ABSTRACT
In the present study, we measured the penetration of silver nanowires with the mobility diameter in the range from 200 to 400 nm through two different types of polyester filters: the screen filter with the solidity of 0.505 and fibrous filter with the solidity of 0.278. The orientation angles of silver nanowires passing through the single layer and multi-layers of polyester filter were experimentally estimated on the basis of the single fiber efficiency theory. In the case of the screen filter, the orientation angle obtained by fitting the experimental data for single layer was found to be close to 40˚, indicating a random orientation of nanowires near the filter. However, the fibrous filter has the orientation angle much larger than 40˚. The orientation angle can be affected by inhomogeneity of the filter. In particular, in the case of the fibrous filter, the solidity and fiber diameter may affect the orientation angle. For multi-layers of both screen and fibrous filters, it is difficult to determine the typical orientation angle and the fibrous filter tends to have a larger orientation angle than the screen filter. In addition, we carried out numerical simulations on the penetration of silver nanowires through the five layers of screen filter and the single layer of both screen and fibrous filters. Numerical prediction was carried out by using the three-dimensional numerical model determined by solidity and thickness of fibrous filter. Numerical predictions are highly congruent with experimental results and theoretical prediction.
© 2017 American Association for Aerosol Research
EDITOR:
1. Introduction
Particles with a high aspect ratio, such as nanowires, nano-rods, and carbon nanotubes, have a wide range of industrial applications. However, since those particles may be harmful to human health, filtration of nanoparticles with a high aspect ratio has been investigated (Ji et al. Citation2012; Wang and Otani Citation2012). The filtration of various kinds of particles with a high aspect ratio, such as fibrous particles, the CNT particles, asbestos, and nanowires, have been investigated in previous research (Seto et al. Citation2010; Wang et al. Citation2011a,Citationb; Bahk et al. Citation2013; Wang and Pui Citation2013; Chen et al. Citation2014; Kuo et al. Citation2014; Son et al. Citation2014). There are four main mechanisms for the single fiber theory: (1) inertial impaction effect; (2) interception effect; (3) diffusion effect; (4) and interception effect of diffusing particles between the filter fiber and the particle (Hinds Citation1999). The size and morphology of particles are related to their dominant filtration mechanism. The interception mechanism becomes particularly important for penetration of particles with a high aspect ratio (Kim et al. Citation2009).
Particles with a high aspect ratio may tend to rotate in the flow field. Penetration characteristics of those particles vary depending on the orientation angle between the major axis of the fibrous particle and the direction of motion (Asgharian and Cheng Citation2002). The orientation angle of particles acts as an important variable to predict the collection efficiency by interception of fibrous particles. Seto et al. (Citation2010) investigated the penetration of the CNT particles through the polycarbonate membrane filter with inhomogeneity. The orientation angle was in the range of 47˚ to 59˚. According to the theory, filtration mechanisms, such as impaction, interception, diffusion, and gravity, were considered. Assuming that the CNT particles have a straight cylinder-shape, Wang et al. (Citation2011b) compared the theoretical and the experimental penetration of the CNT particles passing through the 20 sheets of a screen mesh filter. Three theoretical models related to the interception parameter were presented to predict penetration of the CNT particles. Bahk et al. (Citation2013) predicted the length of the CNT particles by combining the experimental data with the single fiber theory. Chen et al. (Citation2014) investigated the penetration characteristic of the CNT particles through a commercial fiberglass filter, two kinds of electrostatic filters, and a membrane filter. The results demonstrated that the penetration of the CNT particles through filter media depends on length variable, as well as on the bending, curling, and agglomeration of the CNT particles.
Unlike the CNT particles, metal nanowires have a straight morphology. Thus, metal nanowires can be considered as an appropriate test material to compare the experimental results with the theoretical prediction regarding the penetration of cylindrical particles. Son et al. (Citation2014) carried out theoretical prediction, numerical prediction, and experimental measurement of the penetration of silver nanowires through a screen filter with the solidity of 0.288. Both theoretical prediction and numerical simulation results showed that the interception of diffusing particles should be considered to obtain a better agreement with the experimental results.
