ABSTRACT
The impact of necking and overlapping on the radiative properties of coated soot aggregates was investigated numerically by using the Discrete Dipole Approximation (DDA). The present study concerns the situations of slight overlapping between primary particles and small to moderate necking. The effects of overlapping, necking, and coating on the aggregate volume equivalent radius were presented. To show the overlapping effect, the radiative properties of aggregates consisting of N = 200 particles were evaluated with and without coating at refractive indices of m = 1.60 + 0.60i for the soot core and m = 1.46 for the coating material at four different wavelengths in the visible and near-infrared. The radiative properties of coated soot aggregates with three overlapping values of 0.05, 0.1, and 0.2 were calculated. In addition, the relationship between absorption cross-section and wavelength was illustrated at overlapping values of 0.05, 0.1, and 0.2 for uncoated and 25%, 50%, 75%, and 100% coated aggregates. As overlapping and necking increased, the calculated extinction, absorption, and scattering properties also increased in the visible and near-infrared regions. It was found that the volume equivalent radii of coated aggregates increased linearly with coating thickness when the necking values were 0.40–0.50.
Copyright © 2017 American Association for Aerosol Research
EDITOR:
1. Introduction
Freshly emitted soot goes through an aging process in the atmosphere, resulting in a change in its radiative properties. This is the result of absorption and condensation of gases and volatiles on the soot surface. The aging process depends on the concentrations of internally mixed aerosols, organic matter, and sulfates. Soot particles go through a mixing process through the condensation of water vapor and other volatile chemicals present in the atmosphere. Uncoated freshly emitted soot aggregates, which exist in the form of particles, are hydrophobic. Therefore, they do not absorb a significant amount of water vapor under low humidity conditions during the atmospheric aging process. On the other hand, coated soot aggregates, formed as a result of atmospheric aging, become more hydrophilic (Mikhailov et al. Citation2001; Schnaiter et al. Citation2005; Adachi et al. Citation2007; Olfert et al. Citation2007; Khalizov et al. Citation2009a,Citationb).
It takes about a week for the composition, mass, and morphology of soot aerosols to be considerably altered. Sulfuric acid molecules readily condense on soot particles upon exposure because of their sticky nature and then the condensed sulfuric acid is stabilized by the interaction with water vapor. This causes the condensation process to be practically irreversible under typical atmospheric conditions. The water surface tension tends to make the particle a dense compact shape by collapsing the chain-like (or lacy) structure. This process was shown by the Scanning Electron Microscopy (SEM) images (Lu et al. Citation2008). The sulfuric acid condensation rate depends on the particle surface area and size but is independent of the morphology or chemical composition of aerosols (Mikhailov et al. Citation2006; Zhang et al. Citation2008). After internal mixing with sulfuric acid and compaction of aggregates, the soot particles interact collectively with electromagnetic waves, resulting in stronger scattering. The mixed particles are denser than dry hydrophobic and hydrophilic soot. The formation of an aqueous medium and compaction of the soot particles both contribute to the increase of the optical properties of coated soot particles. Similar results were found in the experimental study performed by Miljevic et al. (Citation2012), who studied the change in the mobility diameter for six different combustion sources when exposed to different organic materials and water vapor.
Modeling of the optical properties of the aging soot aerosols has been performed for three mixing states: externally mixed, core–shell structure, and internally mixed. The internally mixed state models were sometimes approximated by the Maxwell–Garnett and Bruggeman effective medium models (Zhang et al. Citation2015). Adachi et al. (Citation2010) investigated the mixing states and three-dimensional shapes of soot particles in the host material by using electron tomography. They found that the particles of aggregates are located mostly off-center of the coated aggregates. The organic materials initially condensed on the surfaces of soot particles and then filled the spaces between the branches of the aggregate. Consequently, although the volume of lightly coated soot particles has increased significantly, the final outer dimension of coated soot aggregates remains nearly the same as that of the uncoated soot aggregates. Black carbon particles with a coating of oleic acid and glycerol were experimentally researched by Shiraiwa et al. (Citation2010) for three different core diameters with various coating thicknesses. The enhanced absorption of coated particles over uncoated ones was calculated using Mie theory. The authors found that the absorption cross-section increased with increasing the coating thickness. Similar results for coated soot aggregates of core–shell structure with a coating thickness of 5 nm calculated with DDA were obtained by Soewono and Rogak (Citation2013). Bueno et al. (Citation2011) investigated the radiative properties of uncoated and coated soot aggregates generated by a diffusion flame using a Santoro style burner and measured the absorption cross-sections with photoacoustic spectroscopy using a laser at 0.405 μm experimentally. They compared the results with the scattering and absorption cross-sections predicted by Mie theory. In their study, the three core sizes of 100, 150, and 200 nm with the same coating thickness at two refractive indexes of m = 1.409 + 0.811i and m = 1.386 + 0.719i were considered in the core–shell model. Kahnert et al. (Citation2012) calculated the optical cross-sections of the soot aggregates with kf = 1.2 and Df = 2.6 encapsulated into sulfate shells with two different volume fractions and three different refractive indices by using DDA. The authors also calculated the effective refractive index for modeling the internally homogenous mixtures of soot aerosols with sulfate. The variation of the scattering properties of internally mixed aggregates consisting of one or more hygroscopic compounds was studied by Freney et al. (Citation2010) and the results were analyzed by concerning transmission electron microscopy (TEM) images.
