ABSTRACT
Cabin air filters are the main barrier for protecting automobile passengers from on road particulate matter. There are many studies about the evaluation of their performance in terms of filtration efficiency. However, the knowledge about the loading capacity of them is still lacking. Meanwhile, there has been no quantitative method to estimate the proper filter service life time. This study focuses on testing the loading capacity of different types of cabin air filters under the conditions of different relative humidity values and particle types. The results indicate that when the relative humidity increases, the activated carbon coated filters can adsorb significant amounts of water with no significant increase of the pressure drop. The normal fibrous filters show in contrast negligible water adsorbance. Compared with the filters loaded by Arizona road dust only, loading the filters by Arizona road dust and soot particles simultaneously will result in the steeper loading curves as well as the shift of most penetrating particle size to the smaller diameter. Finally, a new method to estimate the proper service life time of the cabin air filters is suggested based on the loading curves.
© 2016 American Association for Aerosol Research
EDITOR:
Introduction
In-vehicle air quality has gotten more and more attention recently with the rapidly increasing number of private automobiles, especially in developing countries. Among the potential airborne pollutants in vehicle cabins, research has focused on ultrafine particulate matter (PM) with diameters smaller than 100 nm due to their special characteristics in terms of physical, chemical, and toxicological properties. The in-vehicle environment has been proven to be a major setting for ultrafine PM exposure (Fruin et al. Citation2008; Wallace and Ott Citation2011). At the same time, many epidemiological and toxicological studies have demonstrated that the small size, high specific area, and composition of the traffic-related ultrafine PM are associated with both acute health response and chronic pulmonary and cardiovascular diseases (Salvi et al. Citation1999; Penttinen et al. Citation2001; Brugge et al. Citation2007; Valavanidis et al. Citation2008; Vattanasit et al. Citation2014).
The cabin air filter is an essential component of the ventilation system of an automobile to remove air pollutants, especially PM. Its performance depends on the setting of the ventilation mode. When it is under the Recirculation condition, the cumulative efficiency of the filtration system is much higher than that under the Outside air intake condition, because the air inside of the cabin passes through the filter multiple times due to the recirculation (Pui et al. Citation2008; Qi et al. Citation2008; Hudda et al. Citation2011). However, the advantage of the Outside air intake condition is the lower level of CO2 accumulation in the cabin. To solve this dilemma, Lee and Zhu developed a new type of filter to mitigate ultrafine particles and CO2 simultaneously (Lee and Zhu Citation2014). The filtration efficiency and pressure drop of the cabin air filter can also be influenced by air humidity due to the adsorption of water by both the filter and collected particulates. Xu Citation(2014) found that the filtration efficiency and pressure drop increased with the extending exposure time of the filter under a certain relative humidity (RH) value, and a semi-empirical equation was also developed to account for the humidity influence. To our knowledge, the majority of the studies about cabin air filters are focused on testing the filtration efficiency and studying the factors that can influence it, such as humidity and usage time. However, the loading capacity in terms of the relationship between the loaded mass and pressure drop has not been studied systematically.
Theoretically, the filtration process of the pleated filters such as cabin air filters can be characterized by three sequential stages (Joubert et al. Citation2010): (1) in-depth filtration, where particles are collected inside of the filter media; (2) surface filtration, where the filtration cake is formed on the surface of the filter; (3) reduction of the effective filtration surface area due to the build-up of the filtration cake. The particle collection mechanisms of in-depth filtration and surface filtration are the same for both pleated and flat filters (quantitative methods to calculate the pressure drop of the flat filters can be found in the supplemental information (SI)). However, different from the flat filters, the increasing thickness of the particulate cake formed on the surface of pleated filters decreases the effective filtration surface area. For the surface filtration stage, the pressure drop of the filter is normally linearly proportional to the loaded particle mass indicating a linear loading curve, however the curve grows exponentially in the final filtration stage, due to the decrease of the effective filtration surface area.
Cabin air filters are low efficiency pleated filters rather than high efficiency ones, such as high efficiency particulate air (HEPA) filters, which were commonly used in other studies (Gupta et al. Citation1993; Thomas et al. Citation1999; Joubert et al. Citation2010; Joubert et al. Citation2011), and their loading process is more complicated. The loading curves of low efficiency filters may include the whole three filtration stages. In high efficiency filters, on the other hand, the in-depth filtration stage is accomplished so fast that loading experiments cannot indicate it clearly. Meanwhile the particle size distribution of the filtration cake cannot be treated as the same as that of the upstream of the low efficiency filters, which is the normally utilized method for high efficiency ones, due to the significantly different filtration efficiencies for different particle sizes by the low efficiency filters. Even though there is no exact analytical equation to express the pressure drop for low efficiency pleated filters, Equations (S1) to (S5) in the SI can be used to describe the relationships among the related parameters qualitatively (Cheng and Tsai Citation1998; Endo et al. Citation1998; Kim et al. Citation2009; Liu et al. Citation2013). In practice, loading capacity is an important indicator for the performance of a filter, which can be used to estimate its proper service life time. Currently the vehicle manufacturers recommend changing the cabin air filters every 19,200 to 24,000 km (12,000 to 15,000 miles) during routine maintenance to protect the fan and also to guarantee good ventilation of the automobiles (Schnubel Citation2012). Guidelines for the filter service life time under different conditions are lacking, because the loading characteristics may depend on the particle properties and ambient conditions. In order to fill this knowledge gap, the loading process of various particles at different humidity values for commercial cabin air filters was simulated in a wind tunnel and the trends of loading capacity as well as the evolution of filtration efficiency with the loading process were studied in detail.