There have been numerical studies on the penetration of fibrous particles and nanowires through a fiber filter or screen filter (Kim et al. Citation2009; Liu et al. Citation2011; Podgorski et al. Citation2011; Wang et al. Citation2011a; Son et al. Citation2014). In numerical simulations, the orientation angle and shape of a fibrous particle and geometric characteristics of the filter were considered. For example, Wang et al. (Citation2011a) carried out the numerical simulations considering the inertia impaction and diffusion of the CNT particles with orientation angle (≈ 40˚) and compared the experimental data for a single layer screen mesh filter with the numerical simulation results. CNT particles have a bent and kink shape and a nonuniform diameter. However, in numerical simulations, it was assumed that the CNT particles have a form of a cylinder. Son et al. (Citation2014) modeled a nanowire as a cylinder and considered the dynamic shape factor of silver nanowires according to their electrical mobility diameter in their numerical simulations. In simulations, three filtration mechanisms – namely, diffusion, interception, and interception of diffusing particles – were considered for the penetration of silver nanowires through a screen filter. The numerical simulation results indicate that the interception of diffusing particles is very important.
Up to now, filtration of nanowires through commercial fibrous filters has not been investigated. In the present study, as a continuity of Son et al. (Citation2014), we aimed to find out whether the single fiber theory considering the effect of interception of diffusing particles can be applied to predict the penetration of particles with a high aspect ratio through commercial filters. To this end, we investigated the penetration of silver nanowires through commercial polyester filters and compared our experimental results with the theoretical prediction and numerical simulation results. Polyester filters chosen in this study were a screen filter with a fine regular array and a fibrous filter with a complex and irregular array. Silver nanowires were used as a test material for our experiments, since silver nanowires have a straight morphology and can be modeled as a cylinder. In addition, the diameter of filter fibers and the length and diameter of silver nanowires were obtained by the SEM image analysis. The size range of silver nanowires was from 200 to 400 nm in the mobility diameter similar to the one used in Park et al. (Citation2014) study. The orientation angles were obtained in order to match the experimental data with the theoretical prediction for the penetration of silver nanowires through a commercial polyester filter. In addition, using the orientation angle obtained through fitting experimental data into theoretical model, we carried out numerical simulations on the penetration of silver nanowires through single layer of the screen filter or single layer of the fibrous filter, respectively, considering three particle collection mechanisms. Also, numerical simulations on the penetration of silver nanowires through 5 layers of screen filter was carried out and also the effect of the configuration of multiple layers of screen filter was investigated for the particle penetration through multi-layer of filter.
2. Experimental methods
To measure the penetration efficiency of silver nanowires through commercial filters, it is necessary to generate monodisperse silver nanowire particles. Silver nanowires used in the experiments were synthesized in the polyol reduction process. Details about the synthesis process were explained in Son et al. (Citation2014). Silver nanowires dispersed in DI water were generated in an unaggregated state by a Collison-type atomizer (Son et al. Citation2014). Also, the penetration efficiency of spherical particles was measured and the potassium chloride (KCl) particles were also selected as test material. Figure S1 (see the online supplementary files [SI]) shows the unclassified silver nanowire particles and potassium chloride particles size distribution at the entrance of the test section with a filter chamber and a dummy chamber.
Figure S2a in the SI shows the experimental set-up for the penetration tests for the commercial filters. The commercial polyester filters were used in penetration tests. Before the particles passed through the polyester filter, they passed through a diffusion dryer, a neutralizer (Am-241), and a DMA. Then, particles were classified by the DMA depending on the mobility diameter (). DMA sheath flow was set to 6 LPM and the flow rate through a test chamber was set to 1.5 LPM by the CPC. The face velocity of the polyester filter was set to 5 cm/s. The corresponding particle Reynolds number is equal to 0.114, which belongs to creeping flow region (Re < 1). The length of test section for each chamber along the flow direction is 25 cm and the residence time is equal to 5 seconds. Under the conditions, the concentration and residence time of particles were not enough for the coagulation of particles. In Figure S1, the mode diameter of silver nanowires is 140.7 nm with 2.08 × 104 #/cc. For the reason, DMA classified silver nanowires and KCl particles were all well-dispersed individual particles. The amount of the doubly charged nanowires was estimated with the theoretically predicted length of the nanowire (see the SI). The length of the doubly charged particles was beyond the range of the length histogram presented in Figure S3.