The shapes and positions of embedded soot particles are numerous and depend on many factors such as aging, host materials, atmospheric conditions, temperature, and relative humidity. Wu et al. (Citation2015) studied the optical properties of semi-embedded mixtures and sulfate host with semi-externally, externally, and internally mixed aggregates consisting of N = 200 primary particles using the Superposition T-Matrix (STM) method in the UV and near infrared (IR) regions. They used refractive indexes of m = 1.76 + 0.57i and m = 1.44 for soot aggregates and sulfate, respectively. The number of particles and the soot size were assumed to be constant. According to comparisons of the results from DDA and STM, they found good agreement between the two methods for the optical cross-sections of semi-embedded soot-containing mixtures with non-intersecting surfaces. In a numerical study performed by Kandilian et al. (Citation2015), six fractal dimensions between 2.0 and 3.0 were considered with the number of primary particles in the range of 2 to 1000. All particles in the aggregate were in point-contact, i.e., without overlapping. They calculated the radiative properties by STM and compared the results with those of three other methods: the Rayleigh—Debye–Gans (RDG) method (Dobbins and Megaridis Citation1992), the Latimer's coated-sphere approximations, and the equivalent volume and average projected area coated-sphere approximation. The authors found that in all cases, the absorption cross-section was proportional to the number of primary particles. They also found that the absorption cross-section was independent of fractal pre-factor and dimensions and the absorption cross-section of randomly oriented fractal aggregates were proportional to their total volume.
Scarnato et al. (Citation2013) studied uncoated and coated black carbon (soot) aggregates having the same size with m = 1.95–0.79i. They calculated the optical properties of samples using DDA for uncoated aggregates in the wavelength ranges of 0.2–1.0 μm. In their study, two kinds of coated aggregates were investigated. The first was black carbon aggregates with partial surface contact with NaCl and the second was black carbon aggregates with total inclusion in NaCl. In an experimental study performed by Bambha et al. (Citation2013), the effects of volatile coatings and coating-removal mechanisms on the morphology of soot aggregates were investigated and compared with TEM images. Zhang et al. (Citation2015) calculated the optical properties of black carbon coated with sulfate aerosol in the ultraviolet, visible, and IR regions using three different mixing models: the core–shell, Maxwell–Garnettt, and Bruggeman effective medium methods. The optical properties of aerosol particles were determined by the BBC-RAD program. They researched the variation of heat transfer ratio for the three different mixing methods. The optical properties of mixed aerosols were strongly affected by the mixing method, the volume ratio of black carbon to sulfate, and the relative humidity. The authors concluded that there were no apparent differences between the Maxwell–Garnett and Bruggeman methods for all of the aerosol mixtures, while there were large differences between the core–shell and Maxwell–Garnett/Bruggeman methods.
The effects of primary particle overlapping on the morphology of soot aggregates were formulated by Oh and Sorensen (Citation1997). The overlap parameter was defined as δ = 2a/l, where l is the distance between the centers of two touching primary particles and a is radius of primary particles. Another overlap parameter for two touching particles was defined by Brasil et al. (Citation1999) as Cov = (2a − l)/2a. Schmid et al. (Citation2004) studied the effects of primary particle overlapping, described as sintering, and analyzed the three-dimensional morphology through simulation. The authors tried to define a general rule for overlapping based on the rate of approach of particles toward each other. Overlapping, which is also called surface growth, was investigated by Al Zaitone et al. (Citation2009) using a Monte Carlo algorithm. The effect of overlapping on the time derivative of fractal aggregates was studied by Eggersdorfer and Pratsinis (Citation2013). In their approach, overlapping was inseparable of a neck growth at the contact region of point-touch particles. In order to separate the two effects, Bescond et al. (Citation2013) described two parameters, overlapping and necking, as a utility mechanism that could be used in the analysis of radiative properties of coated soot aggregates. The overlapping parameter (Cov) refers the connection of particles observed in freshly emitted soot aggregates and was defined in the same way as that of Brasil et al. (Citation1999). Necking, which was described by a 3D level-set function driven by the parameter α, accounts for the accumulation of compounds in the contact region between neighboring primary particles. The reader is invited to read the papers by Bescond et al. (Citation2013), Bescond et al. (Citation2014), or Yon et al. (Citation2015) to have some illustrations of the role played by both Cov and α parameters. Based on an explanation by Bescond et al. (Citation2013) and Dong et al. (Citation2015), necking can be understood as filling the voids of the aggregate branches by condensation of organic materials due to their high viscosity properties. Litton and Perera (Citation2014) analyzed the radiative properties of soot aggregates with and without primary particle overlapping by using RDG and DDA. In their study, calculations were conducted for four different refractive indexes of m = 1.692 + ki (where k = 0.25, 0.50, 0.75, 1.25) and at wavelengths of λ = 0.532 and 0.635 μm. They compared the predicted and experimental results of soot obtained from polypropylene and diesel fuel. The authors found the best agreement between experimental and theoretical results for overlapping primary particles. Yon et al. (Citation2015) investigated the effects of overlapping and necking on the radiative properties of soot aggregates using DDA over the spectral range of 0.266–1.064 μm and compared the values with experimental results. They developed a program code that defines the location of dipoles for the calculation of coated soot aggregates with overlapping and necking. Their definition of overlapping was based on the formulation of Bescond et al. (Citation2013). The magnitudes of overlapping and necking are described by overlapping and necking parameters. Liu et al. (Citation2016) studied the effects of coating for four soot aggregates at a spectral range of 266–1064 nm with the spectrally dependent refractive index of soot in detail. They concluded that the absorption enhancement of coated soot aggregates is strongly depended on the coating thickness and wavelength but nearly independent of the size of uncoated aggregates. These findings about absorption and scattering properties of coated aggregates are similar to those obtained by Dong et al. (Citation2015) and Wu et al. (Citation2016). It should be pointed out that condensation of organics onto soot particles, characterized by necking, occurs in atmospheric aging. In the present study, the effects of necking at small overlapping parameters and overlapping on the radiative properties of soot at different coating thicknesses of non-absorbing materials were investigated using DDA.
