ABSTRACT
Water-soluble organic matters (WSOMs) play an important role in determining magnitudes of climatic and environmental impacts of organic aerosol particles because of their contributions to hygroscopic growth and cloud formation. These processes are dependent on water solubility as well as distribution of this property in a particle, yet no method has been available to quantify such characteristics. In this study, we developed a theoretical framework to classify WSOM by 1-octanol-water partitioning that has a strong correlation with water solubility. 1-octanol-water partitioning coefficient also has a strong correlation with a traditional solid phase extraction method, facilitating interpretation of data from the technique. The theoretical analysis demonstrated that the distributions of WSOM classified by 1-octanol-water partitioning depend on (1) the volume ratio of 1-octanol and aqueous phases, and (2) extraction steps. The method was tested by using organic aerosol particles generated by smoldering of a mosquito coil, which serves as a surrogate for biomass burning particles. The WSOM extracted from the mosquito coil burning particles was classified by 1-octanol-water partitioning at different volume ratios. These solutions, including both the 1-octanol and aqueous phases, were nebulized to generate particles for measurements using an online aerosol mass spectrometer. The mass spectra indicated that highly oxygenated species tend to be highly soluble, while high molecular weight compounds are less soluble. Linear combinations of these mass spectra allowed the estimation of the mass fractions of WSOM partitioned to 1-octanol and aqueous phases, thereby facilitating the evaluation of the mass fractions of cloud condensation nuclei (CCN) active materials.
© 2017 American Association for Aerosol Research
EDITOR:
1. Introduction
Aerosol particles in the atmosphere affect both the global climate and local environments (Carslaw et al. Citation2010; Fiore et al. Citation2012). For instance, aerosol particles serve as cloud condensation nuclei (CCN) when water vapor is supersaturated, leading to the formation of cloud droplets (Farmer et al. Citation2015). Aerosol particles also influence visibility as well as the radiation budget of the Earth by scattering solar radiation (Ramanathan et al. Citation2001). This effect is particularly pronounced when these particles uptake water vapor, as hygroscopic growth increases diameter of particles (Yu et al. Citation2006; Wang et al. Citation2008). In addition, aerosol particles induce adverse health effects, including respiratory problems (Laumbach and Kipen Citation2012). The deposition pattern of aerosol particles in the human body depends on their hygroscopic growth properties because the diameter change in particles affects their dynamics (Londahl et al. Citation2008). Understanding the hygroscopic properties of aerosol particles is important for investigating their climatic and environmental impacts.
Hygroscopic properties as well as CCN activities of pure inorganic and organic compounds have been investigated by both theoretical and experimental approaches, demonstrating that water solubility (S) is a key parameter to control water uptake properties (Shulman et al. Citation1996; Petters and Kreidenweis Citation2008; Sullivan et al. Citation2009; Riipinen et al. Citation2015). These studies demonstrated that organic compounds can be classified to three categories, based on their water solubility (Sullivan et al. Citation2009; Kuwata et al. Citation2013). For instance, highly soluble compounds, including levoglucosan and malonic acid, are highly CCN active and hygroscopic (Prenni et al. Citation2001; Mochida and Kawamura Citation2004; Rosenorn et al. Citation2006). On the other hand, slightly soluble species such as adipic and pinonic acids are only slightly CCN active (Kuwata et al. Citation2013). The theoretical analysis of CCN activation mechanisms by the Köhler theory provides an explanation for the experimental results (Petters and Kreidenweis Citation2008; Kuwata et al. Citation2013; Riipinen et al. Citation2015). Highly soluble species deliquesce at subsaturated humidity (i.e., relative humidity [RH < 100%]) to form aqueous particles. On the other hand, slightly soluble species deliquesce only at the supersaturated regime (RH > 100%). Insoluble species (S < ∼10−4 [g cm−3]) exhibit no water uptake, while highly soluble species (S > ∼10−1 [g cm−3]) significantly contribute to water uptake. Compounds with marginal solubility (∼10−4 [g cm−3] < S < ∼10−1 [g cm−3]) are considered as slightly soluble. It is noted that these values separating different types of organic compounds have some variability, depending on particle size and mixing state (Petters and Kreidenweis Citation2008; Sullivan et al. Citation2009; Kuwata et al. Citation2013). A recent theoretical analysis demonstrated that the distribution of water solubility is important for CCN activity when multiple organic compounds exist in a single particle (Riipinen et al. Citation2015).
A significant fraction of atmospheric aerosol particles comprises organic compounds, which include tens of thousands of species (Hamilton et al. Citation2004; Jimenez et al. Citation2009). Classification of these organic compounds is crucial, as the complexity of the chemical composition inhibits identification at the molecular level (Hallquist et al. Citation2009). One of the most popular classifications is the separation of water soluble (WSOM) and insoluble organic matters (Psichoudaki and Pandis Citation2013). Further separation of WSOM is required to understand water uptake properties of organic aerosol particles, as it contains a broad spectrum of compounds (Kuwata et al. Citation2013; Psichoudaki and Pandis Citation2013). For this reason, separation of WSOM has been conducted using solid-phase extraction as well as high performance liquid chromatography techniques (Decesari et al. Citation2000; Sullivan and Weber Citation2006; Asa-Awuku et al. Citation2008; Polidori et al. Citation2008). WSOM extraction by multiple solvents was also found as a useful technique to separate them (Mihara and Mochida Citation2011). Although these techniques are demonstrated as useful in classifying WSOM, relevance between these classification methods and water solubility has been unclear, preventing the detailed theoretical analysis of water uptake properties. In addition, WSOM classifications by these techniques are not predictable, requiring a significant effort for characterizing methods (Sullivan and Weber Citation2006). Development of a method to infer solubility distribution of actual aerosol particles will be advantageous in promoting our understanding on solubility dependent water uptake properties of organic materials.
In this study, we explored the possibility to use 1-octanol-water partitioning as a method to classify WSOM by their water solubility (S). The purpose of the study is to provide a theoretical background to classify WSOM by 1-octanol-water partitioning, and qualitatively test the method using a complex mixture of organic compounds. Water solubility and 1-octanol-water partitioning coefficient (KOW) are tightly correlated, allowing separation of complex mixtures of organic compounds by water solubility. Unlike solid phase extraction methods, KOW can be reliably predicted using functional group contribution methods, allowing detailed interpretation of data without extensive characterizations (Meylan and Howard Citation1995; Meylan et al. Citation1996). A theoretical analysis is provided. The method is tested for organic material produced by smoldering of a mosquito coil, which has similar chemical characteristics as ambient biomass burning particles (Ng et al. Citation2011; Li et al. Citation2012). The result from the experiment using a mosquito coil qualitatively supports the theory, suggesting the further quantitative verification of the method, which includes experimental tests by standard chemicals, could be promising.