The classified particles passing through the dummy chamber and the chamber with the polyester filter were alternately measured with CPC. The sampling time was set to 90 s. The penetration of particles classified by DMA is defined as the ratio of particle concentrations after passing through the filter chamber to the particle concentrations in the dummy chamber (see Equation (1)).[1] where
is experimental penetration,
is number concentration of the classified particle after passing through a filter chamber with the polyester filter and
is number concentration of the classified particle in the dummy chamber. For the mobility diameter of 200 to 400 nm, the concentration of particles was measured to be in range from about 1 × 103 to about 2 × 104 #/cm3 at the exit of a dummy chamber. Figure S2b shows experimental set-up for sampling particles. Monodisperse nanowires in the size range of the mobility diameter from 200 nm to 400 nm were sampled on the SI substrates using a particle sampler (HCT-4650).
Figure S5 shows the SEM images of the unclassified and monodisperse silver nanowires (= 200 nm, 250 nm, 300 nm, 350 nm, 400 nm), respectively. The average length and diameter of the nanowires were measured by using Image J software. Figure S5 shows that unlike the CNT particles with bent and kinks, silver nanowires have a cylindrical shape. This will be helpful to compare the experimental data with the theoretical prediction and numerical simulation results. Figure S3 shows the histograms of the average length and diameter of monodisperse silver nanowires. The average length of the nanowires for each mobility diameter was determined by the analysis of 60 SEM images using Image J software. We determined the average diameter of nanowires from 80 SEM images of polydisperse nanowires which were not classified since the diameter is independent of classified size. The diameter of nanowires was controlled in the synthesis of nanowires using the polyol reduction process. Therefore, Figure S3f provides the overall statistics of the diameter and the average diameter was 49.3 nm. For each mobility diameter, we obtained the average length and aspect ratio. The average length was from 1034 to 2022 nm and the aspect ratio (
) was from 20.9 to 41 for
= 200 nm, 250 nm, 300 nm, 350 nm, and 400 nm.
In our experiments, polyester filters were immersed in Isopropyl alcohol (IPA) for 5 min in order to remove the static charge on both fibrous polyester and screen polyester filters, the polyester filters were tested in our experiments varying the number of layers, i.e., single layer, 5 layers, 10 layers, and 15 layers. shows representative SEM images of two different types of polyester filters. The fiber diameters of the polyester filters were uniform. The primary parameters of the polyester filters were obtained based on the SEM image analysis results and measurements using a micrometer and a micro balance. Table S3 in the SI shows the parameters of each polyester filter. The solidity () of filter is defined by Cheng and Yeh (Citation1980) as follows (see Equation (2)):
[2]
The pressure drop across a clean filter has been proposed by a previous experimental study (Thomas et al. Citation1999). The presented relations are based on the Darcy law, the drag coefficient of a filter fiber (), and the length of all fiber (
). The total length of the fiber is related to the filter thickness and the fiber diameter as follows:
[3]
The pressure drop is expressed as:[4] where
is the gas dynamic viscosity (kg−s/m2),
is filtration face velocity (m/s),
is filter thickness (m),
is diameter of a filter fiber (m), and
is the dimensionless drag coefficient. Previous studies reported different models regarding the dimensionless drag coefficient (Davies, Citation1952; Kuwabara Citation1959; see Equations (Equation5
[5] )–(Equation6
[6] )).
[5]
[6]
Davies' empirical formula for pressure drop across a clean filter was provided for the conditions of 1.6m <
< 80
m and 0.006 <
< 0.3.
3. Theory for filtration
Similar to Son et al. (Citation2014) approach, the single fiber filtration theory was used in the present study for the theoretical prediction. The theoretical penetration was obtained using Equation (Equation7[7] ) (Hinds Citation1999).
[7] where
is theoretical penetration,
is solidity of the filter,
is filter thickness,
is the number of layers,
is the average diameter of a filter fiber, and
is the sum of the efficiency by inertial impaction, interception, interception of diffusing particles, and diffusion. The total efficiency is defined as:
[8] where
is efficiency by inertia impaction,
is efficiency by interception,
is efficiency by interception of diffusing particles, and
is efficiency by diffusion.
