ABSTRACT
The increasing popularity of electronic cigarettes (e-cigarettes) and, more recently, the new “heat-not-burn” tobacco products (iQOS) as alternatives to traditional tobacco cigarettes has necessitated further documentation of and research into the composition and potential health risks/benefits of these devices. In a recent study, we compared second-hand exposure to particulate metals and organic compounds from e-cigarettes and traditional cigarettes, by conducting continuous and time-integrated measurements in an indoor environment, followed by computing the emission rates of these species using a single-compartment mass balance model. In this study, we have used a similar approach to further expand our previous analyses by characterizing black carbon, metal particles, organic compounds, and size-segregated particle mass and number concentrations emitted from these devices in addition to the newly marketed iQOS. Analysis of the iQOS side-stream smoke indicated that the particulate emission of organic matter from these devices is significantly different depending on the organic compound. While polycyclic aromatic hydrocarbons (PAHs) were mostly non-detectable in the iQOS smoke, certain n-alkanes, organic acids (such as suberic acid, azelaic acid, and n-alkanoic acids with carbon numbers between 10 and 19) as well as levoglucosan were still emitted in substantial levels from iQOS (up to 2–6 mg/h during a regular smoking regimen). Metal emissions were reduced in iQOS smoke compared to both electronic cigarettes and conventional cigarettes and were mostly similar to the background levels. Another important finding is the presence of carcinogenic aldehyde compounds, including formaldehyde, acetaldehyde, and acrolein, in iQOS smoke, although the levels were substantially lower compared to conventional cigarettes.
Copyright © 2017 American Association for Aerosol Research
EDITOR:
1. Introduction
Tobacco consumption has been consistently declared as the leading preventable cause of morbidity and mortality in the world (Feigin et al. Citation2016). Numerous past and recent studies have documented the adverse health effects of environmental tobacco smoke (ETS; Castro et al. Citation2011; Wu et al. Citation2012; McCarville et al. Citation2013; Feleszko et al. Citation2014). Following the enhancement of regulatory measures on tobacco consumptions in public spaces, the tobacco industry initiated major investments in the development of new products, in an effort to revive the business by promoting and marketing new (often unregulated) products for the consumers (Savell et al. Citation2014).
These efforts were often disguised in products such as “light” cigarettes, despite the fact that many medical scientists since 1970s have consistently advised against promoting such products as “healthy” alternatives for smokers (TCPGT Panel report 2008). Accordingly, more than 12 years after promotion and wide use of “light” cigarettes, scientific investigations concluded that these products in fact pose an even higher health risk compared to higher tar cigarettes (Harris et al. Citation2004; Rigotti and Tindle Citation2004). This illustrates the importance of scientific investigation and public health scrutiny before releasing new unregulated smoking devices for public consumption.
Electronic cigarettes (EC) are battery-powered devices that produce an aerosol, from a water-based solution, containing a mixture of nicotine, glycerin, propylene glycol, and flavoring chemicals, depending on the different commercial brands. Since ECs appeared in the market in 2006, there has been a steady growth in sales. The introduction of ECs was intended to reduce risks of smoking, but some health care organizations have been reluctant to encourage smokers to switch to ECs, citing lack of evidence of efficacy and safety (Wasowicz et al. Citation2015; Dinakar and O'Connor Citation2016; Franck et al. Citation2016). Most of the investigations concluded that while these products are still not completely harmless, they can be considered “safer” compared to conventional cigarettes (CC) and, if regulated and monitored, perhaps a valid and maybe easier method to quit addiction to conventional cigarettes, especially for smokers with a history of difficulties quitting smoking (Shahab et al. Citation2017). Despite these claims, scientific evidence in this regard is still limited, and more importantly, issues such as second-hand exposure to certain chemicals (e.g., nicotine, heavy metals) are still not investigated in sufficient depth. Moreover, it has been suggested that ECs may alter the epidemiological perceptions of smoking, and likely tempt adolescents into smoking addiction (Barrington-Trimis et al. Citation2016).
Recently, Philip Morris International (PMI) entered the EC market with the launch of “iQOS,” a “heat-not-burn” cigarette that has been described as a hybrid between traditional and electronic cigarettes, declaring that it is a reduced-risk product (Caputi Citation2016). The iQOS heat-not-burn cigarette looks very similar to a second-generation vaporizer, but is very different from modern electronic cigarettes in terms of functionality. The iQOS or Electrically Heated Cigarette Smoking System (EHCSS) is comprised of a small cigarette made of elements that include a tobacco plug, hollow acetate tube, polymer-film filter, cellulose-acetate mouthpiece filter, and outer and mouth-end papers. The iQOS is equipped with sophisticated electronics that heat specially prepared and blended tobacco. iQOS heats the tobacco just enough to release a flavorful nicotine-containing vapor but without burning the tobacco. A key difference between conventional cigarettes and iQOS is that the tobacco in a cigarette burns at temperatures higher than 600°C, generating smoke that contains harmful chemicals. In contrast, in the iQOS the tobacco is heated up to lower temperatures (below 350°C) in an effort to produce lower amounts of air toxics. To use the iQOS, a consumer inserts the tobacco stick into the iQOS holder. The iQOS holder is equipped with an electronically controlled heater that heats the tobacco stick. The consumer pushes a button to turn on the heater, and then draws on the tobacco stick. The iQOS heater has an over-heating protection mechanism to prevent excessive heating (and possible burning) of the tobacco.