Materials and methods
Characterization of cabin air filters
Two types of commercial aftermarket cabin air filters were tested and compared with each other, which were normal fibrous filters (NF) (JC PREMIUM Inc., Czosnow, Poland, Module “B4 × 014PR”) and activated carbon coated filters (CF) (MAPCO Inc., Borkheide, Germany, Module “67713”). The average porosity of NF was obtained by utilizing a pycnometer (MICROMERITICS, USA, AccuPyc II1340), which measured the true volume occupied by the fibers of the filter (Buha et al. Citation2014). The two types of filters shared the same dimensions in length, height, and width, however, the filter medium in CF was much thicker than that in NF due to the structural difference. Images of Scanning Electron Microscopy (SEM) showed that the NF had a double-layer structure, in which the surface layer facing the air flow had a loose fibrous structure, and the second fibrous layer behind it was much denser. Different from NF, the CF had a triple-layer structure, in which a layer of particulate activated carbon was located between the two fibrous layers. The properties of the NF and CF were shown in and the SEM images were shown in the SI.
Table 1. Properties of the NF and CF.
Composition of the loading particles
Previous studies found that the on-road PM often display a bimodal size distribution, with the majority of particle diameters smaller than 300 nm in terms of number or surface area distributions (Kittelson et al. Citation2004; Yao et al. Citation2006). Arizona road dust (ARD) and soot particles were utilized as the loading particles in the experiments to mimic the real on-road particulates. The ARD (ISO 12103-1, A1) injected into the wind tunnel by a powder disperser (PALAS Model RBG 1000) was used to generate the suspended road dust, which had been proven to be an appropriate proxy by previous studies (Kievit et al. Citation1996; Xu et al. Citation2011). Besides that, the soot particles produced by a miniCAST (model 5201, type C, Jing AG, Switzerland, working at operation point 5) were used to simulate the automobile exhaust. The particle generation rates of both the powder disperser and miniCAST were set to achieve high particle concentrations so that enough particles downstream of the filters could be measured for reliable determination of the filtration efficiencies within a reasonable time frame. A Scanning Mobility Particle Sizer (SMPS, Model 3936, TSI Inc.) was utilized to determine particle size distributions of soot particles over their whole size range. In order to cover the wide size distribution of the ARD, the Aerodynamic Particle Sizer (APS, Model 3321, TSI Inc.) with the measuring range from 700 nm to 20 μm was utilized in addition to the SMPS. When the filters were loaded by only ARD, the measuring size range of SMPS was up to 720 nm. However, when the soot particles and ARD were utilized to load the filters simultaneously, the measuring size range of SMPS was up to 245.8 nm to focus on the size distribution of soot particles due to their much higher number concentration of ultrafine particles compared with the ARD.
Wind tunnel and experimental design
The experiments were conducted in a wind tunnel compatible with DIN EN 779 standard and capable of testing full-sized ventilation filters. By controlling the fan, heater, and humidifier, we could maintain the air flow rate between 800 and 5400 m3/h, temperature between room temperature and 40°C, and RH between 20% and 95%. The testing section of the wind tunnel was 11.7 m in length and the cross-section was 610 mm by 610 mm. The upstream injection nozzle for dispersing the test particles into the wind tunnel, the upstream sampling probe, the tested cabin air filters, and the downstream sampling probe for sampling the particles penetrating through the filters were located sequentially along the testing section. Due to the recirculation configuration of the wind tunnel, another high efficiency filter was installed downstream of the testing section to prevent the penetrating particles flowing to the fan. The air velocity and aerosol uniformities at one cross-section in the testing section of the wind tunnel were verified by measuring air velocity and aerosol concentration in different regions of the cross-section (details can be found in the SI). These tests demonstrated that the performance of the wind tunnel was in accordance with the DIN EN 779 standard.
A custom made filter holder was used to hold the tested cabin air filters inside the wind tunnel and to stabilize them against the incoming air flow. Two identical cabin air filters were placed on the filter holder in parallel, and the edges of the filter holder as well as the space between the two cabin air filters were all tightly sealed by rubber gaskets. The schematic of wind tunnel and how the filter holder was positioned in it were shown in . Once the filter holder was placed in the wind tunnel, the air flow in the vicinity of the holder was disturbed and not uniform over the cross-section of the tunnel.
In the experiments, the loading particles were generated and injected into the wind tunnel, then transported by the air flow to challenge the filters. The pressure drop across the tested filters was recorded continuously by a manometer, meanwhile the mass of particles collected by the tested filters, and the particle number concentrations upstream and downstream of the filters were measured a number of times during the entire loading process. Because two identical filters were tested simultaneously, the obtained pressure drop and calculated filtration efficiency were both average values of the two filters. The filters were taken out of the wind tunnel for the mass measurement, and generally a new measurement was taken when the loaded mass of the two filters was increased more than 5 g. The calculated value of the loaded mass per unit surface area was an average value that was the ratio between the total loaded mass of the two filters and the total surface area of them. The loading experiment was conducted until the pressure drop of the tested filters increased to about four to five times of that of the clean filters, which was commonly used as the end point of loading experiments indicative of the service life time of the filters (Thomas et al. Citation1999; Thomas et al. Citation2001; Xu Citation2014). Loading curves were obtained from the testing data of filters that experienced the complete loading process. In order to confirm the representativeness of the results, we performed several separated loading tests with shorter durations, for which the pressure drop and loaded mass were not periodically measured, instead, the final pressure drop and loaded mass were recorded. The temperature inside the wind tunnel was maintained at the room temperature around 25°C and the air flow rate was set to a constant value of 860 m3/ h (430 m3/ h for one filter on average). The average surface filtration velocity of NF and CF was 0.25 m/s and 0.3 m/s, respectively, due to their different effective filtration surface areas. The tested filtration velocity values were within the normal range measured in the automobiles, which is between 0.1 m/s and 0.5 m/s (Xu et al. Citation2011). According to the classification of automobiles in the US (40 CFR 600.315-82-ClASSES OF COMPARABLE AUTOMOBILES), for a typical midsize car with the interior volume about 3.2 m3, the calculated air exchange rate within the car was 134 h−1 based on the flow rate used in the experiments, which is comparable with the measured values for the full fan settings under outside air intake condition (Hudda et al. Citation2011).