The efficiency by impaction, interception, diffusion and interception of diffusing particles for the case of nanowires can be calculated using interception parameter,, which takes account of the morphology of nanowires. For the case of spherical particles, the interception parameter
is considered for the filtration efficiency by interception. For the case of the particles with a high aspect ratio, it is necessary to consider the particle morphology in the theoretical model. Instead of R, the corrected interception parameter
is applied to the filtration efficiency by interception and the interception of diffusing particles. The interception parameter
is defined as follows (Bahk et al. Citation2013):
[9] where
is the length of silver nanowire and
is the orientation angle between the major axis of the nanowire and the direction of motion.
4. Numerical method
The filtration efficiency of the single layer of the screen filter and the single layer of the fibrous filter were numerically obtained by using the commercial CFD code FLUENT v. 16.0 solver (ANSYS, Inc.). Following Wang et al. (Citation2011a) and Son et al. (Citation2014) approach, the single layer of the screen filter was modeled by placing two vertical cylinders with the fiber diameter of the filter and a gap between each fiber for the CFD simulations as shown in Figure S6. The distance between each fiber in the screen filter was 3.65m. In the case of the fibrous filter, it is difficult to model a real configuration of the fibrous filter since it has very irregular and complex morphology. For the reason, the fibrous filter was also modeled to have a regular array similarly to the case of a screen filter using the solidity and fiber diameter of the fibrous filter. The distance between the fibrous filter fibers was modeled to be 61
m based on the thickness and solidity of the fibrous filter.
Previous studies focused on the penetration of nanoparitcles through single layer of screen filter. On the other hand, in the present study, the penetration of the nanowires passing through the multi-layer of filters was numerically investigated. Since it is difficult to standardize the relative orientation of each layer, we modeled five layers of screen filter in two different ways as shown in . shows the uniform arrangement of screen filters. shows the arrangement of screen filters when each layer has mirrored orientation to each other. In the case of 5 layers of screen filter, the distance between each fiber was the same as that in the single layer of screen filter and each layer was modeled to be apart by 27.8 m based on the measurement results.
Figure 2. Modeled 5 layers of screen filter: (a) the uniform arrangement and (b) the arrangement when each layer has mirrored orientation to each other.
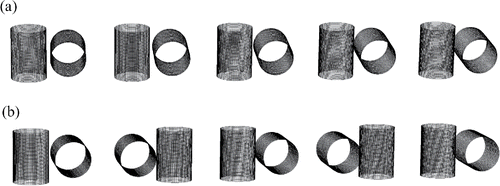
For the numerical simulations of the flow field (stead-state and laminar flow), boundary conditions were face velocity 5 cm/s at the inlet and outflow at the outlet and symmetry on four sides of the domain. The grid numbers used in the CFD simulations were about 0.89 million elements for both single layer of the screen filter and l layer of the fibrous filter, respectively. And in case of 5 layers of screen filter, the gird numbers were about 2.35 million.
In the case of single layer, the grid convergence was checked by comparing the pressure drop obtained from the numerical simulations with the theoretically predicted pressure drop. In the case of single layer of the screen filter, the pressure drop from numerical simulations was 11.92 Pa and it is close to 12.24 Pa from theoretical prediction. In the case of single layer of the fibrous filter, the pressure drop from the numerical simulations was 3.52 Pa, which was close to 3.57 Pa from the theoretical prediction.
In the case of 5 layer of screen filter, we carried out the grid independence tests for pressure dropin order to check the grid convergence. The grid numbers used in the grid independence tests were from about 1.08 to 3.01 million elements. The grid convergence was met when about 2.35 million elements were used resulting in the pressure drop equal to 34.69 Pa.
The impaction, diffusion, interception, and interception of diffusing particles were considered as the primary mechanisms of particle capture by a filter fiber. Each mechanism was computed by the method used in Son et al. (Citation2014). The collection efficiency of diffusion can be obtained by solving the convection diffusion equation defined as:[10] where
is the i th component of the flow velocity and C is particle concentration User Defined Scalar of FLUENT is used for applying the convection equation. In order to solve the equation, boundary conditions are
at the inlet,
on the filter surface and
at the outlet (Wang et al. Citation2011a).
The collection efficiency of interception and interception of diffusing particles was computed by using the Discrete Phase Model (DPM) solving the equation of particle motion. Following Son et al. (Citation2014) approach, the particle length in the DPM trajectories was considered as, not Lw and the slip correction calculated by relationship suggested by Son et al. (Citation2014) should be considered in DPM. By considering the distance between filter fiber and nanowire in user-defined function (Wang et al. Citation2011a), the relationship of interception effect can be taken into account.