2. Discrete dipole approximation
The computations of the absorption and scattering cross-sections for the coated aggregate particles are performed by using the discrete dipole approximation (DDA). The scattering matrix elements are obtained by solving the volume integral formulation of Maxwell's equations in DDA. The DDA models the shape and composition of aggregates as a set of dipoles on a lattice. The model computes the intensity of scattered electromagnetic radiation for a multitude of different orientations of aggregate with the incident beam and averages the scattered intensities over these multiple orientations to generate an average angular intensity distribution. The dipole spacing must be small compared with the electric field wavelength within the feature (Draine and Goodman Citation1993; Draine and Flatau Citation1994; Shen et al. Citation2008). When the target is a strong absorber, accurate calculations of the differential scattering cross-section require that |m|kd < 0.32 should be satisfied (Kahnert et al. Citation2012), where d is the inter-dipole lattice spacing, m is the refractive index, λ is the wavelength, and k = 2π/λ is the wave number. Thus, the model has the maximum lattice spacing allowable for a particular material and wavelength, and the number of dipoles required to model a feature increases in proportion to its size. The direct evaluation of the matrix equations in DDA requires O(Np2) operations, where Np is the total number of dipoles used (Ayrancı et al. Citation2007). The differential scattering cross-section is defined as the energy scattered per unit time and per solid angle for a given direction.
The DDSCAT code (Draine and Flatau Citation2014), which is a particular implementation of DDA, calculates extinction, absorption, and scattering efficiencies (Qext, Qabs, and Qsca). The volume-equivalent radius (aeff) of an aggregate is calculated as [aeff = N1/3a] for each aggregate formed by primary particles in point-touch, here N and a are the total number and radius of primary particles, respectively. In other cases, such as aggregates formed by overlapping primary particles or with coating, aeff is evaluated based on the total volume of the aggregates written in the generic expression, i.e., aeff = (3V/4π)1/3, here V is the total volume of the aggregate with or without coating. Therefore, the optical cross sections (μm−2) can be obtained by Equations Equation(1)–(3):[1]
[2]
[3]
3. Results and discussion
In the present study, DDA was selected due to its flexibility to calculate the scattering properties of a complex structure with arbitrary geometry, as described by Soewono and Rogak (Citation2013), Yon et al. (Citation2015), Liu et al. (Citation2016), and Um and McFarquhar (Citation2013), among other researchers. The DDSCAT version 7.3 (Draine and Flatau Citation2014) was used to calculate the radiative properties of coated soot aggregates. The absorption, scattering, and extinction cross-sections of coated aggregates consisting of N = 200 primary particles with different levels of overlapping and necking were investigated. The input parameters of the target geometry were set to ensure that the dipole distance d confirmed the criterion |m|kd < 0.32. This was ensured by considering a dipole density of 10 (the mean number of dipoles along a primary particle diameter). The refractive index of aggregates, the wavelength of incident electromagnetic radiation, the orientation averages of the aggregates, and the scattering planes were used as DDSCAT input. In DDSCAT calculation of coated aggregates, a shape file is needed to specify the location of dipoles. A code developed by Yon et al. (Citation2015) was used to produce the shape file, which specifies the dipole position and material type for each coated aggregate, and the FROM_FILE option in DDSCAT was activated.
Wavelength is an important parameter, and therefore, four wavelengths, λ = 0.44, 0.67, 0.87, and 1.02 μm, were considered in this study. These wavelengths cover the visible and near-infrared spectrum, which is highly relevant to optical diagnostic of soot and solar radiation by coated soot particles in the atmosphere. The orientation average was calculated for beta (β) from 0 to 360°, theta (θ) from 0 to 180°, and phi (Φ) from 0 to 360°. The Euler angles were averaged over 7 × 7 × 7 orientations, which were found sufficient to obtain orientation-averaged properties. The densities of core material and coating material were 1543 kg/m3 and 1227 kg/m3, respectively.