Totally six experiments were designed to study the influences of RH, particulate properties, and filter type on the performance of cabin air filters as listed in . To be specific, three pairs of comparisons (experiment I versus IV, II versus V, III versus VI) were made to study the performance of filters under two different RH values (30% and 80%). Comparisons of experiment I versus II and I versus III were made to study the influence of particulate properties (ARD versus ARD and soot particles) and filter type (NF versus CF), respectively. The loading experiments with only soot particles were not conducted, due to the low mass of the soot particles and associated uncertainties in the gravimetric measurement. The performances of cabin air filters were compared based on several indicators, such as the shape of the loading curves, average filtration efficiency, and the most penetrating particle size (MPPS).
Table 2. Summary of the six sets of experimental conditions.
Results and discussion
The size distributions of the soot particles and ARD upstream of the filters were monitored over the whole loading processes shown in . When particles were released into the wind tunnel, uncertainties might be introduced due to the occasionally unstable air flow condition and the working states of the miniCAST and powder disperser. As shown in , although the number concentration for specific sizes showed some variances during the experiments, the mode and shape of the size distributions were almost constant, so the analysis of the loading capacity and filtration efficiency of the filters would not be influenced significantly. For the soot particles, the mode of the number-based size distribution () was around 90 nm, which was comparable with the measurement of the exhaust from a normal diesel engine excluding the nucleation mode around 10 nm (Kittelson et al. Citation2006). For the ARD, the size distribution was bimodal based on SMPS and APS measurements covering different size ranges. The first mode was between 300 nm and 400 nm that was comparable with Xu et al. Citation(2011), and the second mode was around 1 μm. The number concentration of the soot particles was much higher than that of the ARD when the particle size was smaller than 300 nm, especially for particles smaller than 100 nm. For the whole size ranges of the two types of particles, the ratio of the total number concentration of soot particles to that of the ARD was about 7.7 × 105:9.13 × 103, which was consistent with the practical measurements that indicated the majority of the particles on roads were ultrafine particles with diameters smaller than 100 nm (Hitchins et al. Citation2000; Molnár et al. Citation2002; Zhu et al. Citation2002). When both ARD and soot particles were loaded on the filters, ARD contributed the majority of the loaded mass, due to its much larger particle size as well as the higher density than that of soot particles (the effective density of ARD was about 2.65 g/cm3; Endo et al. Citation1998, and the effective density of soot particles produced by miniCAST was smaller than 1.5 g/cm3 and varied with different operational conditions; Moore et al. Citation2014).
Figure 2. Size distributions of ARD and soot particles: (a) ARD measured by SMPS; (b) ARD measured by APS; and (c) soot particles measured by SMPS.
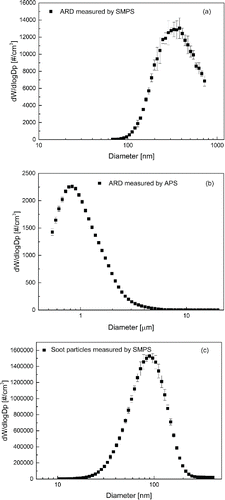
Influence of relative humidity
The loading curves of the tested cabin air filters were shown in , where the discrepancy between the data of the separated loading tests and the loading curves made from the data of the complete loading process could be explained when the experimental errors (summarized in ) were taken into account. When air flow passed through the filter, both the filter medium and collected particles were able to adsorb water in the air. The adsorbed water influenced the loading curve in several ways. On the one hand, it contributed to the total loaded mass on the filter (the abscissa of the loading curve). If the mass of the loaded particles and the pressure drop were kept constant, more adsorbed water indicated higher total loaded mass resulting in the flatter loading curve. In the present study, this effect was referred to as the “mass effect.” Meanwhile the size of the particles was increased due to the hygroscopic growth, and one particle could agglomerate with its surrounding ones and form clusters due to the capillary force induced by water between them, which would also lead to the flatter loading curve (Joubert et al. Citation2010). On the other hand, due to the hygroscopic growth of the particles, the pathway for the air flow could also become narrower, which resulted in a steeper loading curve (Xu Citation2014). For the conditions of NF loaded by only ARD shown in , the mentioned effects compensated each other, or none of them was significant indicating that neither ARD nor the filter medium of NF could adsorb water, so the RH did not influence the overall loading curves. Previous studies had indicated that neither ARD nor nascent soot particles produced by miniCAST had hygroscopic growth (Vlasenko et al. Citation2005; Koehler et al. Citation2009; Henning et al. Citation2012). For the non-hydroscopic particles such as the ARD used in this study, it had also been found that the humidity had no significant influence on the pressure drop of the filtration cake (Joubert et al. Citation2011), which was consistent with the results of this study shown in .