22,000 silver nanowire particles were uniformly injected over the inlet surface. Since the inertial impaction effect was found to be negligible, the total penetration can be expressed as follows (Hinds, Citation1999; see Equation (Equation11[11] )):
[11] where
is the total penetration,
is the penetration by diffusion,
is penetration by interception, and
is penetration by interception of diffusing particles. For the penetration by interception
, the Brownian diffusion effect was not considered. On the other hand, for the penetration by interception of diffusing particles, the Brownian diffusion effect was considered.
5. Results and discussions
In order to investigate the effect of the type of polyester filter on filtration efficiency, we measured the penetration of KCl particles passing through the screen filter and the fibrous filter, respectively. As shown in Figure S7, taking into account of the interception of the diffusing particles effect, our model shows good agreement with the experimental results. For both screen and fibrous filter, the orientation angle of 40˚ was used.
Based on the results reported by Li et al. (Citation2012) and Park et al. (Citation2014), silver nanowires inside the DMA have fully aligned orientation in our experiments. The theoretically predicted penetration should be obtained considering the morphology of nanowires. shows the theoretical prediction and the experimental results for both the fibrous filter and the screen filter when the orientation angle of 40˚ was used as in previous studies (Wang et al. Citation2011b; Son et al. Citation2014). The theoretical prediction took into account the efficiency by diffusion, interception, and interception of diffusing particles. In the theoretical prediction, the filtration efficiency by interception and interception of diffusing particles was obtained with corrected interception parameter. It appears that there is a good agreement between the theoretical prediction and the experimental results for the case of screen filter. However, there is a significant difference between the theoretical prediction and the experimental results for the case of the fibrous filter. This indicates that a different orientation angle needs to be used for the fibrous filter.
Figure 3. The penetration of nanowires through the fibrous filter and the screen filter when the orientation angle of 40˚ is used.
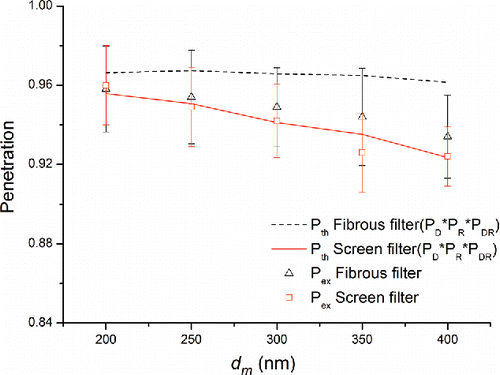
As shown in , the screen filter has a regular array while the fibrous filter has inhomogeneous array. For the reason, it is necessary to investigate the relationship between inhomogeneity and the orientation angle. In our experiments, the orientation angles were obtained by fitting the experimental results into the theoretically predicted penetration based on the single fiber theory.
Figure S8 (see the SI) shows experimental penetration according to the number of layers. summarizes the orientation angles of both the screen filter and the fibrous filter for experimental results shown in Figure S8. Son et al. (Citation2014) show that for the case of multiple layers of the screen filter, e.g., 10, 20, and 30 layers, the orientation angles are below 40˚ but there was no tendency with the increasing number of filter layer. Similar to their findings, in our experimental results for the multiple layers, e.g., 5, 10, and 15 layers, the average orientation angles were below 40˚. Thus, it is difficult to determine the typical orientation angle for the case of multiple layers of the filters. In addition, the orientation angle of a single layer was larger than that of multiple layers. In each case of multiple layers, the mean orientation angle of the fibrous filter was larger than that of the screen filter.
Table 1. The orientation angle based on the experimental data.
In the case of single layer of the screen filter with the solidity of 0.505 and fiber diameter of 34 m, the orientation angles are in the range of 36.38˚ to 48.58˚ (). This agrees well with the specific value 40˚ in the screen filter with the solidity of 0.345 (Wang et al. Citation2011b), in the range of 33˚ to 51˚ in the screen filter with the solidity of 0.288 and fiber diameter of 37.5
m (Son et al. Citation2014).