The accuracy of DDSCAT was tested on the absorption cross-sections of uncoated aggregates formed by point-touch primary particles, for which predictions of the STM are known as exact solutions (Cheng et al. Citation2014). The STM calculates the total scattered electromagnetic field at any given location by summing the contribution from each particle using a program developed by Mackowski and Mishchenko (Citation1996, Citation2011). They used a wide range of particle sizes, shapes, and morphologies by taking the values defined by Bond and Bergstrom (Citation2006). The accuracy of the results of radiative properties of coated soot aggregates was determined by using the refractive index values of m = 1.75 + 0.63i and m = 1.44 for sulfate coating in our research, as was the case in a study by Cheng et al. (Citation2014). The values for the number of primary particles of 200 and the radius of primary particles of 20 nm are the same as those in Cheng et al. (Citation2014). While the fractal dimension (Df) used in this study was 2.1 and the fractal pre-factor (kf) was 2.3, Cheng et al. (Citation2014) considered a range of 2.0–3.0 for the fractal dimension and 1.2 as the fractal pre-factor. A previous study performed by Oh and Sorenson (Citation1997) concluded that the parameters kf and Df increased with the degree of overlapping. However, Cheng et al. (Citation2014) concluded that the fractal dimension does not change with the coating thickness in the aging procedure. Kandilian et al. (Citation2015) also found that the absorption cross-sections of coated aggregates did not depend on the fractal pre-factor or fractal dimension. In the reference coated case, the primary particles in the internally mixed coated aggregate are in point-touch, i.e., without overlapping. Therefore, the case without overlapping was used in the analysis of the accuracy of DDA.
In our study, the impact of overlapping and necking on the morphological features of internally mixed soot aggregates was investigated. Views of the virtual aggregate with N = 200 before atmospheric aging, i.e., without coating, are shown in from different angles. The aggregate was generated using a tuneable cluster-cluster algorithm as described by Liu and Snelling (Citation2008), which was based on the algorithms developed by Filippov et al. (Citation2000). The volume equivalent radii (aeff) of the uncoated aggregate for different necking (α) values between 0.05 and 0.5 at different overlapping parameters are given in . The values of aeff of coated aggregates in the range of necking parameters of 0.25–0.5 for small overlapping Cov = 0.002, 0.01, 0.05, and Cov = 0.1 are presented in , respectively. The coating thickness values used in the figures represent the thickness of the coating with respect to the primary particle diameter. The coating thickness is defined as δ = p × dp, where p is the percentage of coating ranges in 0%–100% and dp is the primary particle diameter. Further details of how coating is added to an aggregate can be found in Liu et al. (Citation2016).
Figure 1. Views from three different angles of the soot particle aggregates for N = 200 particles (dp = 40 nm, aeff = 0.11696 μm, Df = 2.1, kf = 2.3, Cov = 0.0, α = 0.0) before atmospheric aging.
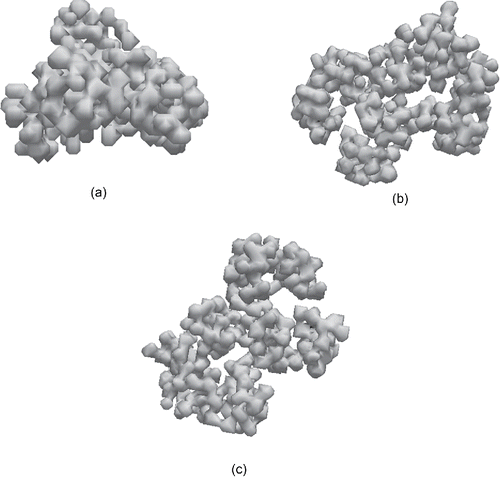
Figure 2. Comparison between the volume equivalent radii of coated aggregates for α range of 0.25–0.5: (a) for Cov = 0.002, (b) for Cov = 0.01, (c) for Cov = 0.05, and (d) for Cov = 0.1.
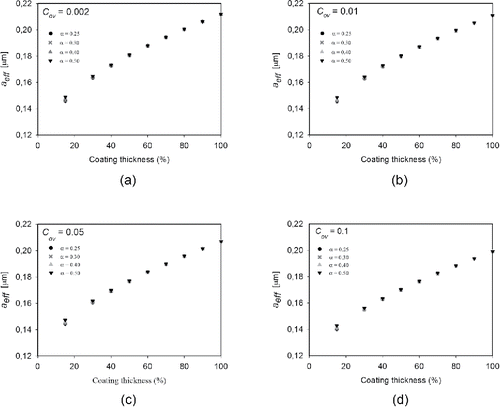
Table 1. The volume equivalent radius values of uncoated aggregates for different overlapping and necking.
3.1. Effects of necking
To investigate of necking effects, uncoated and coated soot aggregates were separately evaluated in this section. First, the uncoated soot aggregates for small overlapping were investigated and obtained aeff values showed in . The results given in should be analyzed in two sections. The necking effects in the range of α = 0.05–0.2 are in the first section and the necking effects in the range of α = 0.25–0.5 are in the second section. In the first section, it was observed that the particles were still in point-touch in uncoated soot aggregates and the geometry of the aggregate did not change. In the second section, it was shown that the overlapping and necking parameters influence the compactness of the soot core.
Similarly, the coated soot aggregates for small overlapping were studied in order to show the necking effects in the range of α = 0.25–0.5, because this necking range is more effective on compactness. show the volume equivalent radii of coated aggregates for overlapping parameter of Cov = 0.002, 0.01, 0.05, and 0.1, respectively. Upon coating of particles by aerosol components, the aeff values increase. As can be seen from , the aeff values of coated aggregates are strongly affected by the coating thickness. The coating thickness characterizes the overall geometrical size of coated aggregates.