Given the growing advertisement of these new products and lack of scientific evidence on their health effects and emission characteristics, we have compared the environmental pollution generated by ECs, iQOS, and Conventional Cigarettes (CC), in a standard indoor environment. Moreover, the emission factors are also calculated for several air pollutants including metals and organic compounds, to further elucidate the extent to which these devices release deleterious compounds into the atmosphere.
2. Methods
2.1. Sampling site description
Air samples were collected at the sitting room of a flat owned by habitual smokers (volume: 48 m3), furnished with typical home appliances (e.g., closets, tables, and chairs). During the experiments, the room was normally occupied by two to three people and equipped with real time analyzers (placed 2 m away from the smokers), samplers and three fans were always in operation during the smoking sessions and blowing in three different directions, two horizontally and one vertically, to assure homogeneity in the sampling environment and maximal mixing.
2.2. Real time measurements
Black Carbon (BC) was measured at two wavelengths (880 nm and 370 nm), using an Aethalometer (model AE31, Magee Scientific) and reported in nanograms per m3 (ng/m3). While the standard BC (measured at 880 nm) represents elemental carbon (i.e., “soot”), the light absorption associated with shorter wavelengths (i.e., the UV BC) provides insights on possible organic compounds present in the air sample. Typically, aerosols with a high content of light-absorbing organic compounds exhibit higher BC levels at UV wavelengths (i.e., 370 nm; Kirchstetter et al. Citation2004). PM number concentration was monitored using a Met One Instruments particle counter operating with two channels (dp > 1.0 µm and dp > 0.3 µm). Additionally, sub-micron particles (ranging from 10 to 1000 nm in size) were measured using a condensation particle counter (CPC model 3007, TSI Inc., Shoreview, MN, USA). Particle mass concentration (in µg/m3) was measured at three size ranges, namely PM1, PM2.5, and PM10, using a Met One PM mass monitor (model Aerocet 531). The instrument was gravimetrically pre-calibrated by parallel comparison with the model BAM-1020 (Met One Instruments Inc.) with US EPA equivalence certificate designation N.° EQPM-0798-122.
2.3. Time integrated chemical analyses
Trace elements, metals, and particle-phase organic compounds were measured by time-integrated collections of PM samples, followed by offline extraction and chemical analysis. Total Suspended Particles (TSPs) were collected on quartz and Teflon filters (2 µm pore size, Whatman International Ltd., Midlestone, UK) loaded on four Sioutas Personal Cascade Impactor Samplers (Sioutas™ PCIS, SKC Inc., Eighty Four, PA, USA; Misra et al. Citation2002) each operating at 10 l/min, outdoors and indoors. To measure the metals and trace element concentrations, Teflon filters were digested in an acid mixture (comprised of nitric acid, hydrochloric acid, and hydrogen peroxide), inside of a microwave-assisted Teflon-made digestion bomb (Milestone ETHOS+), and subsequently analyzed using a high-resolution magnetic sector inductively coupled plasma mass spectrometry (ICP-MS; Thermo-Finnigan Element 2), as described in Herner et al. (Citation2006). To measure the concentration of individual organic species (including but not limited to alkanes, polycyclic aromatic hydrocarbons, and organic acids), quartz filters were extracted in a 1: 1 solution of dichloromethane and acetone, using Soxhlets, followed by volume reduction using rotary evaporation under high purity nitrogen and derivatization of carboxylic acids with diazomethane. The substrates were then analyzed by gas chromatography mass spectrometry (GC-MS) method (GC-6980, quadruple MS-5973, Agilent Technologies). Further details regarding this analytical method can be found in Stone et al. (Citation2008). In addition to the particle phase measurements, gas-phase aldehydes were also collected on silica vials activated with DNPH and analyzed according to the EPA method TO-11A -1999 (method TO-11A; US-EPA 1999). It is important to note that concentrations/emission rates of particulate metals and organics from EC and CC were measured and calculated using the exact same methodology as described here, and reported in a previous study (Saffari et al. Citation2014). Therefore, the ICP-MS and GC-MS analyses in this study were performed only on the iQOS samples and results were compared with the data obtained previously for EC and CC.