In the case of the fibrous filter, the orientation angles obtained by fitting the experimental data based on the single fiber efficiency theory are in the range of 70˚ to 80˚. The reason for the significant difference in the orientation angle between previous studies and our study needs to be found. The orientation angle could be affected by the solidity and fiber diameter of the filters. However, the fibrous filter used in our experiments has the solidity of 0.278 and fiber diameter of 34 m. They are very similar to the solidity and fiber diameter of the screen filter used in Son et al. (Citation2014). The orientation angle of fibrous nanoparticles could be influenced by the aspect ratio of particles. However, the aspect ratios of silver nanowires used in our experiments are similar to those with the aspect ratio in the range of 14.35 to 30.98 in Son et al. (Citation2014). Despite the above mentioned facts, there is still a significant difference between the results of our study and those reported by Son et al. (Citation2014). The only difference between Son et al. (Citation2014) and our study is the type of the filters used in experiments. We used the fibrous filter while Son et al. (Citation2014) used the mesh filter. Thus, we can infer that the orientation angle of nanowires passing through the single layer of the fibrous filter can be affected by the complex and irregular structure of the fibrous filter.
Previously, Seto et al. (Citation2010) investigated the filtration of the MWCNT particles through the fibrous filter. Seto et al. (Citation2010) used the fibrous filter with the solidity of 0.049 and fiber diameter of 2.8 m. The orientation angle in their study was in the range of 40˚ to 59˚ (closer to 40˚ compared to the orientation angle of the fibrous filter in the present study). The aspect ratio of the CNT particles in the range of the mobility diameter from 100 to 300 nm used in Seto et al (Citation2010) study was between 20.4 and 36.8. It is similar to the aspect ratios of the silver nanowires used in Son et al. (Citation2014). Son et al. (Citation2104) used silver nanowires in the range of the mobility diameter from 200 to 400 nm which, correspondingly, had the aspect ratio of 14.35 to 30.98.
Since test materials in Seto et al. (Citation2010) study are different from ours, it would be difficult to make a direct comparison. Nevertheless, while Seto et al. (Citation2010) show that their experimental data can be well fitted with the orientation angle close to 40˚ but our results can be fitted well with the orientation angle in the range from 70˚ to 80˚. We think that the significant difference in the orientation angle is due to the fact that the solidity and fiber diameter of our fibrous filter are much larger than those used in Seto et al. (Citation2010) study.
show the penetration of nanowires through 1 layer of screen filter and fibrous filter. Particle trajectories of silver nanowire (= 250 nm) for the
with Brownian diffusion are shown in Figure S9. In the case of the screen filter, the orientation angle 40˚, which was presented in previous studies (Wang et al. Citation2011b; Son et al. Citation2014), was used in the theoretical prediction and numerical simulations. In the case of the fibrous filter, the orientation angle in the range from 70˚ to 80˚ was used for the theoretical prediction and numerical simulations. In , the magnitude of penetration decreases with the increase of the mobility diameter. The numerical simulation results significantly deviate from the experimental data only when the diffusion and interception effects are considered. In Table S4, both theoretical prediction and numerical simulation show that due to the interception effect the penetration of nanowires through both filters decreases as the mobility diameter increases. In our experiments, the aspect ratio of nanowires increases from 32.2 to 41 for the range of the mobility diameter of 300 to 400 nm.
Figure 4. The penetrations: (a) 1 layer of screen filter ( = 40˚) and (b) 1 layer of fibrous filter.
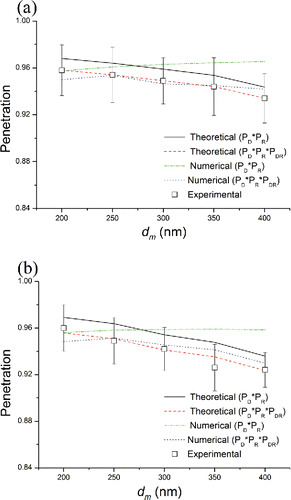
In both theoretical prediction and numerical simulation results, the predictions taking into account the interception of diffusing particles, in addition to diffusion and interception, show a better agreement with the experimental results as compared to when interception of diffusing particles effect is not considered. Our results confirmed that the three-dimensional numerical model suggested by Wang et al. (Citation2011a) and Son et al. (Citation2014) can be used in the numerical prediction of penetration of silver nanowires through the fibrous filter with an irregular fiber array as well.