2. Theory
In this section, a theoretical background for the classification of organic compounds by 1-octanol-water partitioning is provided, after introducing relationships between KOW and S as well as notation for solutions after liquid–liquid extractions.
2.1. KOW–S relationship
The value of KOW of compound i (KOW_i) is defined by the following equation (Sangster Citation1989):[1] where
and
are the molar concentrations of compound i in the 1-octanol and aqueous phases, respectively. The correlation between KOW and S has been established both for molar and mass concentrations (Kenaga and Goring Citation1980; Chiou et al. Citation1982). In this study, we focus on KOW–S (mass concentration) relationship, as the unit is more useful in describing water uptake properties of aerosol particles. Several KOW–S relationships have been proposed using various experimental datasets, as compared in and (Kenaga and Goring Citation1980; Valvani et al. Citation1981; Coates et al. Citation1985; Isnard and Lambert Citation1989).
Figure 1. KOW–S relationships of organic compounds. Empirical relationships for these quantities are also shown ().
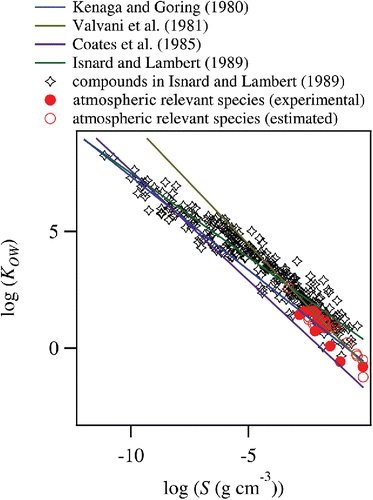
Table 1. The relationships between KOW and S proposed in literature. Unit conversion factors were applied to original equations so that S can be represented in g cm−3.
Due to the nature of empirical correlations, the proposed KOW–S relationships have some variability, especially for highly soluble (low KOW) compounds. To find the best KOW–S relationship for atmospheric application, the empirical correlations proposed by these studies were tested for atmospherically relevant compounds. The list of water-soluble atmospheric relevant compounds, which include both primary and secondary species, is provided in Table S1 (Yu et al. Citation1999; Graham et al. Citation2002). Experimental values for KOW and S were used when these values are available in literature (Yalkowsky and Dannenfelser Citation1992; Hansch et al. Citation1995; Yalkowsky and He Citation2003; Hartz et al. Citation2006). Functional group contribution methods such as KOWWIN v 1.68 and WSKOW v. 1.41, which are implemented in Estimations Programs Interface for Windows (EPI Suite) from the United States Environmental Protection Agency (US EPA), were employed when experimental data are not available (Meylan and Howard Citation1995; Meylan et al. Citation1996). Predicted values of KOW and S by these programs agreed well with these experimental data for atmospheric relevant chemical species (Figure S1). shows that Kenaga and Goring (Citation1980) provide the best estimates for KOW–S relationship for atmospheric relevant species. The parameterization by Kenaga and Goring (Citation1980) successfully reproduced S of the atmospheric relevant compounds (Table S1) from KOW within the error of Δlog S = 0.39 (mean squared error), providing an estimate of the uncertainty.
2.2. Notation for solutions extracted by 1-octanol-water partitioning
Numerous 1-octanol and aqueous solutions are produced, following liquid–liquid extractions by 1-octanol and water ().
Providing appropriate notations to these solutions will facilitate discussing characteristics of chemical species in them. An aqueous solution of WSOM is obtained by dissolving sampled aerosol particles in water using an offline (e.g., filter sampling) or online (e.g., Particle-into-Liquid-Sampler: PILS) technique (Weber et al. Citation2001). This solution is called as A0. 1-octanol is added to an aliquot of A0, classifying organic compounds by 1-octanol-water partitioning. The 1-octanol phase is named as O1A0, whereas the aqueous phase is called as A1A0. Organic compounds in O1A0 are further separated by adding water, while 1-octanol could be added to A1A0. The procedure can be repeated until the desired classification of organic compounds is achieved. In general, the notation of a solution following N extractions (XN: X is A or O) is given as follows:[2] where N is a positive integer. This notation rule is employed throughout the study.
2.3. Single extraction from aqueous solution (N = 1)
By employing the definition of molar concentration, KOW_i for the single extraction case is also represented as[3]
In the case of dilute solutions, the total volumes of the 1-octanol () and aqueous (
) phases are predominantly determined by the volumes of the solvents. The total number of moles of compound i in the original WSOM solution (
) is equivalent to the sum of the number of moles in the 1-octanol (
) and aqueous (
) phases.
[4]
Combining Equations (Equation3[3] ) and (Equation4
[4] ), the mole fractions of compound i in the 1-octanol (
) and aqueous (
) phases are represented as
[5] and
[6] respectively. In these equations, the volumetric ratio of 1-octanol and aqueous phases is defined as
. Both
and
are only dependent on KOW_i and
. The chemical characteristics of compound i determines KOW_i. On the other hand,
is a controllable variable, meaning that the classification of organic compounds can be regulated by this parameter.
As discussed in Section 2.1, KOW of atmospheric relevant compounds could be converted to S using the empirical relationship developed by Kenaga and Goring (Citation1980). Using the relationship (), Equations (Equation5[5] ) and (Equation6
[6] ) can be converted as follows to provide
and
as a function of Si (water solubility of compound i):
[7]
[8]
These equations quantitatively relate fi and water solubility.
shows some examples of and
, which are plotted as functions of KOW and S. Three different values of
(0.01, 0.1, and 1) are employed for the calculation. The calculated distributions of
and
have sigmoidal shapes when plotted in logarithmic scales. Compounds with lower values of KOW tend to partition to the aqueous phase, especially when the values of
are smaller ().
Figure 3. (a) Fractions of organic materials partitioning to 1-octanol and aqueous phases following single extraction, plotted against KOW. (b) Same as (a) but plotted against S.