2.4. Air exchange rate calculations
To measure the air change per hour (ACH) rate, after completion of each smoking session and the exit of last smoker from the room, the Aethalometer continued to operate and the concentrations of standard BC (measured at 880 nm wavelength), which is representative of a conservative and stable pollutant, were recorded for an additional 3 h. The total ACH (which includes the cumulative impact of ventilation, deposition, and pump operation) was then calculated according to the following equation:
where Cini is the initial concentration of BC (ng/m3), Cend is the final concentration of BC (ng/m3), t is the total time (h), and ACH is the air change per hour (h−1).
The mean ACH value based on these measurements was about 1.54 h−1 and was used in the following section for estimation of emission factors. An example of the BC decay curve during ACH tests is presented in . ACH measurements were done continuously throughout the experiments from Fall 2015 to Spring 2016, without any significant changes in the mean ACH values.
2.5. Smoking protocol and emission factor calculations
Three different types of cigarettes were used in these experiments, all consumed by volunteer smokers:
Electronic cigarettes (EC): model “Elips Serie C,” Tank System (Ovale Europe Srl), refilled with 16 mg/ml nicotine cartridges. Each EC vaping session lasted for about 2 to 3 h (one puff every minute for about 7 min, followed by 3 min hold) in order to accumulate sufficient PM on the sampler's filters. A total of 13 smoking (vaping) sessions were performed and results reported as the average of these 13 sessions.
Heat-not-burn cigarettes (iQOS): a total of 10 iQOS with menthol and 14 without menthol were tested. Each session lasted for about 3 h, during which the devices were consumed in cycles consisted of 7 min smoking, followed by a short 3 min pause to change the HeatStick. Total number of iQOS and number of puffs per iQOS were recorded during each smoking session. The iQOS holder was cleaned using the special brush supplied with the kit after about 10–15 HeatSticks were consumed. The EC and iQOS models used for the experiments were not equipped with heater voltage controls and were used under “default” conditions throughout the measurements.
Conventional Cigarettes (CC): During each smoking session, a total of nine conventional cigarettes were smoked in sequence, each for about 7 min, with 3 min pauses in between.
Emission factors (EF): for the measured air pollutants were calculated using the following equation from Repace et al. (Citation2002):
where EF is the emission factor of EC, iQOS, or conventional cigarettes in µg/h, Cave is the time-averaged indoor concentration during the smoking sessions (in µg/m3), nave is the average number of EC or iQOS or conventional cigarettes being smoked during the average unit smoking time (i.e., ts / ΔT, where ts is the total duration of one smoking session and ΔT is the unit smoking time), ACH is the air exchange rate in h−1, and V is the space volume in m3.
EC, iQOS, and conventional cigarettes present very different modes of smoking, not only due to the inherently different working mechanisms of each device, but also because of different individual smoking/vaping habits of the smokers, which can affect the aerosols generated by these devices (Spindle et al. Citation2016). For instance, EC and iQOS do not emit side-stream vapor/smoke between the puffs, while conventional cigarettes continuously emit tobacco smoke even between the puffs. Moreover, some smokers do not inhale deeply into the lungs and therefore we observed very different, and much higher, levels of environmental pollution compared with the pollution levels produced by smokers inhaling deeply. These differences introduce difficulties in comparing EC, iQOS, and CC emission factors. To account for these differences, we further standardized our method by defining the following modes of consumption for our experiments:
EC mode of vaping: one puff every minute for 7 min, followed by 3 min hold. This mode results in a ts / ΔT value of 0.7 in the above equation, which was applied to all EF calculations.
iQOS mode of smoking: average smoking time of about 7 min for each iQOS, followed by a short 3 min pause necessary to change the iQOS HeatStick. This procedure results in a ts / ΔT value of 0.324 applied to all iQOS EF calculations.
CC mode of smoking: average smoking time of about 7 min for each CC followed by a short 3 min pause. This mode results in a ts /ΔT value of 0.432 applied to all CC EF calculations.
3. Results and discussion
3.1. Air pollutant concentrations
3.1.1. Black carbon (BC)
Conventional cigarettes (CC) showed the highest level of BC concentrations, with means of 78 (27) and 2.3 (1.1) µg/m3 (SD) for the 370 nm UV and the 880 nm Standard BC, respectively, as presented in . BC levels associated with CC smoking sessions showed a rapid increase over the background with statistically significant difference (p < 0.05, based on the Mann–Whitney Rank Sum Test). 370 nm UV BC increase during the iQOS consumption sessions was less pronounced, but still measurable, with a statistically significant increase over the background (p < 0.05). Unlike iQOS and CC, BC concentrations during EC consumption did not indicate any difference compared to the background level, consistent with the results obtained in a previous study (Saffari et al. Citation2014). The BC levels during iQOS consumption were non-detectable for 880 nm Standard BC and within 2.81–3.89% of the levels associated with CC, for 370 nm UV BC, indicating overall negligible levels of soot and light absorbing organics compared to CC. The background concentration was measured immediately before each session using the same instrument. At the end of each session, the ACH was calculated based on the decay rate of BC for about 3 h (without any occupants in the room). Following this, the background was again measured prior to the following smoking session for each specific pollutant. Examples of real-time BC concentration plots during EC, iQOS, and CC consumption are presented in . Moreover, average background-subtracted BC levels during the EC, iQOS, and CC smoking sessions are also presented in Figures S1, S2, and S3, respectively (see the online supplemental information [SI]).
