ABSTRACT
The transfer function of TSI's widely used 3071 Differential Mobility Analyzer (DMA) widens drastically when the flow rate Q of sheath gas exceeds 30–40 lit/min, limiting its ability to resolve very small particles. This flow instability is unexpected at the prevailing relatively small Reynolds number (Re < 400). Here, we note that the rings holding the laminarization screens penetrate into the flow channel, generating unsteady vortices. A screen step exists not only on the outer screen region, but also on the inner screen region. Using a new step-free screen, no critical transition is observed up to the highest flow rate achieved of Q = 103 lit/min. The original DMA widens the flow cross-section in the mixing region where the aerosol joins the sheath gas. The flow deceleration then arising at small aerosol input flow rates introduces another source of transfer function broadening, which, however, has negative resolution effects only at Q > 60 lit/min. This feature is suppressed here by modifying a single inlet piece. Although the two flow improvements implemented increase the resolving power in the analysis of very small particles, a substantial non-ideality of unclear origin remains: the best resolving power R found with electrosprayed ions of the protein Immunoglobulin is R = 13.9 for the trimer (IgG)3, and 12 for the monomer, even at a sheath/aerosol flow ratio of 100.
© 2017 American Association for Aerosol Research
EDITOR:
1. Introduction
TSI Inc.'s 3071 DMA was the first Differential Mobility Analyzer (TSI Inc., Shoreview, MN, USA; Liu and Pui Citation1974; Knutson and Whitby Citation1975a) to become available commercially, and has played a leading role in the study of submicron aerosols. This instrument is no longer supported by the manufacturer, having been substituted by the similar 3080 L. Nonetheless, a peculiarity of the 3071 and subsequent TSI DMAs, is that they only operate correctly at relatively low flow rates Q of sheath gas (Q < 30 lit/min), at which the Reynolds number (Equation1(1) ) based on the outer and inner radii R2, R1, and the kinematic viscosity ν of the sheath gas is substantially smaller than the critical value for turbulent transition in a tube or an annulus:
(1)
Indeed, using Q = 30 lit/min, R1 = 0.937 cm; R2 = 1.961 cm and ν = 0.15 cm2/s, we find Re = 375. Because increasing Q would be beneficial to improve the resolving power with small particles, a previous attempt to establish the cause of this early transition was reported by Eichler et al. (Citation1998). They identified two problems. First, the outer ring supporting the laminarization screen penetrates into the flow region, creating a step, downstream from which the flow would tend to shed vortices. Although these structures may decay at sufficiently small Re, they could survive sufficiently downstream to reach beyond the inlet slit, causing a time-dependent flow that would on the average broaden the transfer function. The second problematic feature they noted was a widening of the flow cross-section in the region where the aerosol joins the sheath gas. At ratios qi/Q < 7% between the aerosol inlet flow and the sheath gas flow, this widening leads to a mean deceleration, which may destabilize the flow. Eichler et al. (Citation1998) modified slightly the inlet geometry to moderate these two undesirable effects. Following the insights offered by numerical calculations of Chen and Pui (Citation1997), Eichler et al. (Citation1998) also narrowed the width of the inlet slit. These changes improved the behavior at qi/Q ratios below 10%, but the performance continued to deteriorate for Q > 30 lit/min.
DMAs with resolving powers well in excess of 50 have been developed, but their ability to separate large particles is limited by their short length. There are in fact certain technical difficulties in achieving high concentricity of the two cylindrical electrodes, while at the same time obtaining a highly laminar entry flow. For this reason, we have reexamined TSI's 3071 DMA in a second attempt to improve its inlet characteristics.
A first finding was that the original screen introduces not only the step on the outer electrode identified and corrected by Eichler et al. (Citation1998), but also a step on the inner side. We therefore decided to remove both these screen steps as well as the flow-widening problem at the point of entry of the aerosol. Two entry pieces were changed: the laminarization screens, and the flow channel between the screen and the aerosol inlet slit, to be referred to as P (PTSI, PNew). PNew has an OD slightly larger than 2R2, while the axial length of the inlet slit is maintained at the same small value used by Eichler et al. (Citation1998). The new screen rings fit closely the diameters of the inner and outer electrodes, avoiding any steps.