Figure 3. Influence of the RH on the loading curves of cabin air filters: (a) NF loaded by ARD; (b) CF loaded by ARD; (c) NF loaded by ARD and soot particles. Inserted images were the SEM images of clean NF and CF, and the loaded ones by ARD or ARD with soot particles. More SEM images with higher magnification were shown in the SI.
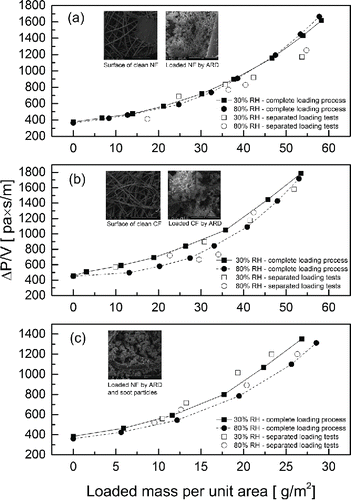
Table 3. Summary of the sources of experimental errors.
For the conditions of CF loaded by ARD and NF loaded by ARD and soot particles, as shown in , the loading curves were flatter at 80% RH. However, the differences among loading curves were not significant to conclude the influences of RH when experimental errors were taken into account. The three main possible errors in the experiments were summarized in . The main sources of the errors were the detachment of already collected particles on the filters when the filters were installed into and removed from the wind tunnel as well as the transportation process of them from the wind tunnel to the balance. Based on our experience, the overall mass of the lost particles was about 1 g to 2 g during the whole loading process, which might contribute up to around 5% of the total loaded mass depending on the types of filters as well as the loaded particles. The error due to the evaporation of adsorbed water on the filters were determined experimentally by weighting the filters continually in ambient condition for 30 min after they were exposed under 80% RH for 1 h in the wind tunnel. The instability of air flow and particle generation was also a main source of error, and the influence of it on the total loaded mass of the filter was estimated based on the monitoring data of the wind tunnel (flow rate, humidity, temperature, etc.) and experimental experience.
showed the water adsorbing capacities of NF and CF under different conditions to further investigate the humidity effect. The water adsorbing capacities were tested by putting the already loaded filters into the wind tunnel again, and the airflow without particles was passed through them with the same flow rate as in the loading experiments. First, the RH was set to 30%, after about 60 min when the pressure drop reached steady state, the humidity was increased to 80% RH. Meanwhile the pressure drop and the filter mass were recorded during the whole process. The results shown in indicated that for the conditions of NF loaded by ARD and NF loaded by ARD and soot particles simultaneously, both the pressure drop and filter mass were almost constant even though the RH increased from 30% to 80%. This was consistent with the study in which a pleated HEPA filter was loaded with the non-hygroscopic aluminum oxide particles, and the loading curves under different humidity were considered to superimpose for the surface filtration stage when RH < 95% (Kievit et al. Citation1996). However, for the CF loaded by ARD, the CF adsorbed more than 10 g of water within 20 min after the RH increased to 80%, which was almost 20% of the mass of the originally loaded particles. This finding was consistent with the previous studies that confirmed the high moisture adsorption capacity of activated carbon (Abiko Citation2011). However, different from previous studies, where the pressure drop either decreased or increased when humidity was changed (Joubert et al. Citation2011; Xu Citation2014), in this study, the pressure drop of the CF stayed almost constant although its mass increased significantly. It indicated the “mass effect” of the adsorbed water was the dominant factor resulting in the flatter loading curves of CF at the higher humidity as shown in , rather than the other effects that changed the geometry of the filtration cake. Meanwhile, it could also be inferred that mainly due to the different hygroscopic properties of both the filter medium and the loaded particles, the influences of the humidity on the loading curves of the filters varied a lot in previous studies.
Comparison of loading curves
The loading curves of tested cabin air filters at 30% RH were shown in . showed the influence of the type of particles on the loading curves. The addition of the soot particles resulted in a much steeper curve compared with loading the filter by only ARD. This was caused by the smaller size of soot particles compared to ARD and the different surface properties of the two particle types. On the one hand, the average size of loaded particles on the filter decreased significantly when soot particles were added. On the other hand, the small soot particles could fill the gaps formed by already loaded ARD and attached on the surface of them, forming more compact filtration cakes with lower porosity (the SEM images of the two loading conditions were provided in the SI). As shown in Equation (S5), the two factors resulted in lower values of (the geometric mean diameter of the particles) and ϵ (the porosity of the filtration cake), which contributed to a higher specific dust cake resistance coefficient and led to a higher pressure drop.
Figure 5. Loading curves under different conditions: (a) NF loaded by ARD and ARD with soot particles, respectively, and (b) NF and CF, taking into account the initial pressure drop and the face velocity.