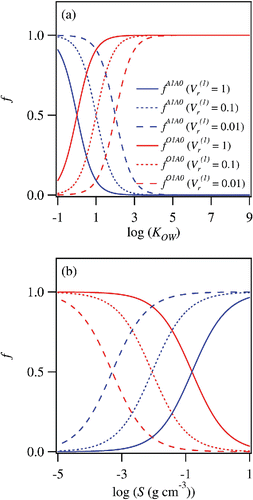
This trend is translated to the capability of 1-octanol-water partitioning as a solubility classifier (). When is equal to 1, the aqueous phase is enriched by organic compounds with high solubility (S > 10−1 [g cm−3]). On the other hand, a significant fraction of much less soluble species (S ∼ 10−3 [g cm−3]) could also partition to the aqueous phase for a lower value of
(
). This theoretical analysis demonstrates that 1-octanol-water partitioning is a useful tool to obtain solubility distribution of WSOM. The estimated distribution functions shown in are relatively broad due to the nature of a single extraction. The classification resolution would be improved by repeating extractions (multiple extractions) at the same values of
, although the method could be time and labor intensive.
2.4. Multiple extractions (N ≥ 2)
The classification resolution of organic compounds by 1-octanol-water partitioning is further improved by repeating the liquid–liquid extraction multiple times. The fractions of compound i in the 1-octanol and aqueous phases following the Nth extraction () are denoted as
and
, respectively. In the case of multiple extractions, these values can be related with KOW_i by the following equations:
[9]
[10]
In Equations (Equation9[9] ) and (Equation10
[10] ), r(N-1) is volume fraction of XN-1 solution, which is used for the Nth extraction. The value of r(N-1) is equal to unity when the whole part of XN−1 is used for the Nth extraction, whereas it is less than one if only a fraction of XN−1 is employed for the Nth extraction. Combinations of Equations (Equation9
[9] ), (Equation10
[10] ), and parameterization by Kenaga and Goring (Citation1980) yield
and
as functions of solubility.
[11]
[12]
shows examples of and
. The values of
are assumed as (
. The distributions of
, and
shown in are similar to
,
, and
shown in , respectively. On the other hand,
and
() are significantly different from
, and
shown in . The distributions of
and
are approximated well by lognormal distributions, and they do not resemble sigmoidal distributions any more. An important implication from this theoretical analysis is that the classification resolution by 1-octanol-water partitioning can be improved by repeating the liquid–liquid extraction multiple times.
3. Experiment
A mosquito coil, which does not contain any artificially synthesized compounds (Tennen Jochuugiku, Kicho-no-uzumaki, natural pyrethrum spiral, Kincho, Osaka, Japan), was burned in an enclosed 100-L stainless steel container. The container was equipped with an air inlet and outlet. Particle free air was continuously supplied from the air inlet. The chemical composition of aerosol particles emitted from the mosquito coil burning was measured using the Time-of-Flight Aerosol Chemical Speciation Monitor (ToF-ACSM; Aerodyne Research, Inc., Billerica, MA, USA) (Froehlich et al. Citation2013). Simultaneously, aerosol particles were sampled using two sets of 47-mm Teflon filters (P0450, Sigma-Aldrich, St. Louis, MO, USA) and filter holders (BGI, Inc., Butler, NJ, USA) at flowrates of 5 L min−1. The collected samples were stored in a freezer (−27°C) until analysis. A schematic diagram of the experimental setup is available in the online supplementary information (SI; Figure S2).
The filter samples were extracted using approximately 10 mL of purified water (Type I, 18.2 MΩ·cm) in an ultrasonic bath for 30 min. The resulting aqueous solution was filtered using a PTFE syringe filter (514-0070, VWR International, Radnor, PA, USA) to remove debris. The pore size of the filter is 0.2 µm. A fraction of the solution was nebulized for the ToF-ACSM analysis to obtain the mass spectrum of WSOM (A0). An aliquot of the aqueous solution was used for liquid–liquid extraction by 1-octanol (Wako first grade, Wako Pure Chemical Industries, Osaka, Japan). The volumes of the aqueous solution and 1-octanol were measured using syringes with metal needles. These liquids were mixed in a separation funnel. Only single extraction experiments were conducted. The values of were set as 0.01, 0.1, and 1. The separation funnel was vigorously shaken to form an emulsion. Subsequently, the funnel was placed at a funnel stand at least for an overnight period, separating 1-octanol and aqueous phases clearly. The 1-octanol and aqueous phases were separately sampled, and transferred to glass vials.
Subsequently, the 1-octanol solutions were diluted more than 10 times by methanol (ACS grade, 99.8%, VWR International). The aqueous phase solutions were used for analysis without any dilution. These solutions were nebulized using glass nebulizers. A mass flow controller (MC-20 SLPM-D, Alicat Scientific, Inc., Tucson, AZ, USA) was used to regulate the particle free air supplied to the nebulizer. Following nebulization, the solvents were removed using a diffusion dryer (Model 42000, Brechtel Manufacturing, Inc., Haywood, CA, USA) filled with activated charcoal (granular 6–10 mm, Wako Pure Chemical Industries Osaka, Japan) or silica gel (Chameleon 83000.360, VWR International). Charcoal was used for the 1-octanol solution diluted by methanol, whereas silica gel was employed for aqueous solutions. As methanol is a highly volatile solvent, it does not affect quantification by the ACSM. A trace amount of residual 1-octanol signal, which is negligibly small compared with that from mosquito coil burning particles, were observed after removal of organic vapor by charcoal (Figure S3). The chemical composition of organic aerosol particles was measured using the ToF-ACSM. The mass spectra measured by the ToF-ACSM were analyzed using a fragmentation table (Allan et al. Citation2004).
4. Results and discussion
4.1. Visual inspection of the 1-octanol and aqueous phases
shows a photo of O1A0 and A1A0 of mosquito coil burning particles, classified at = 0.01, 0.1, and 1. In general, light-absorbing species tend to partition to the 1-octanol phase. On the other hand, A1A0 (
= 1) is almost colorless, whereas A1A0 (
= 0.01) is slightly yellow. This result indicates that light-absorbing organic compounds are relatively less soluble because A1A0 (
= 1) should only contain highly soluble species ().
Figure 5. A photograph of 1-octanol (O1A0) and aqueous (A1A0) phases separated at = 0.01, 0.1, and 1. The samples were prepared by dissolving organic aerosol particles that were generated by mosquito coil burning in water. See the text for details.