2. Experimental
2.1. DMA modifications
The geometrical changes introduced here are best understood with reference to those previously implemented by Eichler in his inlet piece. The TSI and Eichler inlet pieces were sketched (without dimensions) in of Eichler et al. (Citation1998). The Eichler inlet piece is shown here in , from the original Figure in Appendix A1 (p. 72) of Eichler's Diplomarbeit Thesis (Eichler Citation1997). Its upstream ID of 1.48″ (unfaithfully marked 1.56″ in to indicate the only change in the present inlet over the Eichler inlet) was designed by Eichler to match the excessive ID of the original TSI screen ring and therefore avoid this outer step without changing TSI's screen. The Eichler flow channel following the inlet screen widened conically to 1.56″ at the aerosol inlet slit. This downstream diameter is slightly larger than the 1.54″ OD of the DMA column, and is maintained here to achieve a modest flow deceleration in the delicate aerosol entry region. This stabilizing feature was first implemented by G. Reischl. The diverging conical region in Eichler's inlet (going from 1.48″ to 1.56″) was a source of boundary layer instability and has been removed here by widening the ID of the outer laminarization screen ring. Accordingly, the new inlet piece has a cylindrical wetted surface starting and ending with a diameter of 1.56″. It is hence identical to the Eichler inlet piece, except that it is internally shaven in order to convert the Eichler cone into a cylinder with an ID of 1.56″.
Figure 1. Sketches of Eichler's (Citation1997) inlet piece (left) and the new laminarizing screen (right). The new inlet piece is identical to that of Eichler, except that the wetted diverging conical region going from 1.48″ (marked 1.56″ here but actually drawn at 1.48″) to 1.56″ is shaven into a 1.56″ diameter cylinder. All dimensions in inches (″).
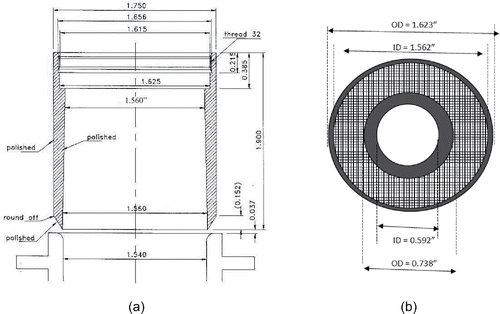
The new laminarizer rings are also sketched in . The outer ring fits tightly both in Eichler's and TSI's original inlet piece housing (1.625″ ID, 0.17″ height) having nominal outer and inner diameters of 1.623″ and 1.562″. These ring dimensions are designed to avoid outer steps in the flow channel following the screen in the new inlet piece (as well as in Eichler's inlet). They also avoid outer downstream steps in TSI's original inlet piece fitted with the new laminarizing structure. The diameters of the inner laminarization ring are 0.738″ and 0.592″. The laminarizer rings include two screen cloths. The upstream screen is 33% transparent, while transparencies of 50% and 33% were tested on the downstream screen.
2.2. Monodisperse aerosol
The sample aerosol was obtained by flowing 1 lit/min of dry and filtered air through a chamber where we electrosprayed a solution of the protein Immunoglobulin G (IgG) in water, to which 100 mM of triethylammonium formate were added to increase the electrical conductivity. After complete drying of the electrosprayed drops, the resulting multiply charged proteins were charge-reduced by passage through a region irradiated with 10 mCi of the low energy beta emitter Ni63. In separate experiments, this aerosol was analyzed with a halfmini DMA (Fernandez de la Mora and Kozlowski Citation2013), which showed that the full relative width at half maximum (FWHM) of the singly charged monomer IgG peak was less than 0.05. Defining the DMA resolution as R = 1/FWHM, this aerosol then permits inferring DMA resolution up to 20, considerably larger than the largest observed with either of the original or modified 3071 DMAs used. This peak width is relatively narrow with respect to what has been previously reported for IgG, due to a process of purification by dialysis (You et al. Citation2014), as well as by the favorable feature of the Ni-63 neutralizer of drying the drops prior to charge reduction (Fernandez de la Mora Citation2015). The dialyzed sample was kindly provided by Drs. Rian You and Michael Zachariah (NIST and University of Maryland).