It is shown that the aeff values of coated soot aggregates increase with increasing coating thickness due to the increase in the total mass and volume. Adachi et al. (Citation2010) found similar results related to the effects of condensation on the surfaces of soot particles, which prevented the particles from becoming compact. This situation can be seen from . Qiu et al. (Citation2012) reported the soot aging for OH-initiated oxidation of toluene and published TEM images of the changed morphologies of fresh and coated soot particles as shown in and . Pósfai et al. (Citation1999) studied three sets of aerosol samples that were internally mixed with sulfate and published TEM images of aerosol particles as shown in . It was found that as the aging time increased, the volume equivalent radii of coated soot increased (). The structure and the morphology of the soot aggregates were changed and became more compact when the necking values were larger. The aeff values of coated aggregates increase with the coating thickness. Moreover, the volume equivalent radii were found to be similar for the same coating thickness when the necking values were in the range 0.40–0.50. The experimental evidence shown in and , where the dimensions of the original fresh soot and coated aggregate are shown similar, supports the numerical results. Similar findings were reported by Khalizov et al. (Citation2009b), who found that H2SO4-coated soot aggregates display different hygroscopic growth patterns depending on their initial size. As the coating thickness increased, the particles inside the coated aggregates were encapsulated by other aerosol components and surrounded by medium. Finally, the heavily coated aggregates had more compact morphology, which affected their radiative properties (Adachi et al. Citation2010). It can be seen from that at 15% coating, the average relative changes of aeff between coated and uncoated aggregates were 20.32%, 20.1%, 19.4%, and 17% in the necking range of α = 0.25–0.5 when the overlapping parameter is changed from Cov = 0.002 to 0.1. Similarly, when we compared the 50% coated aggregates with the uncoated aggregates in the necking effect range of α = 0.25–0.5, the average relative increase in aeff is found to be 35.32%, 35.01%, 33.9%, and 31.2% when the overlapping parameter is increased from Cov = 0.002 to 0.1. The findings are in good agreement with the experiments of Freney et al. (Citation2010). This study is the first numerical analysis of the relationship between the coating thickness and the aeff values of coated aggregates for small values of overlapping and necking.
Figure 3. TEM images of changes in morphology: (a) fresh soot; (b) coated soot (Qiu et al. Citation2012); (c) a typical chain-like soot aggregate; the arrows point to a carbon film that connects individual spherules within the aggregate (Pósfai et al. Citation1999); (d) particles containing KCl and K2SO4, whose emissions from biomass-burning take up water at 83% (Freney et al. Citation2010); (e) particles collected from an inland area and exposed to humidity (Freney et al. Citation2010).
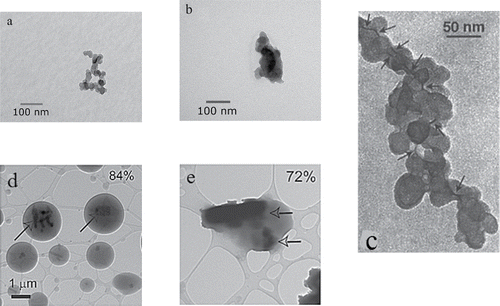
The values obtained in our study showed that the results shown in for the calculated aeff values of coated aggregates decreased slightly with increasing degree of overlapping for the same necking parameter and the same coating thickness. For example, the aeff values at 50% coating thickness at α = 0.4 are 0.1809 μm (), 0.1800 μm (), 0.1769 μm (), and 0.1702 μm () for Cov = 0.002, 0.01, 0.05, and 0.1, respectively. Experimental studies by Litton and Perera (Citation2014) produced similar results. They reported that an increase in the overlapping values resulted in a decrease in the size of coated aggregates, which is in agreement with the results of this research. In order to illustrate the heavily coated aggregates, two samples collected from two different environments (coastal and inland sites) are presented in and (Freney et al. Citation2010). It is evident that necking values of 0.4 or 0.5 in all the studied overlapping situations provided better results for the aeff values of coated soot aggregates, which are similar to the cases shown in and .
From the results shown in , it can be observed that the variability of the overlapping and necking with increasing thickness of coated aggregates is similar. According to a recent detailed study performed by Yon et al. (Citation2015), an increase in overlapping causes a decrease not only in the size of coated aggregates but also in their masses. However, an increase in necking leads to an increase in the mass and in the volume effective radii of the aggregates. The results shown in confirmed these influences of overlapping and necking.
3.2. Effects of overlapping
This research investigated non-overlapping and three levels of low overlapping, Cov = 0.002, 0.01, and 0.05. The necking value of α = 0.20 was taken for a coating thickness of less than 50% and α = 0.40 was taken for a coating thickness of more than 50%. The effect of coating on the radiative properties of coated aggregates was calculated by Cheng et al. (Citation2014) using, where they considered the volume-equivalent shell/core ratios (S/C) of 1.5 and 2.0 using values of Df = 2.4 and 2.8 for thinly coated and heavily coated aggregates, respectively. The accuracy of results of radiative properties of coated soot aggregates was determined by using the refractive index value of m = 1.75 + 0.63i and m = 1.44 for sulfate coating in our research as was the case in Cheng et al. (Citation2014). Comparisons of the radiative properties of coated aggregates without overlapping are presented in . The small overlapping cases are shown in . In these figures, black, blue, and red lines represent fresh soot, thinly coated aggregate, and heavily coated aggregate, respectively. It can be seen from that coating has a significant influence on absorption at wavelengths in the visible and near IR ranges. In addition, the effect of overlapping seems quite small for the narrow range of Cov investigated.
Figure 4. Comparison of the absorption cross-sections at different overlapping values for uncoated and coated soot aggregates.