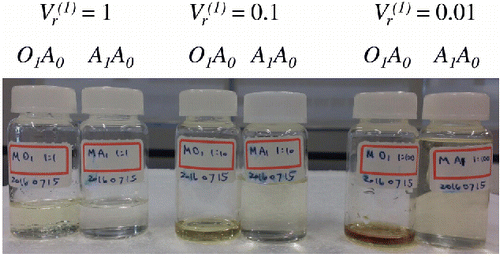
Light absorbing species in biomass burning particles is known to be dominantly composed of humic like substances (HULIS; Hoffer et al. Citation2006; Wu et al. Citation2016), which is frequently separated from aerosol samples using solid phase extraction techniques (Graber and Rudich Citation2006; Fan et al. Citation2013). One of the most commonly used materials for solid phase extraction of HULIS is XAD-8. The separation characteristics of organic compounds by XAD-8 are compared with KOW in . In general, organic compounds can easily penetrate XAD-8 column if the values of their KOW is smaller than unity (log KOW < 0). On the other hand, organic compounds with higher values of KOW (KOW > 1 or log KOW > 0) tend to stay on the surface of XAD-8 column. The experiment by Sullivan and Weber (Citation2006) demonstrated that fluvic and humic acids from Suwannee River, which serve as surrogate of HULIS, do not penetrate XAD-8, although KOW of these compounds are unknown, The implication of this experiment is that HULIS likely have relatively large values of KOW (KOW > 1). Thus, we hypothesize that HULIS in mosquito coil burning particles dominantly partitioned to 1-octanol phase, producing light-absorbing solutions.
4.2. ACSM mass spectra
shows the ACSM mass spectra of mosquito coil burning particles, which are (a) directly measured from the burning chamber (online) and (b) WSOM (A0). Ordinate of the figure is fractional contribution of ions at each m/z to the whole mass spectra. The online measurement data have a minor contribution of m/z44 signal and intense peaks of hydrocarbons, such as m/z55 (C4H7+) and 57 (C4H9+). Intensities of these ions indicate that mosquito coil burning particles are not highly oxygenated, as m/z44 is considered as a marker for degree of oxygenation (Ng et al. Citation2011). The marker ion for levoglucosan-like species (m/z60), which is known as an indicator of biomass burning particles, is clearly identified (Cubison et al. Citation2011). These results are qualitatively consistent with those of a previous study on mosquito coil burning particles (Li et al. Citation2012). However, some high m/z ions reported in the previous work (m/z121, 137, and 167) are not distinct in . The dissimilarity could be due to the differences in burning condition and/or types of mosquito coils. The ACSM mass spectrum of WSOM extracted from mosquito coil (A0) has a higher fraction of m/z44 than that obtained in the online measurement. In addition, the intensities of the hydrocarbon peaks are much less than those in the online data. These results show that the WSOM fraction is more oxygenated. The fraction of high-molecular weight ions (m/z > 100) for A0 is less than that for the online measurement, implying that most of high molecular weight species are insoluble in water.
Figure 6. Normalized mass spectra of organic materials quantified by the ToF-ACSM. (a) Online measurement and (b) WSOM.
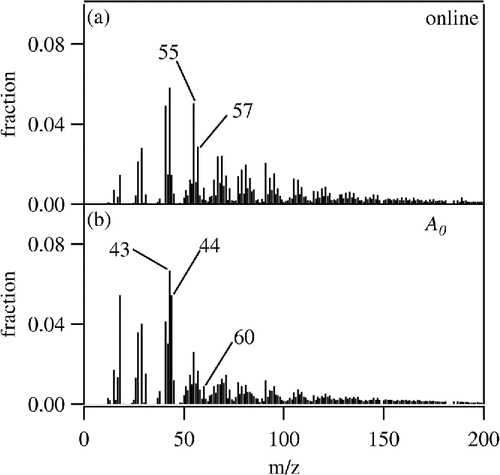
shows the mass spectra of A1A0 and O1A0 separated at different values of. The most significant signal for A1A0 (
= 1) is m/z44, indicating that the organic material in this fraction is highly oxygenated (Ng et al. Citation2011). The mass spectrum also shows a strong signal at m/z60, implying the significant abundance of levoglucosan-like species (Cubison et al. Citation2011). On the other hand, the m/z44 signal for O1A0 (
= 1) is much less pronounced than that for A1A0 (
= 1). The intensity of the marker ion for levoglucosan-like species (m/z60) is also almost negligible for O1A0 (
= 1). Furthermore, the signals for hydrocarbons (e.g., m/z55 and 57) and high molecular weight ions (m/z >100) for O1A0 (
= 1) are much more intense than that for A1A0 (
= 1). Taking the theoretical consideration (Section 2.3) and KOW–S relationship () into account, these results experimentally demonstrate the following: (Equation1
[1] ) both highly oxygenated and levoglucosan-like species are highly soluble in water, and (Equation2
[2] ) hydrocarbon-like and high molecular weight compounds are less soluble, although they could be a part of WSOM. These findings are also supported by comparing A1A0 and O1A0 at different values of
(
= 0.01 and 0.1). The intensities of m/z44 for A1A0 decrease for lower values of
, indicating that highly oxygenated compounds predominantly exist in highly soluble fraction. In fact, m/z44 signals for the less soluble fractions (O1A0 [
= 0.01 and 0.1]) are as small as “hydrocarbon-like organic aerosol (HOA)” fractions retrieved by the Positive Matrix Factorization (PMF) analysis of atmospheric organic aerosol particles (Ng et al. Citation2011). Similarly, fractions of high molecular weight ions (m/z > 100) are consistently larger for less soluble fractions.
Figure 7. Mass spectra of WSOM measured by the ToF-ACSM following classification by 1-octanol-water partitioning (single extraction). (a) Aqueous phase (A1A0, = 1), (b) 1-octanol phase (O1A0,
= 1), (c) aqueous phase (A1A0,
= 0.1), (d) 1-octanol phase (O1A0,
= 0.1), (e) aqueous phase (A1A0,
= 0.01), and (f) 1-octanol phase (O1A0,
= 0.01).