2.3. DMA
Two different prototypes of TSI's 3071 DMA were used, one of which had an additional modification: The numerous holes on the sheath exit plane were bored to larger diameters to enable larger values of Q. Both DMAs were run in a fairly conventional closed circuit mode. Briefly, the excess air went through a flow meter and a flow control valve (both included in TSI's commercial instrument). The sheath gas then went to the inlet of a Gast diaphragm pump, whose outlet passed through a heat exchanger (to bring the recirculating gas near room temperature) and a HEPA filter into the sheath gas inlet. The closed circuit and the DMA were leak tight. In some early calibration experiments, the DMA circuit included TSI's original instrument flowmeter. This enabled measuring the classification voltage for the singly charged IgG monomer versus Q, yielding a ratio k = Q/VDMA= 1/1.75 lit/min/Volt for Q < 60 lit/min. The almost exactly constant value found for k gives confidence that TSI's 25 year-old flow calibration remains accurate. In subsequent experiments, the flowmeter was removed from the circuit to allow increased Q values up to 93 lit/min. The maximum Q achieved when removing entirely the control valve was slightly larger than 100 lit/min. In experiments without flowmeter Q was inferred from the formula Q = k VDMA. The DMA voltage was scanned automatically with an exponential ramp, such that each of the selected voltages differed by 1% from the prior voltage, with a dwell time of 2 s at each voltage. A high voltage power supply (Applied Kilovolts NPIN/0.8m, 0-700 Volt) different from the one attached to the commercial DMA was used in order to cover accurately the voltage range below 100 Volt.
2.4. Detector
The concentration of the monodisperse aerosol selected by the DMA was quantified with a condensation particle counter (TSI model 3760 CPC). The aerosol flow out of the DMA was kept at ambient pressure by introducing a T open to the laboratory on one of its three sides, immediately before the CPC inlet. The aerosol flow qi (∼1 lit/min) into the DMA was controlled to be slightly larger than that sampled by the CPC (∼0.8 lit/min), to avoid entry of ambient air through the T into the CPC. The aged CPC was operated at a sample flow rate smaller than its nominal 1.5 lit/min because it is no longer sensitive to small particles at the nominal flow.
3. Results
shows a series of spectra for the singly charged IgG monomer ion at flow rates increasing from right to left. Q was in these two cases determined through the peak voltage. is for the original 3071, and for the modified instrument including the step-free screen and the new inlet. The peak voltages VDMA, and relative widths FWHM for TSI's original DMA are collected in , showing a drastic deterioration of the peak shape beyond Q = 46 lit/min. Although the indirectly inferred value of Q is not reliable at the larger flow rates (corresponding to broad peaks), the figure illustrates qualitatively the well-known catastrophic loss of resolving power of this instrument at increased flow rate. The spectra in span the range 35 < Q (lit/min) < 94, with no sign of any loss of flow stability, with FWHM decreasing monotonically from 0.13 to 0.08. The original DMA model underperforms substantially even before FWHM catastrophically inverts its natural dependence on Q. For instance, at Q ∼ 30 lit/min, FWHM is 0.16 in the original and 0.118 in the modified configurations, justifying the manufacturer's recommendation of a flow rate of 20 lpm.
Figure 2. Flow rate dependence of the mobility spectrum of IgG. (a) TSI's original 3071 DMA. (b) Step-free screen with new inlet piece (including Gaussian fitting lines).