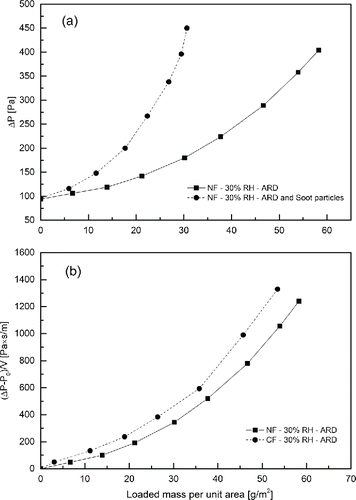
compared the performances of NF and CF. The ordinate of the figure was formulated to compensate the influences of different average face velocities (V) and initial pressure drops (ΔP0) on the performance of the filters. Experimental results showed that the increasing rates of the pressure drop were similar at the early filtration stage. With continuous loading, the pressure drop of CF increased faster than that of NF gradually, which indicated that other factors besides the average face velocity could also influence the buildup of the filtration cake. Based on the comparisons of filtration efficiency to be discussed later, the CF showed higher filtration efficiency than that of NF, especially for small particles. The higher filtration efficiency generally indicated a faster clogging process and a higher pressure drop. In practice, higher pressure drop means more energy consumption of the fan and influences the service life time of the filters, which is discussed in detail in the section of practical implications.
Comparison of the filtration efficiency
showed the evolution of the average filtration efficiency during the loading process at 30% RH. When the comparison was made between the same type of filters, the abscissa was the pressure drop as shown in . For the comparison between different types of filters, the abscissa was changed to the loaded mass per unit area as shown in , due to the large difference of the pressure drop between them. Because the diameters measured by SMPS and APS were mobility diameter () and aerodynamic diameter (
) of the particles respectively, the particle diameters shown in were all transformed to
to keep the consistency of the comparisons based on the equation (Buha et al. Citation2014):
Figure 6. Evolution of the average filtration efficiencies in different particle size ranges during the loading process under different experimental conditions: (a) NF loaded by ARD (as a function of pressure drop); (b) NF loaded by ARD and soot particles (as a function of pressure drop); (c) NF loaded by ARD (as a function of loaded mass); and (d) CF loaded by ARD (as a function of loaded mass).
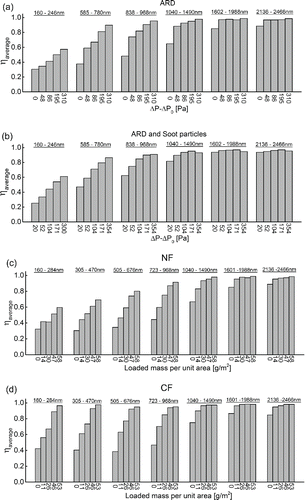
Based on the above transformation, the rang of measured by APS from 777 nm to 3278 nm was transformed to the rang of
from 585 to 2466 nm, then it was combined with the range of
measured by SMPS, which results in the final size range of ARD from 159.6 nm to 2466 nm in terms of
.
compared the filtration efficiencies of NFs loaded by only ARD and ARD with soot particles. As mentioned before, the size ranges of the SMPS measurements were different under the two conditions due to the much smaller size of soot particles than that of ARD. The size ranges used for the comparison were chosen based on the consideration that the particle number concentrations within them were high enough to calculate the filtration efficiencies reliably, thus the lowest one was from 160 nm to 246 nm that was covered by the size distributions of both ARD and soot particles and the number concentrations within it were high enough, especially for ARD. In order to compare the results, the data points with similar pressure drops under the two loading conditions were chosen from all data obtained in the loading experiments. It can be seen that the addition of soot particles had no significant influence on the filtration efficiency for particles with diameters larger than 585 nm. When the diameters of particles were larger than 1602 nm, the filtration efficiency was always higher than 90% and almost constant over the whole loading process. For the particles with diameters between 1040 nm and 1490 nm, their filtration efficiencies increased quickly from the beginning of the loading process and finally kept almost constant at the highest values. The experimental results indicated that the original cabin air filter was effective to collect large particles, meanwhile it was possible that the filtration cake collected them mainly by the sieving effect, so the increasing thickness of the filtration cake did not influence the performance of the filter. However, for small particles collected mainly by diffusion, the increasing thickness of filtration cake indicated longer travelling path through the filtration medium and resulted in the increasing filtration efficiencies. It can be corroborated by the case of particles with diameters in the size range from 160 nm to 246 nm under both loading conditions, for which the filtration efficiency kept increasing during the whole filtration process. For the particles within the size range between 585 nm and 968 nm, which was between the former mentioned small and large size ranges, the filtration efficiencies under both loading conditions increased gradually, and approached the maximum values at the end of the loading process.
compared the filtration efficiencies between CF and NF. The chosen data for comparison were with the similar loaded mass per unit area. They indicated that CF had higher filtration efficiency than NF for particles within the chosen size ranges starting from 160 nm, when they were loaded by ARD. However, as already discussed, CF also showed faster increase of the pressure drop than NF during the loading process. When CF is utilized in practice, achieving the higher filtration efficiency is at the expense of the higher increase in pressure drop.
For the tested CF and NF, the filtration efficiencies against small particles increased gradually during the loading process. However, neither filter was very effective to collect them. Especially for NF, the filtration efficiency for particles with diameters between 160 nm and 284 nm was still lower than 0.6 at the end of the loading process. It confirmed that cabin air filters are not high efficiency filters, especially for small particles (Qi et al. Citation2008; Hudda et al. Citation2011).