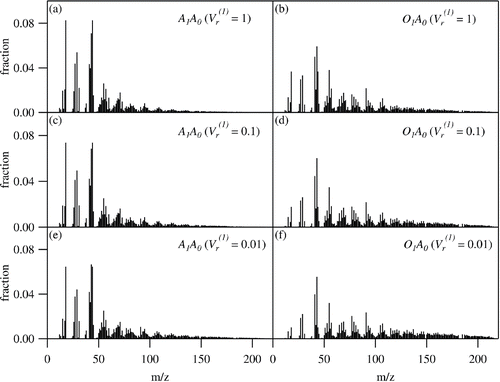
4.3. Fractions of WSOM partitioned to 1-octanol and aqueous phases
Mass fractions of WSOM partitioned to the 1-octanol and aqueous phases were estimated, combining the mass spectra of A0, A1A0, and O1A0. The estimation was performed by numerically minimizing the following metric:[13] where
denotes the normalized signal intensity (i.e., fraction) of ions at m/z = i observed for Xn solution and a is the parameter to be optimized (0 ≤ a ≤ 1) (Figure S4). The mass fraction of organic materials partitioned to the 1-octanol phase corresponds to a, while that for the aqueous phase is equivalent as (1 − a). The numerical calculation successfully reconstructed the original mass spectrum of A0, confirming the validity of the approach (Figure S5). A merit of this experimental approach is that absolute concentrations of WSOM in the aqueous and 1-octanol phases do not affect reconstruction of the mass spectra as long as mass spectra of these species are accurately quantified.
shows the estimated fractions of organic materials partitioned to the 1-octanol and aqueous phases. The optimized values of a are 0.16 ( = 0.01), 0.33 (
= 0.1), and 0.55 (
= 1). In general, the fractions of organic materials partitioned to the 1-octanol phase increase with
, which is in good agreement with the theoretical prediction (). The obtained value of a (
= 0. 01) indicates that solubility of approximately 84% of extracted WSOM could be higher than 10−4 (g cm−3), potentially contributing to CCN activation (; Petters and Kreidenweis Citation2008; Kuwata et al. Citation2013). It should be noted that particle size also needs to be considered for CCN activation in the atmosphere (Dusek et al. Citation2006). Furthermore, the fraction of WSOM in the highly soluble range for CCN activation (S > 10−1 [g cm−3]) is estimated to be around 45% on the basis of the values of a (
= 1) and the theoretical evaluation for solubility classification (; Petters and Kreidenweis Citation2008; Kuwata et al. Citation2013).
Figure 8. Mass fractions of WSOM partitioned to 1-octanol and aqueous phases at = 0.01, 0.1, and 1. These values are estimated from the ACSM mass spectra. See the text for details.
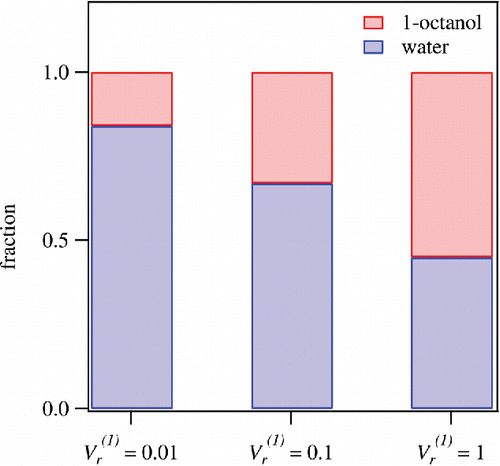
The majority of the uncertainty in estimating the solubility distribution using this experimental approach is likely associated with the empirical correlation between KOW and S. Considering the very good agreement (slope = 1.007, r2 = 0.999) between the observed and reconstructed WSOM mass spectra (Figure S5), which occurs only at a certain value of a, (Figure S4), there would not be a significant error in estimating fractions of compounds those partitioned to aqueous and 1-octanol phases. The good correlation also supports that there was not a significant artifact associated with separation and measurement processes. On the other hand, the empirical relationship between KOW and S has an uncertainty of Δlog (S) = ±0.39. The uncertainty associated with the nature of the empirical correlation should be considered for quantitative interpretation of the data.
The promising result from the mosquito coil burning experiment encourages further verification and development of the method. For instance, quantitative experiments using atmospheric relevant pure species will help validating the method in more detail. A systematic test of multiple extraction approaches, which was indicated to be successful for our preliminary tests (not shown), will enhance our capability to classify WSOM by the 1-octanol-water partitioning method. Development of an appropriate data inversion algorithm, which employs the theoretical curves as kernel functions ( and ), will be helpful to estimate solubility distribution from experimental data. Experimentally determined solubility distribution by such a method will be eventually useful for predicting the CCN activity of aerosol particles (Riipinen et al. Citation2015).
5. Conclusions
A classification method for water-soluble organic matters (WSOMs), which employs 1-octanol-water partitioning, was developed. The partitioning coefficients of organic compounds between 1-octanol and water are known to correlate with their water solubility. A theoretical analysis of the method demonstrated that the solubility of organic compounds partitioning to the aqueous phase tends to be higher when a volume ratio of 1-octanol to water () is large. In addition, the theoretical analysis showed that classification resolution by the partitioning method is improved by multiple extraction approaches.
The developed concept was evaluated using organic aerosol particles generated by smoldering of a mosquito coil. Following sample collection, WSOM in the collected samples were extracted. A mass spectrum of WSOM was recorded using the Time-of-Flight Aerosol Chemical Speciation Monitor (ToF-ACSM). In addition, WSOM was classified by the 1-octanol-water partitioning method at = 0.01, 0.1, and 1. The mass spectra of WSOM partitioned to both the 1-octanol and aqueous phases were measured using the ToF-ACSM. WSOM partitioned to aqueous phases were highly oxygenated, whereas those for 1-octanol phases contained significant fractions of hydrocarbon peaks. In fact, the fraction of m/z44 for WSOM partitioned to a 1-octanol phase (
= 0.01) was as insignificant as that of hydrocarbon-like organic aerosol (HOA).
The reconstruction of the WSOM mass spectrum allowed the estimation of the fractions of materials partitioned to them. The analysis indicated that the major fraction of WSOM was sufficiently soluble for contributing to cloud condensation nuclei (CCN) activation. The promising result from the mosquito coil burning experiment encourages conducting detailed verification experiment using standard chemicals in the future. In addition, future development of an appropriate data inversion algorithm will be helpful in establishing an accurate estimation method of solubility distribution of WSOM, which is important for predicting the CCN activity of aerosol particles.