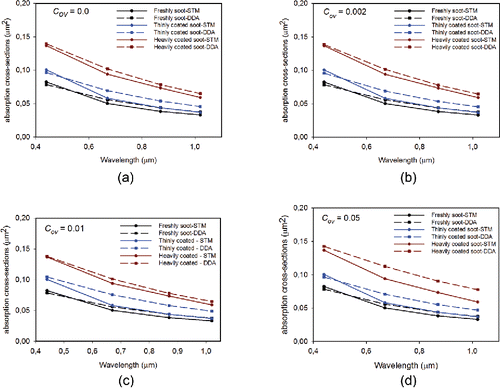
In this study, absorption cross-section values were calculated by using different coating thicknesses. The results are listed in . Considering the thinly coated aggregate, the average relative changes of the absorption cross-section between uncoated and coated aggregates were found to be 11.8%, 11.9%, and 13.9% when the overlapping changed from Cov = 0.002 to 0.05. Similarly, when we compared the heavily coated with the uncoated and coated aggregates, the average relative deviations of absorption cross-section were found to be 5.7%, 5.5%, and 15.8% when overlapping changed from Cov = 0.002 to 0.05. The differences between the DDA results and those of STM are also attributed to the slight difference in the aggregate morphology, in addition to the difference in numerical methods.
Table 2. Values of relative difference of the calculations of DDA for small overlapping cases.
As can be seen from and , increasing the coating thickness of coated soot aggregates has considerable effects on the absorption characteristics. However, the effect of overlapping is fairly small for the small range of overlapping considered. For the same coating thickness and necking, increasing overlapping results in higher absorption values with about 10%–15% increase under the present conditions. It was concluded that the coating thickness is a very important parameter.
In the second part of the study, the refractive index values of m = 1.60 + 0.60i for soot aggregate and the refractive index values of m = 1.46 for organic volatile matter were used. The results of absorption cross-sections and single scattering albedo for the studied overlapping cases are given in and for Cov = 0.05, (c) and (d) for Cov = 0.1, and (e) and (f) for Cov = 0.2. As the values of overlapping and wavelength increase, these radiative properties of coated aggregates decrease. For overlapping of Cov = 0.1, the absorption cross-section increases by 19.5%, 26.5%, 37.7%, and 41.2% for the four studied coating thickness of 25%, 50%, 75%, and 100%, respectively. Similarly, for overlapping of Cov = 0.2, the absorption cross-section increases by 12.3%, 19%, 29.9%, and 33.7% as the coating thickness increases. As the degree of overlapping of coated aggregates increases, the average relative deviation in the absorption cross-section decreases, as can be seen in and . The single scattering albedos (SSA) of the coated soot aggregates for Cov = 0.05, 0.1, and 0.2 are also shown in . This parameter was found strongly affected by overlapping and necking (Yon et al. Citation2015; Liu et al. Citation2016). As the coating thickness increases, SSA also increases over the entire wavelength range considered, as can be seen from and . The average relative variations in SSA are 46%, 58%, 61%, and 66% for the coating thicknesses of 25%, 50%, 75%, and 100% at Cov = 0.1. For Cov = 0.2 the average relative increases in SSA are 41%, 54%, 59%, and 63% for the coating thickness of 25%, 50%, 75%, and 100%, respectively. It is also found that SSA decreases with increasing overlapping. In addition, it is observed that the scattering cross-sections are greater than the absorption cross-sections at 0.44 μm, i.e., SSA > 0.5, when the coating thickness is 50% or larger.
4. Conclusions
In this study, the effects of primary particle overlapping and necking on the volume-equivalent radii and radiative properties of coated soot aggregates were investigated numerically by using the discrete dipole approximation (DDA). Selected necking and overlapping values for the calculation of radiative properties could lead to maximum 15% average differences. The absorption cross-section of the coated aggregate decreases while increasing overlapping between the core aggregate primary spheres and wavelength. As the overlapping increases, the coated aggregates become more compact. For each value of overlapping as the coating thickness increases, both the absorption cross-section and the single-scattering albedo increase in the visible and the near-infrared. Another interesting result is that as the overlapping and necking increase, the calculated absorption and the other radiative properties increase at wavelengths in the visible and near-IR regions. Further research is required to improve our understanding of the effects of coating under different conditions of coating thickness and the position of the soot core within the coating material when it is fully encapsulated.
Funding
This work was supported by the Scientific and Technological Research Council of Turkey.
References
- Adachi, K., Chung, S. H., and Buseck, P. R. (2010). Shapes of Soot Aerosols Particles and Implications for Their Effects on Climate. J. Geophys. Res., 115:D15206.
- Adachi, K., Chung, S. H., Friedrich, H., and Buseck, P. R. (2007). Fractal Parameters of Individual Soot Particles Determined Using Electron Tomography: Implications for Optical Properties. J. Geophys. Res., 112:D14202.
- Al Zaitone, B., Schmid, H.-J., and Peukert, W. (2009). Simulation of Structure and Mobility of Aggregates Formed by Simultaneous Coagulation, Sintering and Surface Growth. J. Aerosol Sci., 40:950–964.
- Ayrancı, I., Vaillon, R., Selçuk, N. (2007). Performance of Discrete Dipole Approximation for Prediction of Amplitude and Phase of Electromagnetic Scattering by Particles. J. Quant. Spectrosc. Radiat. Transf., 103:83–101.