Most penetrating particle size
illustrated the trends of the MPPS within the measuring rang of the SMPS and APS as a function of the pressure drop of NF, which were loaded by either only ARD or ARD with soot particles. For the tests with only ARD, the MPPS was almost constant between 150 nm and 200 nm during the whole loading process of the filters at both 30% RH and 80% RH. For the tests with both ARD and soot particles, the MPPS decreased gradually from around 200 nm to less than 100 nm with the increasing pressure drop under the conditions of both humidity values, which was due to several combined factors. The MPPS lies in the particle size range in which diffusion and interception are the most important capture mechanisms. Diffusion efficiency decreases with the increasing particle size whereas the interception efficiency increases. During the loading process, the thickness of the filtration cake increases, leading to longer particle residence times and tortuous air flow paths within the filter. Consequently, both diffusion and interception efficiencies increase and their competition determines the shift direction of the MPPS. For loading with only ARD, apparently diffusion and interception efficiencies increased at similar rates and the MPPS stayed relatively constant. For loading with ARD and soot particles, the cake structure became more non-uniform due to different filtration efficiencies against small and large particles (shown in ). The small soot particles could penetrate deeper, therefore the bottom part of the filtration cake gradually became less porous with smaller average pore sizes. Thus, it was possible that the interception efficiency increased more than the diffusion efficiency, leading to smaller MPPS (Lee and Liu Citation1980; Lee Citation1981). Previous studies reported MPPS of cabin air filters was around 300 nm (Qi et al. Citation2008; Xu et al. Citation2011), which was larger than the results of this study. The difference could be attributed to the different types of filters tested in the experiments as well as the different experimental conditions. Many factors could influence the MPPS of a filter, as mentioned, and more studies are needed in the future to quantity their combined effect.
Practical implications
The influences of the RH on the cabin air filters vary significantly for different types of filters based on our experimental results. For the CF, the high capacity of water adsorbance implies that the filter medium will be in wet conditions if the ambient RH is kept at a high level, such as in tropical areas. Moisture in the filter medium as well as the loaded particles that contain organic matters will favor the growth of microorganisms. It is possible that these microorganisms and their metabolites can be blown into the cabin and pose a threat to the health of passengers. It has been found that the in-use cabin air filters could accumulate high levels of microorganisms and their metabolites (Li et al. Citation2013). Compared to the NF that has a low water adsorption capacity, CF is therefore recommended to be changed more frequently.
Our study shows that the types of loaded particles can influence the performance of cabin air filters significantly, especially for the ultrafine particles that are dominant in terms of number concentration under real conditions. In practice, the flow rate of the fan of the ventilation system is controlled by a blow motor resistor (BMR), and its rated power is normally tens of Watts depending on different designs and manufacturers. The power of the motor of the fan should be kept lower than the rated power, otherwise the BMR is damaged in short time. The power of the motor can be estimated by the equation[3] where
is the power of the fan (W);
is the pressure drop caused by the cabin air filter (Pa); and
is the air flow rate (m3/s).
If the flow rate () is kept constant to maintain a stable ventilation condition, the power (
) will be linearly proportional to the pressure drop (
) and increase gradually due to the increasing
caused by continually loaded particles. The end-of-life of the filter can be set to the point when the power equals to the value of the rated power of the BMR, and the corresponding pressure drop (maximum pressure drop) can be matched with the mass of the loaded particles according to the loading curves. For the tested NF under 30% RH used in this study, assuming the rated power is 80 W, the flow rate is 430 m3/h (0.24 m3/s), then the maximum pressure drop is 333.3 pa. Based on , when only ARD is loaded on the filter, the corresponding loaded mass is about 51 g/m2, however, when both ARD and soot particles are loaded on the filter simultaneously, the corresponding loaded mass is only about 26 g/m2. For simplicity, assuming the loaded mass on the cabin air filter is linearly proportional to the usage time, the estimated result indicates that loading soot particles together with ARD decreases nearly half of the life time of the cabin air filters compared with the condition loaded by only ARD. In practice, this implies that the automobiles normally driving under the heavy traffic conditions should change the cabin air filters more frequently, not only because of the higher concentration of air pollutants, but also due to the existence of more soot particles that contribute little to the total mass of pollutants, however can decrease the life time of the filters significantly. Although nowadays the automobiles do not equip the instruments to monitor the pressure drop of cabin air filters, and the loading curves of the filters can be varied in different road conditions, the method suggested in the present study will provide a new way for the automobile manufacturers to improve the design of the ventilation system based on the quantitative measurements.
Conclusion
The experimental results of this study confirm the low filtration efficiency of cabin air filters, especially for ultrafine particles. In practice, using the recirculation mode of the ventilation system is better to improve the filtration efficiency of ultrafine particles at the expense of CO2 accumulation. Meanwhile the soot particles used in this study demonstrate the influence of small particles, which are dominant under on-road conditions, on the structure of filtration cake. And it also shows that the particle types can influence the performance of cabin air filters significantly, although it is hard to mimic actual on-road conditions in a laboratory. Many other factors may also play important roles in practice and need to be studied further, such as the change of the particle size due to hygroscopic characteristics of the particles. Finally, the performances of different types of filters vary significantly due to their different size dimensions and structures. Generally, high filtration efficiency is normally at the expense of high pressure drop, such as in the case of CF used in this study. Developing new types of filters is therefore essential for solving this dilemma.
Funding
This work was partially supported by the Center for Filtration Research including 3M Company, BASF Corporation, Boeing Company, Cummins Filtration, Inc., Donaldson Company, Inc., Entegris, Inc., Guangxi Wat Yuan Filtration System Co., Ltd., H.B. Fuller Company, Mann+Hummel GmbH, MSP Corporation, Samsung Electronics Co., Ltd., Shigematsu Works Co., Ltd., TSI Inc., W. L. Gore & Associates, Inc., Xin-xiang Shengda Filtration Technique Co., Ltd., and the affiliate member National Institute for Occupational Safety and Health (NOISH).
supplemental_information.docx
Download MS Word (1.7 MB)References
- Abiko, H. (2011). Water Vapor Adsorption and Desorption Isotherms of Activated Carbon Products used in Japanese Gas Respirators. TANSO, 248:127–132.