Figure S10 shows the particle trajectories of silver nanowire (= 250 nm) for
with Brownian diffusion at each model of 5 layers (). The blue solid lines represent trajectories of noncaptured particles and the other solid lines represent trajectories of captured particles by filter fibers, respectively. The orientation angle obtained by fitting experimental data of 5 layers of screen filter into theoretical model was used in numerical simulations.
shows the penetration results for each model of 5 layers of screen filter from our experiments and numerical simulations. In numerical simulations, the interception of diffusing particles, diffusion, and interception effect were taken into account. For all cases of numerical simulations, the orientation angle obtained from experimental data was used. From numerical simulation results, the arrangement of 5 layers of screen filters does not affect the penetration results. Also, the numerical simulation results are in very good agreement with experimental results. Although the arrangement of 5 layers of screen filters were randomly changed in our repeat experiments, our experimental results were not affected. Thus, our numerical prediction and experimental data for the penetration of nanowires through 5 layers of screen filter indicate that the penetration of silver nanowires through multi-layer of screen filter is not affected by the arrangement of layers.
It should be noted that the numerical simulation results for single layer of each filter and 5 layers of screen filter have a good agreement with experimental results when the solidity of filter and the orientation angle obtained by fitting experimental data were used in numerical simulations. Three-dimensional numerical models suggested by Wang et al. (Citation2011a) and Son et al. (Citation2014) are effective to predict penetration of silver nanowires through multi-layer of fibrous filter when the solidity and structure of filter, when using the filter fiber diameter, solidity and thickness of filter are known.
6. Conclusions
In this study, the theoretical, numerical, and experiment studies were carried out regarding the penetration of silver nanowires through two types of polyester filters: the screen filter and the fibrous filter. The silver nanowires were classified using DMA in the size range of 200 nm to 400 nm as the mobility diameter increased by 50 nm. In the experiments, we used the screen filter with a regular array of filter fibers and the fibrous filter with an irregular array of filter fibers. Overall, theoretical and numerical predictions taking into account of interception, diffusion, and interception of diffusing particles agree very well with the experimental results.
The orientation angles of silver nanowires passing through the single layer and multi-layers of polyester filter were obtained by fitting experimental data. In cases of single layer, the orientation angle of silver nanowires passing through the screen filter is very close to the average orientation angle of 40˚ reported by Wang et al. (Citation2011b) and in the range from 33˚ to 51˚ reported by Son et al. (Citation2014). Seto et al. (Citation2010) show that the orientation angle for the case of the fibrous filter with relatively low solidity is close to 40˚. However, in our experiments for the case of the fibrous filter with relatively high solidity, the orientation angle is in the range from 70˚ to 80˚, which is larger than the orientation angle in previous studies. The fibrous filter with relatively high solidity is likely to have a larger orientation angle than the screen filter. Although the screen filters with homogeneity has relatively high solidity, the orientation angle is still close to 40˚. Thus, it indicates that the orientation angle obtained by fitting experimental data is affected by structure of filter more than solidity.
Also, we experimentally investigated the penetration of silver nanowires through the multiple layers of both screen and fibrous filters. The orientation angle of nanowires passing through the multiple layers for both the screen and the fibrous filters is smaller than the orientation angle of nanowires passing through the single layer.
Numerical predictions for the penetration of silver nanowires passing through single polyester filter and 5 layers of screen filter were carried out using orientation angle obtained through a fitting process of experimental data. Numerical prediction results show good agreement with experimental data and theoretical prediction. Our results show that the three-dimensional numerical models for the prediction of penetration of silver nanowires are effective when the filter fiber diameter, solidity and thickness of filter are known.
UAST_1258113__Supplemental_file.zip
Download Zip (4.1 MB)Acknowledgments
The authors thank Prof. Jing Wang at ETH for helpful discussions.
Funding
This work was conducted under the framework of Research and Development Program of the Korea Institute of Energy Research (KIER) (B6-2440).
References
- Asgharian, B. and Cheng, Y. S. (2002). The Filtration of Fibrous Aerosols. Aerosol Sci. Technol., 36:10–17.
- Bahk, Y. K., Buha, J., and Wang, J. (2013). Determination of Geometrical Length of Airborne Carbon Nanotubes by Electron Microscopy, Model Calculation, and Filtration Method. Aerosol Sci. Technol., 47:776–784.
- Chen, S.-C., Wang, J., Bahk, Y. K., Fissan, H., and Pui, D. Y. H. (2014). Carbon Nanotube Penetration Through Fiberglass and Electret Respirator Filter and Nuclepore Filter Media: Experiments and Models. Aerosol Sci, Technol., 8:997–1008.