UAST_1283004_Supplementary_File.zip
Download Zip (196.2 KB)Acknowledgments
The authors acknowledge Jing Chen for useful discussions and assistance in the experiment.
Funding
This research was supported by the National Research Foundation Singapore under its Singapore NRF Fellowship scheme (National Research Fellow Award, NRF2012NRF-NRFF001-031), the Earth Observatory of Singapore (EOS), and Nanyang Technological University.
References
- Allan, J. D., Delia, A. E., Coe, H., Bower, K. N., Alfarra, M. R., Jimenez, J. L., Middlebrook, A. M., Drewnick, F., Onasch, T. B., Canagaratna, M. R., Jayne, J. T., and Worsnop, D. R. (2004). A Generalised Method for the Extraction of Chemically Resolved Mass Spectra from Aerodyne Aerosol Mass Spectrometer Data. J. Aerosol Sci., 35:909–922.
- Asa-Awuku, A., Sullivan, A. P., Hennigan, C. J., Weber, R. J., and Nenes, A. (2008). Investigation of Molar Volume and Surfactant Characteristics of Water-Soluble Organic Compounds in Biomass Burning Aerosol. Atmos. Chem. Phys., 8:799–812.
- Carslaw, K. S., Boucher, O., Spracklen, D. V., Mann, G. W., Rae, J. G. L., Woodward, S., and Kulmala, M. (2010). A Review of Natural Aerosol Interactions and Feedbacks Within the Earth System. Atmos. Chem. Phys., 10:1701–1737.
- Chiou, C. T., Schmedding, D. W., and Manes, M. (1982). Partitioning of Organic Compounds in Octanol-Water Systems. Environ. Sci. Technol., 16:4–10.
- Coates, M., Connell, D. W., and Barron, D. M. (1985). Aqueous Solubility and Octan-1-ol to Water Partition-Coefficients of Aliphatic-Hydrocarbons. Environ. Sci. Technol., 19:628–632.
- Cubison, M. J., Ortega, A. M., Hayes, P. L., Farmer, D. K., Day, D., Lechner, M. J., Brune, W. H., Apel, E., Diskin, G. S., Fisher, J. A., Fuelberg, H. E., Hecobian, A., Knapp, D. J., Mikoviny, T., Riemer, D., Sachse, G. W., Sessions, W., Weber, R. J., Weinheimer, A. J., Wisthaler, A., and Jimenez, J. L. (2011). Effects of Aging on Organic Aerosol from Open Biomass Burning Smoke in Aircraft and Laboratory Studies. Atmos. Chem. Phys., 11:12049–12064.
- Decesari, S., Facchini, M. C., Fuzzi, S., and Tagliavini, E. (2000). Characterization of Water-Soluble Organic Compounds in Atmospheric Aerosol: A New Approach. J. Geophys. Res., 105:1481–1489.
- Duarte, R., and Duarte, A. C. (2005). Application of Non-Ionic Solid Sorbents (XAD resins) for the Isolation and Fractionation of Water-Soluble Organic Compounds from Atmospheric Aerosols. J. Atmos. Chem., 51:79–93.
- Dusek, U., Frank, G. P., Hildebrandt, L., Curtius, J., Schneider, J., Walter, S., Chand, D., Drewnick, F., Hings, S., Jung, D., Borrmann, S., and Andreae, M. O. (2006). Size Matters More than Chemistry for Cloud-Nucleating Ability of Aerosol Particles. Science, 312:1375–1378.
- Fan, X. J., Song, J. Z., and Peng, P. A. (2013). Comparative Study for Separation of Atmospheric Humic-Like Substance (HULIS) by ENVI-18, HLB, XAD-8 and DEAE Sorbents: Elemental Composition, FT-IR, H-1 NMR and Off-Line Thermochemolysis with Tetramethylammonium Hydroxide (TMAH). Chemosphere, 93:1710–1719.
- Farmer, D. K., Cappa, C. D., and Kreidenweis, S. M. (2015). Atmospheric Processes and their Controlling Influence on Cloud Condensation Nuclei Activity. Chem. Rev., 115:4199–4217.
- Fiore, A. M., Naik, V., Spracklen, D. V., Steiner, A., Unger, N., Prather, M., Bergmann, D., Cameron-Smith, P. J., Cionni, I., Collins, W. J., Dalsoren, S., Eyring, V., Folberth, G. A., Ginoux, P., Horowitz, L. W., Josse, B., Lamarque, J.-F., MacKenzie, I. A., Nagashima, T., O'Connor, F. M., Righi, M., Rumbold, S. T., Shindell, D. T., Skeie, R. B., Sudo, K., Szopa, S., Takemura, T., and Zeng, G. (2012). Global Air Quality and Climate. Chem. Soc. Rev., 41:6663–6683.
- Froehlich, R., Cubison, M. J., Slowik, J. G., Bukowiecki, N., Prevot, A. S. H., Baltensperger, U., Schneider, J., Kimmel, J. R., Gonin, M., Rohner, U., Worsnop, D. R., and Jayne, J. T. (2013). The ToF-ACSM: A Portable Aerosol Chemical Speciation Monitor with TOFMS Detection. Atmos. Meas. Tech., 6:3225–3241.
- Graber, E. R., and Rudich, Y. (2006). Atmospheric HULIS: How Humic-Like are they? A Comprehensive and Critical Review. Atmos. Chem. Phys., 6:729–753.
- Graham, B., Mayol-Bracero, O. L., Guyon, P., Roberts, G. C., Decesari, S., Facchini, M. C., Artaxo, P., Maenhaut, W., Koll, P., and Andreae, M. O. (2002). Water-Soluble Organic Compounds in Biomass Burning Aerosols Over Amazonia-1. Characterization by NMR and GC-MS. J. Geophys. Res., 107:8047.
- Hallquist, M., Wenger, J. C., Baltensperger, U., Rudich, Y., Simpson, D., Claeys, M., Dommen, J., Donahue, N. M., George, C., Goldstein, A. H., Hamilton, J. F., Herrmann, H., Hoffmann, T., Iinuma, Y., Jang, M., Jenkin, M. E., Jimenez, J. L., Kiendler-Scharr, A., Maenhaut, W., McFiggans, G., Mentel, T. F., Monod, A., Prevot, A. S. H., Seinfeld, J. H., Surratt, J. D., Szmigielski, R., and Wildt, J. (2009). The Formation, Properties and Impact of Secondary Organic Aerosol: Current and Emerging Issues. Atmos. Chem. Phys., 9:5155–5236.