- Brugge, D., Durant, J. L., and Rioux, C. (2007). Near-highway Pollutants in Motor Vehicle Exhaust: A Review of Epidemiologic Evidence of Cardiac and Pulmonary Health Risks. Environ. Health, 6:23. doi:10.1186/1476-069X-6-23.
- Buha, J., Mueller, N., Nowack, B., Ulrich, A., Losert, S., and Wang, J. (2014). Physical and Chemical Characterization of Fly Ashes from Swiss Waste Incineration Plants and Determination of the Ash Fraction in the Nanometer Range. Environ. Sci. Technol., 48(9):4765–4773.
- Cheng, Y.-H., and Tsai, C.-J. (1998). Factors Influencing Pressure Drop through a Dust Cake during Filtration. Aerosol Sci. Technol., 29(4):315–328.
- DIN EN 779. (2002). Particulate Air Filters for General Ventilation–Determination of the Filtration Performance. European Committee for Standardization.
- Endo, Y., Chen, D.-R., and Pui, D. Y. (1998). Bimodal Aerosol Loading and Dust Cake Formation on air Filters. Filtr. Sep., 35(2):191–195.
- Endo, Y., Chen, D.-R., and Pui, D. Y. (1998). Effects of Particle Polydispersity and Shape Factor During Dust Cake Loading on Air Filters. Powder Technol., 98(3):241–249.
- Fotovati, S., Hosseini, S. A., Tafreshi, H. Vahedi, and Pourdeyhimi, B. (2011). Modeling Instantaneous Pressure Drop of Pleated Thin Filter Media During Dust Loading. Chem. Eng. Sci., 66(18):4036–4046.
- Fruin, S., Westerdahl, D., Sax, T., Sioutas, C., and Fine, P. (2008). Measurements and Predictors of On-road Ultrafine Particle Concentrations and Associated Pollutants in Los Angeles. Atmos. Environ., 42(2):207–219.
- Gupta, A., Novick, V. J., Biswas, P., and Monson, P. R. (1993). Effect of Humidity and Particle Hygroscopicity on the Mass Loading Capacity of High Efficiency Particulate Air (HEPA) Filters. Aerosol Sci. Technol., 19(1):94–107.
- Henning, S., Ziese, M., Kiselev, A., Saathoff, H., Möhler, O., Mentel, T. F., Buchholz, A., Spindler, C., Michaud, V., Monier, M., Sellegri, K., and Stratmann, F. (2012). Hygroscopic Growth and Droplet Activation of Soot Particles: Uncoated, Succinic or Sulfuric Acid Coated. Atmos. Chem. Phys., 12(10):4525–4537.
- Hitchins, J., Morawska, L., Wolff, R., and Gilbert, D. (2000). Concentrations of Submicrometre Particles from Vehicle Emissions Near a Major Road. Atmos. Environ., 34(1):51–59.
- Hudda, N., Kostenidou, E., Sioutas, C., Delfino, R. J., and Fruin, S. A. (2011). Vehicle and Driving Characteristics that Influence in-cabin Particle Number Concentrations. Environ. Sci. Technol., 45(20):8691–8697.
- Joubert, A., Laborde, J. C., Bouilloux, L. S., Callé-Chazelet, and Thomas, D. (2010). Influence of Humidity on Clogging of Flat and Pleated HEPA Filters. Aerosol Sci. Technol., 44(12):1065–1076.
- Joubert, A., Laborde, J. C., Bouilloux, L., Chazelet, S., and Thomas, D. (2011). Modelling the Pressure Drop Across HEPA Filters During Cake Filtration in the Presence of Humidity. Chem. Eng. J., 166(2):616–623.
- Kievit, O., Neveling, V., Valkenburg, J., and Waqué, J. (1996). Development of a Realistic Loading Aerosol for Cabin air Filter Testing. J. Aerosol Sci., 27:S633–S634.
- Kim, S. C., Wang, J., Shin, W. G., Scheckman, J. H., and Pui, D. Y. H. (2009). Structural Properties and Filter Loading Characteristics of Soot Agglomerates. Aerosol Sci. Technol., 43(10):1033–1041.
- Kittelson, D. B., Watts, W., and Johnson, J. (2004). Nanoparticle Emissions on Minnesota Highways. Atmos. Environ., 38(1):9–19.
- Kittelson, D. B., Watts, W. F., and Johnson, J. P. (2006). On-road and Laboratory Evaluation of Combustion Aerosols—Part 1: Summary of Diesel Engine Results. J. Aerosol Sci., 37(8):913–930.
- Koehler, K., Kreidenweis, S., DeMott, P., Petters, M., Prenni, A., and Carrico, C. (2009). Hygroscopicity and Cloud Droplet Activation of Mineral Dust Aerosol. Geophys. Res. Lett., 36(8). doi:10.1029/2009GL037348
- Lee, E. S., and Zhu, Y. (2014). Application of a High-efficiency Cabin Air Filter for Simultaneous Mitigation of Ultrafine Particle and Carbon Dioxide Exposures Inside Passenger Vehicles. Environ. Sci. Technol., 48(4):2328–2335.
- Lee, K. (1981). Maximum Penetration of Aerosol Particles in Granular Bed Filters. J. Aerosol Sci., 12(1):79–87.