- Cheng, Y. S., and Yeh, H. C. (1980). Theory of a Screen-type Diffusion Battery. J. Aerosol Sci., 11:313–320.
- Davies, C. N. (1952). The Separation of Airborne Dust and Mist Particles. Proc. Inst. Mech. Engng., 1B:185–198.
- Hinds, W. C. (1999). Aerosol Technology: Properties, Behavior, and Measurement of Airborne Particles. John Wiley & Sons, New York, NY, pp. 190–195.
- Ji, Z., Wang, X., Zhang, H., Lin, S., Meng, H., Sun, B., Grorge, S., Xia, T., Nel, A. E., and Zink, J. I. (2012). Designed synthesis of CeO2 Nanorods and Nanowires for Studying Toxicological Effects of High Aspect Ratio Nanomaterials. Acs Nano., 6:5366–5380.
- Kim, S. C., Wang, J., Emery, M. S., Shin, W. G., Mulholland, G. W., and Pui, D. Y. H. (2009). Structural Property Effect of Nanoparticle Agglomerates on Particle Penetration through Fibrous Filter. Aerosol Sci. Technol., 43:344–355.
- Kuo, Y. Y., Bruno, F. C., and Wang, J. (2014). Filtration Performance Against Nanoparticles by Electrospun Nylon-6 Media Containing Ultrathin Nanofibers. Aerosol Sci. Technol., 48:1332–1344.
- Kuwabara, S. (1959). The Forces Experienced by Randomly Distributed Parallel Circular Cylinders or Spheres in a Viscous Flow at Small Reynolds Numbers. J. Phys. Soc. Japan, 14:527–532.
- Li, M., Mulholland, G. W., and Zachariah, M. R. (2012). The Effect of Orientation on the Mobility and Dynamic Shape Factor of Charged Axially Symmetric Particles in an Electric Field. Aerosol Sci. Technol., 46:1035–1044.
- Liu, J., Pui, D. Y., and Wang, J. (2011). Removal of Airborne Nanoparticles by Membrane Coated Filters. Sci. Total Environ., 409(22):4868–4874.
- Park, M., Li, M., Shin, W. G., Moon, H. J., and Ko, S. H. (2014). Electrical Mobility of Silver Nanowires in Transition and Continuum Regimes. J. Aer. Sci., 72:21–31.
- Podgorski, A., Maißer, A., Szymanski, W. W., Jackiewicz, A., and Gradon, L. (2011). Penetration of Monodisperse, Singly Charged Nanoparticles through Polydisperse Fibrous Filters. Aerosol Sci. Technol., 45:215–233.
- Seto, T., Furukawa, T., Otani, Y., Uchida, K., and Endo, S. (2010). Filtration of Multi-Walled Carbon Nanotube Aerosol by Fibrous Filters. Aerosol Sci. Technol., 44:734–740.
- Son, M., Park, H., Park, M., Wang, J., and Shin, W. G. (2014). Silver Nanowire Penetration Through Screen Filter. Aerosol Sci. Technol., 48:480–488.
- Thomas, D., Contal, P., Renaudin, V., Penicot, P., Leclerc, D., and Vendel, J. (1999). Modelling pressure drop in HEPA filters during dynamic filtration. J. Aerosol Sci., 30:235–246.
- Wang, C., and Otani, Y. (2012). Removal of Nanoparticles from Gas Streams by Fibrous Filters: A Review. Ind. Engineering Chem. Res., 52:5–17.
- Wang, J., Kim, S. C., and Pui, D. Y. H. (2011a). Carbon Nanotube Penetration Through a Screen Filter: Numerical Modeling and Comparison with Experiments. Aerosol Sci. Technol., 45:443–452.
- Wang, J., Kim, S. C., and Pui, D. Y. H. (2011b). Measurement of Multi-wall Carbon Nanotube Penetration Through a Screen Filter and Single-fiber Analysis. J. Nanopart. Res., 13(10):4565–4573.
- Wang, J., and Pui, D. Y. (2013). Dispersion and Filtration of Carbon Nanotubes (CNTs) and Measurement of Nanoparticle Agglomerates in Diesel Exhaust. Chem. Eng. Sci., 85:69–76.