- Bambha, R. P., Dansson, M. A., Schrader, P. E., and Michelsen, H. A. (2013). Effects of Volatile Coatings and Coating Removal Mechanisms on the Morphology of Graphitic Soot. Carbon, 61:80–96.
- Bescond, A., Yon, J., Girasole, T., Jouen, C., Rozé, C., and Coppalle, A. (2013). Numerical Investigation of the Possibility to Determine the Primary Particle Size of Fractal Aggregates by Measuring Light Depolarization. J. Quant. Spectrosc. Radiat. Transf., 126:130–139.
- Bescond, A., Yon, J., Ouf, F.-X., Ferry, D., Delhaye, D., Gaffié, D., Coppalle, A., and Rozé, C. (2014). Automated Determination of Aggregate Primary Particle Size Distribution by TEM Image Analysis: Application to Soot. Aerosol Sci. Technol., 48:831–841.
- Bond, T. C., and Bergstrom, R. W. (2006). Light Absorption by Carbonaceous Particles: an Investigative Review. Aerosol Sci. Technol., 40:27–67.
- Brasil, A. M., Farias, T. L., and Carvalho, M. G. (1999). A Recipe for Image Characterization of Fractal-Like Aggregates. J. Aerosol Sci., 30:1379–1389.
- Bueno, P., Havey, D., Mulholland, G. W., Hodges, J. T., Gillis, K., Dickerson, R. R., and Zachariah, M. R. (2011). Photoacoustic Measurements of Amplification of the Absorption Cross Section for Coated Soot Aerosols. Aerosol Sci. Technol., 45:1217–1230.
- Cheng, T., Wu, Y., and Chen, H. (2014). Effects of Morphology on the Radiative Properties of Internally Mixed Light Absorbing Carbon Aerosols With Different Aging Status. Opt. Exp., 22:15904–15917.
- Dobbins, R., and Megaridis, C. (1992). Absorption and Scattering of Light by Polydisperse Aggregates. Appl. Opt., 30:4747–4754.
- Dong, J., Zhao, J. M., and Liu, L. H. (2015). Morphological Effects on the Radiative Properties of Soot Aerosols in Different Internally Mixing States With Sulfate. J. Quant. Spectrosc. Radiat. Transf., 165:43–55.
- Draine, B. T., and Flatau, P. J. (1994). Discrete-Dipole Approximation for Scattering Calculations. J. Opt. Soc. Am., 11:1491–1499.
- Draine, B. T., and Flatau, P. J. (2014). User Guide to the Discrete Dipole Approximation Code DDSCAT 7.3. Available at http://arxiv.org/abs/1002.1505
- Draine, B. T., and Goodman, J. (1993). Beyond Clausius–Mossotti-Wave Propagation on a Polarizable Point Lattice and the Discrete Dipole Approximation. Astrophys. J., 405:685–697.
- Eggersdorfer, M. L., and Pratsinis, S. E. (2013). Restructuring of Aggregates and Their Primary Particle Size Distribution During Sintering. AIChE J., 59:1118–1126.
- Filippov, A. V., Zurita, M., and Rosner, D. E. (2000). Fractal-Like Aggregates: Relation Between Morphology and Physical Properties. J. Colloid Interf. Sci., 229:261–273.
- Freney, E., Adachi, K., and Buseck, P. (2010). Internally Mixed Atmospheric Aerosol Particles: Hygroscopic Growth and Light Scattering. J. Geophys. Res., 115:D19210.
- Kahnert, M., Nousiainen, T., Lindqvist, H., and Ebert, M. (2012). Optical Properties of Light Absorbing Carbon Aggregates Mixed with Sulfate: Assessment of Different Model Geometries for Climate Forcing Calculations. Opt. Exp., 20:10042–10058.
- Kandilian, R., Heng, R. L., and Pilon, L. (2015). Absorption and Scattering by Fractal Aggregates and by their Equivalent Coated Spheres. J. Quant. Spectrosc. Radiat. Transf., 151:310–326.
- Khalizov, A., Xue, H., Wang, L., Zheng, J., and Zhang, R. (2009a). Enhanced Light Absorption and Scattering by Carbon Soot Aerosol Internally Mixed Sulfuric Acid. J. Phys. Chem., A113:1066–1074.
- Khalizov, A., Zhang, R., Zhang, D., Xue, H., Pagels, J., and McMurry, P. H. (2009b). Formation of Highly Hygroscopic Soot Aerosols upon Internal Mixing With Sulfuric Acid Vapor. J. Geophys. Res., 114:D05208.
- Litton, C. D., and Perera, I. E. (2014). Modeling the Optical Properties of Combustion-Generated Fractal Aggregates. Fuel, 130:215–220.
- Liu, F., and Snelling, D. R. (2008). Evaluation of the Accuracy the RDG Approximation for the Absorption and Scattering Properties of Fractal Aggregates of Flame-Generated Soot. in 40th Thermophysics Conference, 23–26 June, Seattle, Washington, USA.
- Liu, F., Yon, J., and Bescond, A. (2016). On the Radiative Properties of Soot Aggregates-Part 2: Effects of Coating. J. Quant. Spectrosc. Radiat. Transf., 172:134–145.
- Lu, Z., Hao, J., Hu, L., and Takekawa, H. (2008). The Compaction of Soot Particles Generated by Spark Discharge in the Propene Ozonolysis System. J. Aerosol Sci., 39:897–903.