- Lee, K., and Liu, B. (1980). On the Minimum Efficiency and the Most Penetrating Particle Size for Fibrous Filters. J. Air Pollut. Control Assoc., 30(4):377–381.
- Li, J., Li, M., Shen, F., Zou, Z., Yao, M., and Wu, C.-y. (2013). Characterization of Biological Aerosol Exposure Risks from Automobile Air Conditioning System. Environ. Sci. Technol., 47(18):10660–10666.
- Liu, J., Swanson, J. J., Kittelson, D. B., Pui, D. Y. H., and Wang, J. (2013). Microstructural and Loading Characteristics of Diesel Aggregate Cakes. Powder Technol., 241:244–251.
- Molnár, P., Janhäll, S., and Hallquist, M. (2002). Roadside Measurements of Fine and Ultrafine Particles at a Major Road North of Gothenburg. Atmos. Environ., 36(25):4115–4123.
- Moore, R. H., Ziemba, L. D., Dutcher, D., Beyersdorf, A. J., Chan, K., Crumeyrolle, S., Raymond, T. M., Thornhill, K. L., Winstead, E. L., and Anderson, B. E. (2014). Mapping the Operation of the Miniature Combustion Aerosol Standard (Mini-CAST) Soot Generator. Aerosol Sci. Technol., 48(5):467–479.
- Penttinen, P., Timonen, K., Tiittanen, P., Mirme, A., Ruuskanen, J., and Pekkanen, J. (2001). Ultrafine Particles in Urban Air and Respiratory Health Among Adult Asthmatics. Eur. Respir. J., 17(3):428–435.
- Pui, D. Y., Qi, C., Stanley, N., Oberdorster, G., and Maynard, A. (2008). Recirculating Air Filtration Significantly Reduces Exposure to Airborne Nanoparticles. Environ. Health Perspect., 116(7):863–866.
- Qi, C., Stanley, N., Pui, D. Y., and Kuehn, T. H. (2008). Laboratory and On-road Evaluations of Cabin Air Filters Using Number and Surface Area Concentration Monitors. Environ. Sci. Technol., 42(11):4128–4132.
- Rebai, M., Prat, M., Meireles, M., Schmitz, P., and Baclet, R. (2010). Clogging Modeling in Pleated Filters for Gas Filtration. Chem. Eng. Res. Design, 88(4):476–486.
- Salvi, S., Blomberg, A., Rudell, B., Kelly, F., Sandstrom, T., Holgate, S. T., and Frew, A. (1999). Acute Inflammatory Responses in the Airways and Peripheral Blood after Short-term Exposure to Diesel Exhaust in Healthy Human Volunteers. Am. J. Respir. Crit. Care Med., 159(3):702–709.
- Schnubel, M. (2012). Today's Technician: Automotive Heating & Air Conditioning Classroom Manual and Shop Manual. Cengage Learning.
- Thomas, D., Contal, P., Renaudin, V., Penicot, P., Leclerc, D., and Vendel, J. (1999). Modelling Pressure drop in HEPA Filters During Dynamic Filtration. J. Aerosol Sci., 30(2):235–246.
- Thomas, D., Penicot, P., Contal, P., Leclerc, D., and Vendel, J. (2001). Clogging of Fibrous Filters by Solid Aerosol Particles Experimental and Modelling Study. Chem. Eng. Sci., 56(11):3549–3561.
- Valavanidis, A., Fiotakis, K., and Vlachogianni, T. (2008). Airborne Particulate Matter and Human Health: Toxicological Assessment and Importance of Size and Composition of Particles for Oxidative Damage and Carcinogenic Mechanisms. J. Environ. Sci. Health, Part C, 26(4):339–362.
- Vattanasit, U., Navasumrit, P., Khadka, M. B., Kanitwithayanun, J., Promvijit, J., Autrup, H., and Ruchirawat, M. (2014). Oxidative DNA Damage and Inflammatory Responses in Cultured Human Cells and in Humans Exposed to Traffic-related Particles. Int. J. Hyg. Environ. Health, 217(1):23–33.
- Vlasenko, A., Sjögren, S., Weingartner, E., Gäggeler, H., and Ammann, M. (2005). Generation of Submicron Arizona Test Dust Aerosol: Chemical and Hygroscopic Properties. Aerosol Sci. Technol., 39(5):452–460.
- Wallace, L., and Ott, W. (2011). Personal Exposure to Ultrafine Particles. J. Expo. Sci. Environ. Epidemiol., 21(1):20–30.
- Xu, B. (2014). Investigation of Air Humidity Affecting Filtration Efficiency and Pressure Drop of Vehicle Cabin Air Filters. Aerosol Air Qual. Res., 14(3):1066–1073.
- Xu, B., Liu, S., Liu, J., and Zhu, Y. (2011). Effects of Vehicle Cabin Filter Efficiency on Ultrafine Particle Concentration Ratios Measured In-Cabin and On-Roadway. Aerosol Sci. Technol., 45(2):234–243.
- Yao, X., Lau, N., Fang, M., and Chan, C. (2006). On the Time-averaging of Ultrafine Particle Number Size Spectra in Vehicular Plumes. Atmos. Chem. Phys., 6(12):4801–4807.
- Zhu, Y., Hinds, W. C., Kim, S., and Sioutas, C. (2002). Concentration and Size Distribution of Ultrafine Particles Near a Major Highway. J. Air Waste Manag. Assoc., 52(9):1032–1042.