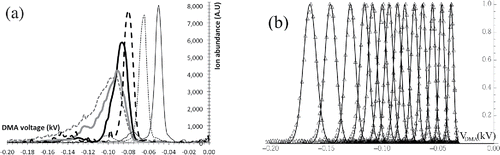
Table 1. Peak width dependence on sheath gas flow rate Q for two DMA configurations.
compares the peak widths for the two inlet pieces P (PNew, PTSI) when the DMA operates with the step-free screens. For reference, the figure includes predictions for pure Brownian diffusion, which, in the variables used, FWHM versus VDMA−1/2 is a straight line. The slope of 0.0192 kV1/2 used for that line is calculated for Poiseuille flow, based on the analysis of Rosell et al. (Citation1996), rather than earlier studies from Tammet (Citation1970) and Stolzenburg (Citation1988). The two inlet pieces have almost identical responses for Q < 60 lit/min. At larger flow rates, PTSI suffers a flow instability, which degrades the performance. The new inlet maintains a monotonic increase of resolution with increasing Q, though with a tendency to reach a plateau at FWHM ∼ 0.08 (∼0.07 for the dimer and trimer particles). While the slopes of both data sets are similar to the theoretical slope, both DMA configurations are substantially nonideal.
Figure 3. Effect of the inlet pieces (PNew, PTSI) on peak width for the singly charged IgG monomers (triangles), dimers (circles), and trimers (squares), with a step-free screen used in both panels. (a) PNew, data from the spectra of , including also dimer and trimer peak widths. (b) PTSI, with performance similar to that of PNew at moderate flow rates, but inferior at Q > 60 lit/min.
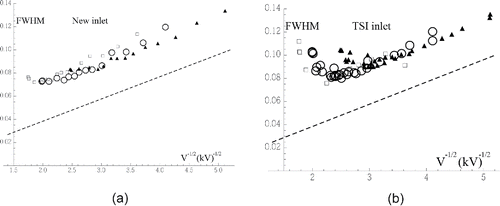
More direct confirmation of the notion that the loss of performance of TSI's inlet is due to a flow instability may be seen in , where the same resolution data are represented for the monomer dimer and trimer particles versus the flow rate Q, rather than peak voltage. For all three particles, the narrowest peak is obtained at 56 lit/min. The substantial reduction in peak width seen between the monomer and the dimer highlights the fact that a fair part of the peak width is due to Brownian diffusion.
Figure 4. Dependence of the peak width of the protein monomer (triangles), dimer (circles), and trimer particles (squares) on sheath gas flow rate Q for TSI inlet piece with the step-free laminarizer. Note a loss of performance above 56 lit/min.
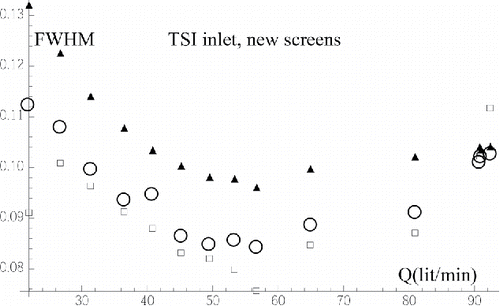
In an attempt to understand the source of non-ideal peak width seen in and , we have examined larger ions formed by aggregates containing from 2 to 4 protein molecules. includes one such spectrum, indicating the number n of monomers contained in the protein clusters associated with each of the peaks. The corresponding FWHM values are 0.072, 0.073, and 0.083 for n = 3, 2, 1, showing that the peak width is not limited just by geometrical or fluid flow imperfections of the DMA, but also by the sample aerosol used (monomer versus trimer). We have also tested several screens, some of them giving an advantage over others. For instance, the monomer peak width FWHM ∼ 0.083 seen in with a second screen 50% transparent, increased to ∼0.1 when the second screen was 33% transparent.
Figure 5. Mobility spectrum for electrosprayed and charge-reduced IgG in the doubly modified 3071 DMA. The peaks labeled n = 1–4 are for protein aggregates from the monomer to the tetramer. The small peaks on the left are for the doubly charged monomer and dimer. The dimer and trimer peaks are narrower than the monomer peak.