- Hamilton, J. F., Webb, P. J., Lewis, A. C., Hopkins, J. R., Smith, S., and Davy, P. (2004). Partially Oxidised Organic Components in Urban Aerosol Using GCXGC-TOF/MS. Atmos. Chem. Phys., 4:1279–1290.
- Hansch, C. H., Leo, A., and Hoekman, D. H. (1995). Exploring QSAR: Hydrophobic, Electronic, and Steric Constraints. ACS Publications, Washington, DC.
- Hartz, K. E. H., Tischuk, J. E., Chan, M. N., Chan, C. K., Donahue, N. M., and Pandis, S. N. (2006). Cloud Condensation Nuclei Activation of Limited Solubility Organic Aerosol. Atmos. Environ., 40:605–617.
- Hoffer, A., Gelencser, A., Guyon, P., Kiss, G., Schmid, O., Frank, G. P., Artaxo, P., and Andreae, M. O. (2006). Optical Properties of Humic-Like Substances (HULIS) in Biomass-Burning Aerosols. Atmos. Chem. Phys., 6:3563–3570.
- Isnard, P., and Lambert, S. (1989). Aqueous Solubility and N-Octanol Water Partition-Coefficient Correlations. Chemosphere, 18:1837–1853.
- Jimenez, J. L., Canagaratna, M. R., Donahue, N. M., Prevot, A. S. H., Zhang, Q., Kroll, J. H., DeCarlo, P. F., Allan, J. D., Coe, H., Ng, N. L., Aiken, A. C., Docherty, K. S., Ulbrich, I. M., Grieshop, A. P., Robinson, A. L., Duplissy, J., Smith, J. D., Wilson, K. R., Lanz, V. A., Hueglin, C., Sun, Y. L., Tian, J., Laaksonen, A., Raatikainen, T., Rautiainen, J., Vaattovaara, P., Ehn, M., Kulmala, M., Tomlinson, J. M., Collins, D. R., Cubison, M. J., Dunlea, E. J., Huffman, J. A., Onasch, T. B., Alfarra, M. R., Williams, P. I., Bower, K., Kondo, Y., Schneider, J., Drewnick, F., Borrmann, S., Weimer, S., Demerjian, K., Salcedo, D., Cottrell, L., Griffin, R., Takami, A., Miyoshi, T., Hatakeyama, S., Shimono, A., Sun, J. Y., Zhang, Y. M., Dzepina, K., Kimmel, J. R., Sueper, D., Jayne, J. T., Herndon, S. C., Trimborn, A. M., Williams, L. R., Wood, E. C., Middlebrook, A. M., Kolb, C. E., Baltensperger, U., and Worsnop, D. R. (2009). Evolution of Organic Aerosols in the Atmosphere. Science, 326:1525–1529.
- Kenaga, E. E., and Goring, C. A. (1980). Relationship Between Water Solubility, Soil Sorption, Octanol-Water Partitioning, and Concentration of Chemicals in Biota. in Aquatic Toxicology Proceedings of the Third Annual Symposium on Aquatic Toxicology, J. G. Eaton, P. R. Parrish, and A. C. Hendriclcs, eds., American Society for Testing and Materials, Baltimore, MD, pp. 78–115.
- Kuwata, M., Shao, W., Lebouteiller, R., and Martin, S. T. (2013). Classifying Organic Materials by Oxygen-To-Carbon Elemental Ratio to Predict the Activation Regime of Cloud Condensation Nuclei (CCN). Atmos. Chem. Phys., 13:5309–5324.
- Laumbach, R. J., and Kipen, H. M. (2012). Respiratory Health Effects of Air Pollution: Update on Biomass Smoke and Traffic Pollution. J. Allergy Clin. Immunol., 129:3–13.
- Li, Y. J., Yeung, J. W. T., Leung, T. P. I., Lau, A. P. S., and Chan, C. K. (2012). Characterization of Organic Particles from Incense Burning Using an Aerodyne High-Resolution Time-of-Flight Aerosol Mass Spectrometer. Aerosol Sci. Technol., 46:654–665.
- Londahl, J., Pagels, J., Boman, C., Swietlicki, E., Massling, A., Rissler, J., Blomberg, A., Bohgard, M., and Sandstrom, T. (2008). Deposition of Biomass Combustion Aerosol Particles in the Human Respiratory Tract. Inhal. Toxicol., 20:923–933.
- Meylan, W. M. and Howard, P. H. (1995). Atom Fragment Contribution Method for Estimating Octanol-Water Partition-Coefficients. J. Pharm. Sci., 84:83–92.
- Meylan, W. M., Howard, P. H., and Boethling, R. S. (1996). Improved Method for Estimating Water Solubility from Octanol Water Partition Coefficient. Environ. Toxicol. Chem., 15:100–106.
- Mihara, T., and Mochida, M. (2011). Characterization of Solvent-Extractable Organics in Urban Aerosols Based on Mass Spectrum Analysis and Hygroscopic Growth Measurement. Environ. Sci. Technol., 45:9168–9174.
- Mochida, M., and Kawamura, K. (2004). Hygroscopic Properties of Levoglucosan and Related Organic Compounds Characteristic to Biomass Burning Aerosol Particles. J. Geophys. Res., 109:D21202.
- Ng, N. L., Canagaratna, M. R., Jimenez, J. L., Chhabra, P. S., Seinfeld, J. H., and Worsnop, D. R. (2011). Changes in Organic Aerosol Composition with Aging Inferred from Aerosol Mass Spectra. Atmos. Chem. Phys., 11:6465–6474.
- Petters, M. D., and Kreidenweis, S. M. (2008). A Single Parameter Representation of Hygroscopic Growth and Cloud Condensation Nucleus Activity-Part 2: Including Solubility. Atmos. Chem. Phys., 8:6273–6279.
- Polidori, A., Turpin, B. J., Davidson, C. I., Rodenburg, L. A., and Maimone, F. (2008). Organic PM2.5: Fractionation by Polarity, FTIR Spectroscopy, and OM/OC Ratio for the Pittsburgh Aerosol. Aerosol Sci. Technol., 42:233–246.