Table 1. Environmental pollution associated with CC, EC, and iQOS. Numbers represent the arithmetic mean (standard deviation). All concentration values reported here are background-subtracted, thus reflecting the increase in indoor concentration during the smoking sessions compared to the background levels in the experiment room. “N.D.” represents non-detectable levels.
3.1.2. PM number concentration
Particle number was measured in three different size ranges, PM > 1.0 (i.e., particles larger than 1.0 µm), PM > 0.3 (i.e., particles larger than 0.3 µm), and PMnm (i.e., particles between 10 and 1000 nm). CC PM number concentrations during the smoking sessions were characterized by statistically significant (p < 0.05) peaks over the background, with means of 1012 (153) and 1.1 (0.79) particles per cm3 (SD) for the PM > 0.3 and PM > 1.0, respectively, as presented in and Figure S6 (see the SI). PM > 0.3 and PM > 1.0 number concentration during EC vaping were trivial compared to CC, showing slight increases over the background, without statistically significant difference (p > 0.05). It is important to note that the peaks clearly visible in the real time measurements, although small in magnitude, still imply the possible presence of PM generated during the vaping of the EC. IQOS-related PM > 0.3 and PM > 1.0 number concentrations were characterized by temporary, but very high peaks over the background, but were only statistically significant (i.e., p < 0.05) for PM > 0.3. Compared to the PM > 0.3 and PM > 1.0 size ranges, the increase in number concentration of nano-sized particles (PMnm) was remarkably more pronounced. PMnm showed a consistent increase compared to the background during both EC and iQOS consumption, although the increase was still lower compared to the PMnm elevation during CC smoking sessions (5.7%–7.0% and 22%–24% for EC and iQOS, respectively), as shown in . Real-time PM number concentration plots during iQOS, EC and CC consumption are presented in . Moreover, average background-subtracted number concentration levels during the EC, iQOS, and CC smoking sessions are also presented in Figures S4, S5, and S6, respectively (see the SI).
Figure 3. PM > 0.3, PM > 1.0 µm (top plot) and PMnm (i.e., between 10 and 1000 nm, bottom plot) number concentrations during EC vaping plotted as a function of time.
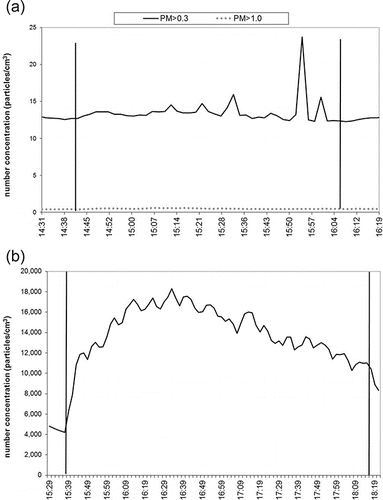
3.1.3. PM mass concentration
PM mass concentration during CC smoking was significantly higher than the background, with average levels of 151 (33), 500 (128), and 529 (127) µg/m3 (SD) for PM1, PM2.5, and PM10, respectively. All of these three PM size fractions also demonstrated consistent increase during the iQOS smoking sessions, although the level was substantially lower compared to CC (less than 2% of the CC, for all three size ranges). PM concentrations during EC vaping, unlike iQOS and CC, did not show a statistically significant increase over the background (p > 0.05), implying that EC emissions are mostly partitioned in the vapor phase. These results are summarized in . Also, examples of real-time PM mass concentration plots during iQOS, EC, and CC consumption are presented in Figures S7–S9 (see the SI).
3.1.4. Aldehydes
Concentrations of three aldehyde species, namely, acrolein, acetaldehyde, and formaldehyde were measured concurrently indoors and outdoors, and reported in . All of these species showed a statistically significant (p < 0.05) higher concentration indoors compared to outdoors during iQOS consumption, with their indoor concentrations being equivalent to 1.8%–2.3%, 5.0%–5.8%, and 6.9%–7.1% of the indoor concentration during CC smoking, for acrolein, acetaldehyde, and formaldehyde, respectively. During EC vaping, indoor concentration of acetaldehyde and formaldehyde showed a slight increase (significant at p < 0.05) compared to the outdoor concentrations, although these levels were still very small compared to that associated with CC smoking (less than 1%). Indoor concentration of acrolein did not show any significant increase during the EC consumption.
