and Zhong, K. (2015). An Improved Theoretical Model of Cigarette Smoke Filtration across Mono-Segment Cellulose Acetate Filters. Beitr. Tabakforsch. Int., 26(5):233–240.
- Dube, M. M., and Green, C. R. (1982). Method of Collection of Smoke for Analytical Purposes. Recent Adv. Tobacco Sci., 8:42–102.
- Futamura, Y., Takeda, K., Tokida, A., and Muramatsu, M. (1991). A Ventilation Model of Cigarettes -A One-Dimensional Mathematical Model of Tobacco Rod, in Proceedings of Papers Presented at the Joint Meeting of the Smoke and Technology Groups (CORESTA). pp. 25–32. Paris, France: CORESTA.
- George, H. F., and Poehkein, G. W. (1974). Capture of Aerosol Particles by Spherical Collectors. Electrostatic, Inertial, Interception and Viscous Effects. Environ. Sci. Technol., 8(1):46–49.
- Inagaki, M., Namiki, N., and Otani, Y. (2003). Influences of Fiber Crimp and Cross-sectional Shape on Particle Collection Performance of Cigarette Filter. Earozoru Kenkyu., 18(4):278–286 (in Japanese).
- Johnson, T. J., Olfert, J. S., Cabot, R., Treacy, C., Yurteri, C. U., Dickens, C., McAughey, J., and Symonds, J. P. R. (2014). Steady-State Measurement of the Effective Particle Density of Cigarette Smoke. J. Aerosol Sci., 75:9–16.
- Jung, Y., and Tien, C. (1992). Increase in Collector Efficiency Due to Deposition in Polydispersed Granular Filtration—an Experimental Study. Aerosol Sci. Technol., 23(5):525–537.
- Kaneki, K., and Tokida, A. (1991). Filtration on Mainstream Smoke in a Tobacco Column, in Proceedings of the 8th Symposium on Aerosol Science and Technology. pp. 92–94 (in Japanese). Kyoto, Japan: Association of Aerosol Science and Technology.
- Katayama, K., Miura, K., Kitao, S., Shimada, M., and Okuyama, K. (2005). Measurement of Property Change in Cigarette Main-Stream Smoke Using Laser Light Scattering Method. Earozoru Kenkyu. 20(4):345–351 (in Japanese).
- Keith, C. H. (1978). Physical Mechanism of Smoke Filtration. Recent Adv. Tobacco Sci., 4:25–45.
- Kimura, N., Mori, H., Tomita, T., Yagi, A., and Shirato, M. (1983a). Collection Efficiency of Granular Bed Filters by Diffusion and Direct Interception. Kagaku Kogaku Ronbun., 9(5):536–541 (in Japanese).
- Kimura, N., Mori, H., Yagi, A., Imanishi, Y., and Shirato, M. (1983b). Effect of Granular Shape on the Collection Efficiency of Granular Bed Filter by Diffusion and Direct Interception. Kagaku Kogaku Ronbun., 9(5):578–580 (in Japanese).
- Lee, K. W., and Gieseke, J. A. (1979). Collection of Aerosol Particles by Packed Beds. Environ. Sci. Technol., 13(4):466–470.
- Mann, L. A., and Goren, S. L. (1984). Aerosol Capture in Granular Beds in the Sedimentation and Diffusion Dominated Regimes. Aerosol Sci. Technol., 3(2):195–213.
- Mori, H., Kimura, N., Aragaki, T., Toyama, S., and Shirato, M. (1985). Collection Efficiency of Granular Bed Filters Due to Diffusion, Interception and Sedimentation. Kagaku Kogaku Ronbun., 11(2):134–139 (in Japanese).
- Otani, Y., Kanaoka, C., and Emi, H. (1989). Experimental Study of Aerosol Filtration by the Granular Bed Over a Wide Range of Reynolds Numbers. Aerosol Sci. Technol., 10:463–474.
- Takahashi, Y., Endo, K., and Suzuki, H. (1972). Studies on Handling Process of Wood Fibers for Dry processing of Fiberboard. V. On Pressure Drop of Air thorough Fibermat. J. Wood Sci., 18(5):231–236 (in Japanese).
- Tang, H., Richards, G., Benner, C. L., Tuominen, J. P., Lee, M. L., Lewis, E. A., Hansen, L. D., and Eatough, D. J. (1990). Solanesol: A Tracer for Environmental Tobacco Smoke Particles, Environ. Sci. Technol., 24(6):848–852.
- Veerapaneni, S., and Wiesner, M. (1993). Role of Suspension Polydispersivity in Granular Media Filtration. J. Environ. Eng., 119(1):172–190.