- Prenni, A. J., DeMott, P. J., Kreidenweis, S. M., Sherman, D. E., Russell, L. M., and Ming, Y. (2001). The Effects of Low Molecular Weight Dicarboxylic Acids on Cloud Formation. J. Phys. Chem. A, 105:11240–11248.
- Psichoudaki, M., and Pandis, S. N. (2013). Atmospheric Aerosol Water-Soluble Organic Carbon Measurement: A Theoretical Analysis. Environ. Sci. Technol., 47:9791–9798.
- Ramanathan, V., Crutzen, P. J., Kiehl, J. T., and Rosenfeld, D. (2001). Atmosphere-Aerosols, Climate, and the Hydrological Cycle. Science, 294:2119–2124.
- Riipinen, I., Rastak, N., and Pandis, S. N. (2015). Connecting the Solubility and CCN Activation of Complex Organic Aerosols: A Theoretical Study Using Solubility Distributions. Atmos. Chem. Phys., 15:6305–6322.
- Rosenorn, T., Kiss, G., and Bilde, M. (2006). Cloud Droplet Activation of Saccharides and Levoglucosan Particles. Atmos. Environ., 40:1794–1802.
- Sangster, J. (1989). Octanol-Water Partition-Coefficients of Simple Organic-Compounds. J. Phys. Chem. Ref. Data, 18:1111–1229.
- Shulman, M. L., Jacobson, M. C., Carlson, R. J., Synovec, R. E., and Young, T. E. (1996). Dissolution Behavior and Surface Tension Effects of Organic Compounds in Nucleating Cloud Droplets. Geophys. Res. Lett., 23:277–280.
- Sullivan, A. P., and Weber, R. J. (2006). Chemical Characterization of the Ambient Organic Aerosol Soluble in Water: 1. Isolation of Hydrophobic and Hydrophilic Fractions with a XAD-8 Resin. J. Geophys. Res., 111:D05314.
- Sullivan, R. C., Moore, M. J. K., Petters, M. D., Kreidenweis, S. M., Roberts, G. C., and Prather, K. A. (2009). Effect of Chemical Mixing State on the Hygroscopicity and Cloud Nucleation Properties of Calcium Mineral Dust Particles. Atmos. Chem. Phys., 9:3303–3316.
- Valvani, S. C., Yalkowsky, S. H., and Roseman, T. J. (1981). Solubility and Partitioning IV: Aqueous Solubility and Octanol-Water Partition-Coefficients of Liquid Non-Electrolytes. J. Pharm. Sci., 70:502–507.
- Wang, J., Jacob, D. J., and Martin, S. T. (2008). Sensitivity of Sulfate Direct Climate Forcing to the Hysteresis of Particle Phase Transitions. J. Geophys. Res., 113:D11207.
- Weber, R. J., Orsini, D., Daun, Y., Lee, Y. N., Klotz, P. J., and Brechtel, F. (2001). A Particle-Into-Liquid Collector for Rapid Measurement of Aerosol Bulk Chemical Composition. Aerosol Sci. Technol., 35:718–727.
- Wu, G.-M., Cong, Z.-Y., Kang, S.-C., Kawamura, K., Fu, P.-Q., Zhang, Y.-L., Wana, X., Gao, S.-P., and Liu, B. (2016). Brown Carbon in the Cryosphere: Current Knowledge and Perspective. Advan. Climate. Change. Res., 7:82–89.
- Yalkowsky, S. H., and Dannenfelser, R. M. (1992). AQUASOL Database of Aqueous Solubility, version 5, in College of Pharmacy. University of Arizona, Tucson, AZ, USA.
- Yalkowsky, S. H., and He, Y. (2003). Handbook of Aqueous Solubilities. CRC Press, Boca Raton, FL.
- Yu, H., Kaufman, Y. J., Chin, M., Feingold, G., Remer, L. A., Anderson, T. L., Balkanski, Y., Bellouin, N., Boucher, O., Christopher, S., DeCola, P., Kahn, R., Koch, D., Loeb, N., Reddy, M. S., Schulz, M., Takemura, T., and Zhou, M. (2006). A Review of Measurement-Based Assessments of the Aerosol Direct Radiative Effect and Forcing. Atmos. Chem. Phys., 6:613–666.
- Yu, J. Z., Cocker, D. R., Griffin, R. J., Flagan, R. C., and Seinfeld, J. H. (1999). Gas-Phase Ozone Oxidation of Monoterpenes: Gaseous and Particulate Products. J. Atmos. Chem., 34:207–258.
Appendix:
Comparison with solid phase extraction
Classification of WSOM in atmospheric aerosol particles has been conducted using solid phase extraction technique (Duarte and Duarte Citation2005; Sullivan and Weber Citation2006). Comparison of the existing technique with 1-octanol-water extraction method, which is newly introduced in the present study, is beneficial in comparing outputs from different techniques. Sullivan and Weber (Citation2006) tested penetration efficiency of XAD-8 column using 37 compounds. Among these species, values of KOW and penetration efficiency of XAD-8 column were compared for 33 compounds. Experimentally determined values of KOW were employed when these data are available. Values of KOW estimated using KOWWIN v1.68 were also used for this comparison. All the data for the comparison are summarized in Table S2.
compares KOW with penetration ratios through XAD-8 column. All the compound with high penetration ratios (∼100%) have relatively low values of KOW (log KOW ≤ 0.59). On the other hand, the values of KOW for non-penetrating species are higher ((log KOW ≥ −0.48). The clear separation of penetration experiment data for XAD-8 by KOW demonstrates that classification of WSOM by 1-octanol-water partitioning is comparable to traditional solid phase extraction. An advantage of 1-octanol-water partitioning is that the flexibility of the method allows designing shapes and thresholds of WSOM classification.
Figure A1. Comparison of penetration ratio of organic compounds through XAD-8 column measured by Sullivan and Weber (Citation2006) with (a) experimentally determined KOW, and with (b) estimated values of KOW.
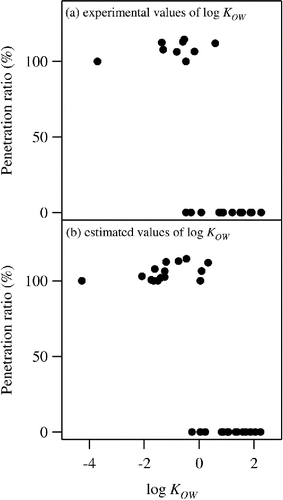