3.1.5. Metals and organic groups
Indoor and outdoor cumulative concentrations of metals/trace elements as well as organic groups, including PAHs, hopanes and steranes, n-alkanes, organic acids, and levoglucosan during iQOS smoking are presented in . Metals concentrations were, overall, higher during iQOS consumption with menthol, compared to when the device was in use without menthol, although there was no statistically significant difference between the indoor and outdoor levels for either of the two configurations. The mechanism behind higher metal emissions from menthol iQOS compared to iQOS without menthol is unclear and warrants further investigation. PAHs, hopanes, and steranes were all non-detected indoors, indicating that iQOS is not an emission source of these organic groups. n-Alkanes, organic acids, and levoglucosan, however, showed elevated concentrations indoors compared to outdoors during iQOS consumption (about two orders of magnitude higher for organic acids and n-alkanes, and 8–20 times higher for levoglucosan), with comparable levels for iQOS operating with and without menthol. Unlike iQOS, indoor concentrations during EC consumption were lower than or comparable to the outdoor levels (as shown in a previous study (Saffari et al. Citation2014), indicating that iQOS is, overall, more prone to releasing particulate organics compared to EC.
Figure 6. Outdoor and indoor concentrations of organic groups and metals during iQOS consumption. Similar data corresponding to EC and CC are reported in a previous study (Saffari et al. Citation2014). Error bars represent one standard deviation.
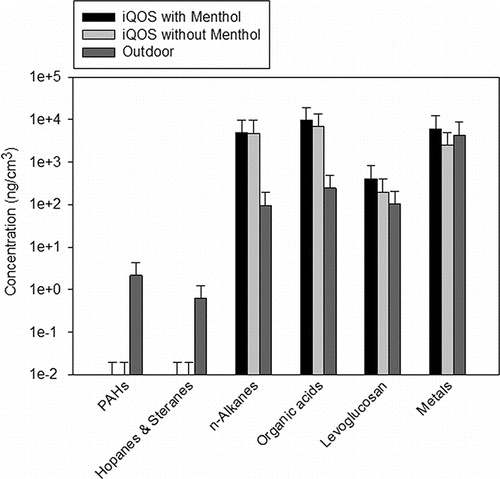
3.2. Pollutant emission factors
3.2.1. Black carbon (BC)
As presented in , 370 nm UV BC and 880 nm Standard BC were emitted during CC smoking with rates of 231,986(74,418) and 6045(2316) ng/min (SD), respectively. iQOS-associated emissions of BC were substantially less (less than 1% of CC for 370 nm UV BC and non-detectable for 880 nm Standard BC). BC was non-detectable in EC emissions. Our results indicate that while EC emissions are fairly clean in terms of particulate organic emissions, iQOS is still emitting, to some degree, light absorbing organic compounds. As discussed in Sections 3.1.6 and 3.2.6, these organic compounds are mostly comprised of n-alkanes and organic acids and unlike CC, the PAHs content of iQOS emissions is still negligible.
Table 2. Air pollutant emission factors of CC, EC, and iQOS. Numbers represent the mean value (standard deviation). “N.D.” represents not detectable levels.
3.2.2. PM number concentration
CC was a significant source of PM number concentration in all three measured size ranges, including PM > 0.3, PM > 1.0, and PMnm (i.e., between 10 nm and 1000 nm), with emission rates of 8.4E+08 (2.6E+08), 2.6E+06 (1.9E+06), and 1.3E+11 (7.7E+10) particles per min (SD), respectively. Particle number emissions from EC were non-detectable for PM > 0.3 and PM > 1.0, and ranged within 5.7%–8.0% of CC for PMnm. Similarly iQOS particle number emissions were non-detectable for PM > 1.0 and less than 1% of CC for PM > 0.3 size range, but were equivalent to 38%–41% of the CC for PMnm. It is noteworthy that one of the factors contributing to the EC PM number emissions observed in our measurements could be the possible release of un-evaporated e-liquid during the EC consumption.
3.2.3. PM mass concentration
CC smoking released 320.8(132.6), 1481.5(571.6), and 1535.9(570.6) µg/min (SD) for PM1, PM2.5, and PM10, respectively, as shown in . PM mass emission factors from both EC and iQOS were trivial, with values that were either non-detectable or equivalent to less than 2% of that for CC.
3.2.4. Aldehydes
Acrolein, acetaldehyde, and formaldehyde emission factors from CC were 6.5 (4.5), 101 (20), and 58 (9.1) µg/min (SD), respectively. iQOS emission of these compounds was substantially lower, equivalent to about 1.0%, 3.5%, and 4.5% of CC, respectively. Formaldehyde emission factor from EC was equivalent to about 1.9%–2.3% of CC, while emission factors of the other two measured compounds (i.e., acrolein and acetaldehyde) from EC were trivial compared to CC (less than 1%). Although these aldehydes are emitted from iQOS and EC at levels much lower than CC, this is still an alarming finding, given that they are widely recognized as carcinogens (Feron et al. Citation1991; Lindahl Citation1992). Presence of formaldehyde and acetaldehyde in EC emissions was also reported in a number of previous studies (Jensen et al. Citation2015; Gillman et al. Citation2016; Sleiman et al. Citation2016).