- Mackowski, D. W., and Mishchenko, M. I. (1996). Calculation of the T-Matrix and the Scattering Matrix for Ensembles of Spheres. J. Opt. Soc. Am., A13:2266–2278.
- Mackowski, D. W., and Mishchenko, M. I. (2011). A Multiple Sphere T-Matrix Fortran Code for use on Parallel Computer Clusters. J. Quant. Spectrosc. Radiat. Transf., 112:2182–2192.
- Mikhailov, E. F., Vlasenko, S. S., Kramer, L., and Niessner, R. (2001). Interaction of Soot Aerosol Particles With Water Droplets: Influence of Surface Hydrophilicity. J. Aerosol Sci., 32:697–711.
- Mikhailov, E. F., Vlasenko, S. S., Podgorny, I. A., Ramanathan, V., and Corrigan, C. E. (2006). Optical Properties of Soot-Water Drop Agglomerates: An Experimental Study. J. Geophys. Res., 111:D07209.
- Miljevic, B., Surawski, N. C., Bostrom, T., and Ristovski, Z. D. (2012). Restructuring of Carbonaceous Particles upon Exposure to Organic and Water Vapours. J. Aerosol Sci., 47:48–57.
- Oh, C., and Sorensen, C. M. (1997). The Effect of Overlap between Monomers on the Determination of the Fractal Cluster Morphology. J. Colloid. Interf. Sci., 193:17–25.
- Olfert, J. S., Symonds, J. P. R., and Collings, N. (2007). The Effective Density and Fractal Dimension of Particles Emitted from a Light-Duty Diesel Vehicle With a Diesel Oxidation Catalyst. Aerosol Sci., 38:69–82.
- Pósfai, M., Anderson, J. R., Buseck, P. R., and Sievering, H. (1999). Soot and Sulfate Aerosol Particles in the Remote Marine Troposphere. J. Geophys. Res., 104:21685–21693.
- Qiu, C., Khalizov, A. F., and Zhang, R. (2012). Soot Aging from OH-Initiated Oxidation of Toluene. Environ. Sci. Technol., 46:9464–9472.
- Scarnato, B. V., Vahidinia, S., Richard, D. T., and Kirchstetter, T. W. (2013). Effects of Internal Mixing and Aggregate Morphology on Optical Properties of Black Carbon using a Discrete Dipole Approximation Model. Atmos. Chem. Phys., 13:5089–5101.
- Schmid, H.-J., Tejwani, S., Artelt, C., and Peukert, W. (2004). Monte Carlo Simulation of Aggregate Morphology for Simultaneous Coagulation and Sintering. J. Nanopart. Res., 6:613–626.
- Schnaiter, M., Linke, C., Möhler, O., Naumann, K.-H., Saathoff, H., Wagner, R., and Schurath, U. (2005). Absorption Amplification of Black Carbon Internally Mixed with Secondary Organic Aerosol. J. Geophys. Res., 110:D19204.
- Shen, Y., Draine, B. T., and Johnson, E. T. (2008). Modeling Porous Grains With Ballistic Aggregates. I. Geometry and Optical Properties. Astrophys. J., 689:260–275.
- Shiraiwa, M., Kondo, Y., Iwamoto, T., and Kita, K. (2010). Amplification of Light Absorption of Black Carbon by Organic Coating. Aerosol Sci. Technol., 44:46–54.
- Soewono, A., and Rogak, S. N. (2013). Morphology and Optical Properties of Numerically Simulated Soot Aggregates. Aerosol Sci. Technol., 47:267–274.
- Um, J., and McFarquhar, G. M. (2013). Optimal Numerical Methods for Determining the Orientation Averages of Single-Scattering Properties of Atmospheric Ice Crystals. J. Quant. Spectrosc. Radiat. Transf., 127:207–223.
- Wu, Y., Cheng, T., Zheng, L., and Chen, H. (2016). Models for the Optical Simulations of Fractal Aggregated Soot Particles Thinly Coated with Non-absorbing Aerosols. J. Quant. Spectrosc. Radiat. Transf., 182:1–11.
- Wu, Y., Cheng, T., Zheng, L., Chen, H., and Xu, H. (2015). Single Scattering Properties of Semi-Embedded Soot Morphologies With Intersecting and Non-Intersecting Surfaces of Absorbing Spheres and Non-Absorbing Host. J. Quant. Spectrosc. Radiat. Transf., 157:1–13.
- Yin, J. Y., and Liu, L. H. (2010). Influence of Complex Component and Particle Polydispersity on Radiative Properties of Soot Aggregate in Atmosphere. J. Quant. Spectrosc. Radiat. Transf., 111:2115–2126.
- Yon, J., Bescond, A., and Liu, F. (2015). On the Radiative Properties of Soot Aggregates Part 1: Necking and Overlapping. J. Quant. Spectrosc. Radiat. Transf., 162:197–206.
- Zhang, H., Zhou, C., Wang, Z., Zhao, S., and Li, J. (2015). The Influence of Different Black Carbon and Sulfate Mixing Methods on the Their Optical and Radiative Properties. J. Quant. Spectrosc. Radiat. Transf., 157:105–116.
- Zhang, R., Khalizov, A., Pagels, J., Zhang, D., Xue, H., and McMurry, H. (2008). Variability in Morphology, Hygroscopicity, and Optical Properties of Soot Aerosols during Atmospheric Processing. PNAS, 105:10291–10296.