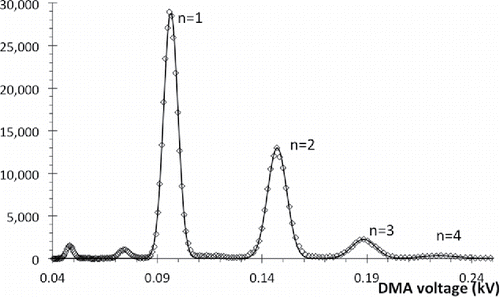
4. Discussion
There are several peak broadening routes that will need to be explored in the future in order to overcome the resolution limitation remaining with the new configuration. One possibility is that the protein peak itself is not as narrow as observed with the halfmini DMA (FWHM < 5%). We have previously seen that protein peaks are often narrower when the electrospray is sampled within the very short residence times available with a parallel plate DMA, suggesting that the protein is unstable and its cross-section naturally broadens within times of a few ms. Because TSI's long 3071 DMA has residence times of hundreds of ms, perhaps much of the peak width found is due to the aerosol rather than to geometric or flow imperfections in the DMA. This ambiguity could be resolved by relying on a narrower and more stable mobility standard than the protein ions used here, perhaps based on two DMAs of high resolution used in tandem.
Another possibility is that the flow inhomogeneities created by the laminarization grid would have insufficient time to decay prior to joining with the sheath gas. Most laminarization inlets in wind tunnels (as well as in DMAs having demonstrated high resolving power) place the screens in a region where the flow cross-section is considerably larger than in the test channel of the wind tunnel (or the separation region of the DMA), resulting in a more effective decay of grid inhomogeneities. In TSI's 3071 DMA, however, there is no contraction following the screens, so the flow cross-section in the screens is smaller than in the analyzing section by a factor equal to the screen transparency (33–50% for the screen most downstream). In our particular case, there are other sources of flow inhomogeneity besides the grid itself. Indeed, the outer ring in the laminarizer is attached to the screen with epoxy, and is so thin that it is hard to avoid some wetting by the glue of the screen region near this ring.
A common cause of nonideality is eccentricity between the two DMA cylinders. This is especially true in long DMAs of the Reischl type, where the bullet-shaped inner electrode is supported only on the downstream end. We do not think eccentricity is the primary cause of the limited resolution observed here because the inner electrode is centered both upstream and downstream and the gap R2–R1 between both electrodes is close to 1 cm. Knutson (Citation1971) has shown that a relative displacement ϵ in the parallel axes of the two electrodes increases the peak width by ∼2ϵ. Accordingly, to explain an anomalously wide peak with FWHM ∼ 7%, an unlikely eccentricity of 3.5% of 1 cm (0.35 mm) would be required. In fact, Knutson and Whitby (Citation1975b) have shown that FWHM as small as 2.5% is sometimes achieved in their 3071 prototype, though only at impractically low values of Q ∼ 1.5 L/min.
We are indebted to Prof. Michel Attoui (Paris) for perceptively noting that the laminarization screen of TSI's Electrical Aerosol Analyzer (EAA) predecessor to the 3071 DMA (Liu and Pui Citation1975) is step-free. Possibly for that reason the EAA stood flow rates of 50 lpm. It follows that the designers of the 3071 knew well how to avoid the screen problems here described, which must have resulted from a later accidental error. Tracing the origin of this error may be of more than historical interest, as several subsequent generations of TSI DMAs still suffer from decreased performance at comparably small flow rates. We have considered studying the resolution of the EAA with the same IgG aerosol. We were dissuaded by other flow stability and small particle transmission considerations. In particular, the disparate speeds of the sample and the sheath gas flows at the point where they meet is likely to produce an unstable mixing layer.