3.2.5. Metals and trace elements
Indoor emission factors of metals and trace elements are calculated for iQOS with and without menthol, and presented in . Moreover, the data are compared with emission factors of similar species from EC and CC, calculated using a similar set up in a previous study are shown in the table for comparison (Saffari et al. Citation2014). Sn was the only metal with considerable emission rates from both iQOS with menthol and without menthol (18,832 and 5668 ng/h, respectively). S, K, and Al also exhibited significant emissions (greater than 1 µg/h) from iQOS with menthol, while their emission was non-detectable for iQOS without menthol.
Table 3. Emission factors (ng/h) of selected metals and trace elements during iQOS, EC, and CC consumption. The data corresponding to EC and CC are adopted from Saffari et al. (Citation2014). Values correspond to average (±standard deviation). “N.D.” represents not detectable values.
3.2.6. Organic compounds
Emission factor of organic compounds detected in iQOS smoke are presented in , along with the data corresponding to EC and CC adopted from a previous study (Saffari et al. Citation2014). Overall, organic emissions from iQOS were substantially higher compared to EC, although they are still well below the emission factors associated with CC smoke. Hentriacontane and hectacosane were the most abundant n-alkanes in the iQOS smoke, with emission factors of 9236 and 6344 ng/h, respectively. Organic acids were more abundant in iQOS smoke compared to n-alkanes, with hexadecanoic acid and linoleic acid exhibiting the highest emission factors (11,453 and 11,137 ng/h, respectively). Unlike EC, levoglucosan (a strong tracer of biomass combustion, Simoneit et al. Citation1999) was detected in the smoke of iQOS, although the emission factor was still two orders of magnitude smaller than that for CC. It is important to note that the lower emission of PAHs and other organic compounds observed in this study is consistent with the results obtained in previous investigations. In an early study by McAuley et al. (Citation2012), the emission of carcinogenic organic compounds from first-generation vaporizers was reported to be substantially lower compared to tobacco cigarettes and well below the “cancer risk limit for both children and adults in an indoor environment.
Table 4. Emission factors (ng/h) of individual organic compounds during iQOS, EC, and CC consumption. The data corresponding to EC and CC are adopted from Saffari et al. (Citation2014). “N.D.” represents not detectable values.
4. Summary and conclusions
Overall, our results indicate that iQOS devices, while having substantially lower emissions of most toxic compounds compared to CC, are still not risk-free. iQOS emissions seem to be fairly clean in terms of metal emissions compared to both CC and EC. Detectable and statistically significant emissions of several organic compounds, including n-alkanes, organic acids, and aldehyde species such as formaldehyde, acetaldehyde, and acrolein from iQOS, though lower than those of CC, still warrant caution in unregulated use of these products, especially in public spaces where other people can be exposed passively to the second hand and side-stream smoke of these devices. We should note that, with the exception of aldehydes, all other organic data presented in this manuscript represent particle-phase organics. A wide range of organic emissions from EC and iQOS may likely be in the gas-phase, which was not measured here. This is clearly one of the limitations of our study and warrants future investigations. Moreover, it is important to note that all of the results presented in this manuscript pertain to one brand of iQOS/EC, and may not represent the possible variability among different brands and manufacturers.
Moreover, the data presented here correspond to the specific brands of EC and iQOS that were studied, and may not necessarily represent other brands/configurations of these devices. Considering the results of our studies as well as other studies reported in literature, it is our opinion that smoking/vaping in indoor public environments should be restricted.
Nomenclature
BC | = | black carbon |
CC | = | conventional cigarettes (Marlboro red) |
EC | = | electronic cigarettes (“Elips Serie C”, Tank System (Ovale Europe Srl) |
EF | = | emission factor |
iQOS | = | heat-not-burn tobacco cigarettes (PM iQOS) |
PM | = | particulate matter |
PM1 | = | particulate matter with aerodynamic diameter smaller than 1 µm |
PM2.5 | = | particulate matter with aerodynamic diameter smaller than 2.5 µm |
PM10 | = | particulate matter with aerodynamic diameter smaller than 10 µm |
PM > 1.0 | = | particulate matter with aerodynamic diameter larger than 1 µm |
PM > 0.3 | = | particulate matter with aerodynamic diameter larger than 0.3 µm |
PMnm | = | particulate matter with aerodynamic diameter between 10 and 1000 nm |
UAST_1300231_Supplementary_File.zip
Download Zip (448.4 KB)Acknowledgments
The authors thank Zhi Ning at the City University of Hong Kong for making available the instruments for the nanoparticle measurement. No conflict of interest is declared by any of the authors. The authors wish to dedicate this work in memoriam to Giovanni Invernizzi and Manel Nebot.
Funding
This study was supported by Fondazione IRCCS, Istituto Nazionale dei Tumori, Milan, Italy, and by the University of Southern California.
References
- Barrington-Trimis, J. L., Urman, R., Berhane, K., Unger, J. B., Cruz, T. B., Pentz, M. A., Samet, J. M., Leventhal, A. M., and McConnell, R. (2016). E-Cigarettes and Future Cigarette Use. Pediatrics, 138:e20160379. doi:10.1542/peds.2016-0379
- Caputi, T. L. (2016). Industry Watch: Heat-Not-Burn Tobacco Products Are About to Reach Their Boiling Point. Tob. Control, 053264. doi:10.1136/tobaccocontrol-2016-053264
- Castro, W., Perrino, C., Nath, A., Grunin, A., Habre, R., Moshier, E., Wolfson, M., Rohr, A., Godbold, J., Schachter, P. E. N., et al. (2011). Is A Smoke Free Home Really Smoke Free? Am. J. Respir. Crit. Care Med., 183:A4029.
- Dinakar, C., and O'Connor, G. T. (2016). The Health Effects of Electronic Cigarettes. N. Engl. J. Med., 375:1372–1381. doi:10.1056/NEJMra1502466
- Feigin, V. L., Roth, G. A., Naghavi, M., Parmar, P., Krishnamurthi, R., Chugh, S., Mensah, G. A., Norrving, B., Shiue, I., Ng, M., Estep, K., Cercy, K., Murray, C. J. L., and Forouzanfar, M. H. (2016). Global burden of Stroke and Risk Factors in 188 Countries, During 1990–2013: A Systematic Analysis for the Global Burden of Disease Study, (2013). Lancet Neurol., 15:913–924. doi:10.1016/S1474-4422(16)30073-4
- Feleszko, W., Ruszczyń ski, M., Jaworska, J., Strzelak, A., Zalewski, B. M., and Kulus, M. (2014). Environmental Tobacco Smoke Exposure and Risk of Allergic Sensitisation in Children: A Systematic Review and Meta-Analysis. Arch. Dis. Child., 99:985–992. doi:10.1136/archdischild-2013-305444
- Feron, V. J., Til, H. P., de Vrijer, F., Woutersen, R. A., Cassee, F. R., and van Bladeren, P. J. (1991). Aldehydes: Occurrence, Carcinogenic Potential, Mechanism of Action and Risk Assessment. Mutat. Res. Toxicol., 259:363–385. doi:10.1016/0165-1218(91)90128-9
- Franck, C., Filion, K. B., Kimmelman, J., Grad, R., and Eisenberg, M. J. (2016). Ethical Considerations of E-Cigarette Use for Tobacco Harm Reduction. Respir. Res., 17:53. doi:10.1186/s12931-016-0370-3
- Gillman, I. G., Kistler, K. A., Stewart, E. W., and Paolantonio, A. R. (2016). Effect of Variable Power Levels on the Yield of Total Aerosol Mass and Formation of Aldehydes in E-Cigarette Aerosols. Regul. Toxicol. Pharmacol., 75:58–65. doi:10.1016/j.yrtph.2015.12.019
- Harris, J. E., Thun, M. J., Mondul, A. M., and Calle, E. E. (2004). Cigarette Tar Yields in Relation to Mortality from Lung Cancer in the Cancer Prevention Study II Prospective Cohort, 1982-8. BMJ, 328:72. doi:10.1136/bmj.328.7431.72
- Herner, J. D., Green, P. G., and Kleeman, M. J. (2006). Measuring the Trace Elemental Composition of Size-Resolved Airborne Particles. Environ. Sci. Technol., 40:1925–1933. doi:10.1021/es052315q
- Jensen, R. P., Luo, W., Pankow, J. F., Strongin, R. M., and Peyton, D. H. (2015). Hidden Formaldehyde in E-Cigarette Aerosols. N. Engl. J. Med., 372:392–394. doi:10.1056/NEJMc1413069
- Kirchstetter, T. W., Novakov, T., and Hobbs, P. V. (2004). Evidence That the Spectral Dependence of Light Absorption by Aerosols is Affected by Organic Carbon. J. Geophys. Res. Atmos., 109:D21208. doi:10.1029/2004JD004999
- Lindahl, R. (1992). Aldehyde Dehydrogenases and Their Role in Carcinogenesis. Crit. Rev. Biochem. Mol. Biol., 27:283–335. doi:10.3109/10409239209082565
- McAuley, T. R., Hopke, P. K., Zhao, J., and Babaian, S. (2012). Comparison of the Effects of E-Cigarette Vapor and Cigarette Smoke on Indoor Air Quality. Inhal. Toxicol., 24:850–857. doi:10.3109/08958378.2012.724728
- McCarville, M., Sohn, M.-W., Oh, E., Weiss, K., and Gupta, R. (2013). Environmental Tobacco Smoke and Asthma Exacerbations and Severity: The Difference Between Measured and Reported Exposure. Arch. Dis. Child., 98:510–514. doi:10.1136/archdischild-2012-303109
- Misra, C., Singh, M., Shen, S., Sioutas, C., and Hall, P. M. (2002). Development and Evaluation of a Personal Cascade Impactor Sampler (PCIS). J. Aerosol Sci., 33:1027–1047. doi:10.1016/S0021-8502(02)00055-1
- Repace, J. L., Ott, W. R., and Klepeis, N. E. (2002). Indoor Air Pollution From Cigar Smoke, in Smoking and Tobacco Control Monograph, Chapter 5.
- Rigotti, N. A., and Tindle, H. A. (2004). The Fallacy of “Light” Cigarettes. BMJ, 328:E278–E279. doi:10.1136/bmj.328.7440.E278
- Saffari, A., Daher, N., Ruprecht, A., Marco, C. D., Pozzi, P., Boffi, R., Hamad, S. H., Shafer, M. M., Schauer, J. J., Westerdahl, D., and Sioutas, C. (2014). Particulate Metals and Organic Compounds from Electronic and Tobacco-Containing Cigarettes: Comparison of Emission Rates and Secondhand Exposure. Environ. Sci. Process. Impacts, 16:2259–2267. doi:10.1039/C4EM00415A
- Savell, E., Gilmore, A. B., and Fooks, G. (2014). How Does the Tobacco Industry Attempt to Influence Marketing Regulations? A Systematic Review. PLOS ONE, 9:e87389. doi:10.1371/journal.pone.0087389
- Shahab, L., Goniewicz, M. L., Blount, B. C., Brown, J., McNeill, A., Alwis, K. U., Feng, J., Wang, L., and West, R. (2017). Nicotine, Carcinogen, and Toxin Exposure in Long-Term E-Cigarette and Nicotine Replacement Therapy Users: A Cross-sectional Study. Ann. Intern. Med. doi:10.7326/M16-1107
- Simoneit, B. R., Schauer, J. J., Nolte, C. G., Oros, D. R., Elias, V. O., Fraser, M. P., Rogge, W. F., and Cass, G. R. (1999). Levoglucosan, A Tracer for Cellulose in Biomass Burning and Atmospheric Particles. Atmos. Environ., 33:173–182.
- Sleiman, M., Logue, J. M., Montesinos, V. N., Russell, M. L., Litter, M. I., Gundel, L. A., and Destaillats, H. (2016). Emissions from Electronic Cigarettes: Key Parameters Affecting the Release of Harmful Chemicals. Environ. Sci. Technol., 50:9644–9651. doi:10.1021/acs.est.6b01741
- Spindle, T., Hiler, M., Breland, A., Karaoghlanian, N., Shihadeh, A. L., and Eissenberg, T. (2016). The Influence of a Mouthpiece-Based Topography Measurement Device on Electronic Cigarette User's Plasma Nicotine Concentration, Heart Rate, and Subjective Effects Under Directed and Ad Libitum Use Conditions. Nicotine Tob. Res., ntw174.
- Stone, E. A., Snyder, D. C., Sheesley, R. J., Sullivan, A. P., Weber, R. J., and Schauer, J. J. (2008). Source Apportionment of Fine Organic Aerosol in Mexico City During the MILAGRO Experiment 2006. Atmos. Chem. Phys., 8:1249–1259. doi:10.5194/acp-8-1249-2008
- TCPGT Panel (2008). A Clinical Practice Guideline for Treating Tobacco Use and Dependence: 2008 Update. Am. J. Prev. Med., 35:158–176. doi:10.1016/j.amepre.2008.04.009
- US-EPA. (1999). Compendium of Methods for the Determination of Toxic Organic Compounds in Ambient Air Second Edition Compendium Method TO-11A.
- Wasowicz, A., Feleszko, W., and Goniewicz, M. L. (2015). E-Cigarette Use Among Children and Young People: The Need for Regulation. Expert Rev. Respir. Med., 9:507–509. doi:10.1586/17476348.2015.1077120
- Wu, T., Fallin, M. D., Shi, M., Ruczinski, I., Liang, K. Y., Hetmanski, J. B., Wang, H., Ingersoll, R. G., Huang, S., Ye, X., Wu-Chou, Y.-H., Chen, P. K., Jabs, E. W., Shi, B., Redett, R., Scott, A. F., Murray, J. C., Marazita, M. L., Munger, R. G., and Beaty, T. H. (2012). Evidence of Gene-Environment Interaction for the RUNX2 Gene and Environmental Tobacco Smoke in Controlling the Risk of Cleft Lip With/Without Cleft Palate. Birt. Defects Res. A. Clin. Mol. Teratol., 94:76–83. doi:10.1002/bdra.22885