Aerosol analyzers typically covering a wide size range rarely need resolving powers in excess of 10–20. There are however special situations where the size distribution being investigated is very narrow, and an accurate determination of its width is needed. The case of viral particles is a clear example where the size range may extend to 100 nm, the particle may be highly monodisperse, and the ability to distinguish different virions with close sizes would be invaluable. Virion size analysis was in fact the application that motivated the present investigations. Many prior studies have examined viral particles with DMAs, two of which have demonstrated resolving powers superior to the one achieved here with IgG. The narrowest viral peak so far reported (FWHM = 0.039 for the ∼30 nm human rhinovirus) is that of Kallinger et al. (Citation2013), based on Tapcon's PDMA, a long variant of a DMA developed in Reischl's laboratory (Steiner Citation2011). This instrument achieves such relatively narrow peaks by combining an inlet trumpet with a well-centered long column supported on both ends. This inlet enables sheath gas flow rates of at least 50 lit/min under sufficiently laminar conditions to provide the reported performance. This positive result seems at first sight to suggest that the non-ideality having precluded a comparable performance here is indeed incomplete decay of grid turbulence. If this were true, the changes required to provide adequate laminarization in the 3071 DMA would be substantial. A second report of narrow viral mobility peaks (FWHM = 0.047 for the ∼25 nm PP7 bacteriophage) by You et al. (Citation2014) is most relevant here, as it used TSI's 3085 DMA (R1 = 9.37 mm, R2 = 19.05 mm, L = 49.87 mm). Except for the much smaller length, the 3085 is geometrically similar to the 3071, with a comparably modest recommended operational flow rate, and with no inlet trumpet. The operational condition of You et al. (Citation2014) were too slow for practical measurements (25 s of accumulation time at each DMA voltage, requiring 6.5 min to scan a narrow peak including only 15 data points). Nonetheless, they achieved FWHM = 0.047 at Q = 30 lit/min, suggesting that, after all, a laminarizing trumpet may not be essential. You et al. (Citation2014) studied also several large proteins, of which the narrowest peaks were obtained for the same sample of IgG used here (FWHM = 0.105). Their wider protein peak in a DMA capable of good resolving power and less sensitive to Brownian diffusion broadening than ours may seem puzzling. In our view, it simply confirms that our electrospray charge-reduction method is considerably better than theirs for proteins, as previously argued by Fernandez de la Mora (Citation2015). The same does apparently not hold for viral particles. Pending a more thorough evaluation of the performance of our modification of the 3071 DMA with monodisperse particles considerably larger than 10 nm, we cannot answer the question of its ability to analyze viral particles with singularly high resolution. We have nonetheless established the ability of a simple modification of this seasoned DMA to analyze 10 nm protein particles with performance considerably better than the 3071 as well as its younger kins.
5. Conclusions
i. | The presence of inner and outer steps in the laminarization screens of TSI's 3071 DMA is primarily responsible for its known loss of performance at relatively modest Re. Related problems include a substantial decrease in resolving power at a sheath gas flow rate of 30 lit/min, and a catastrophic flow transition at higher flow rates. | ||||
ii. | A step-free laminarization screen reduces substantially these two undesirable effects, at least up to a sheath gas flow rate Q ∼ 56 lit/min. A new flow instability arising with the step-free screens at Q ∼ 56 lit/min is removed by changing the tubular piece guiding the aerosol flow into the region where it mixes with the sheath gas. This second change is more costly than changing only the screen, and has less dramatic effects. However, this double modification stabilizes the flow and produces approximately symmetric peaks up to at least Q = 103 lit/min. | ||||
iii. | Using IgG ions as mobility standards, the narrowest peak seen in the modified instrument still has FWHM = 7.2% for the trimer (IgG)3 and 8.3% for the monomer. Flow nonidealities evidently subsist, since the IgG monomer tested with a halfmini DMA shows FWHM < 5%, while sheath/aerosol flow rate ratios as high as 100 have been used. |
Conflict of interest statement
Juan Fernandez de la Mora declares a personal interest in the companies NEC and SEADM commercializing various components used in this work.
Acknowledgments
The authors thank Jerome J. Schmitt and Charles Powell from NanoEngineering Corporation (NEC) for kindly providing laminarizer rings and modified inlet tubes commercialized by NEC. The authors are most grateful to Profs. Peter McMurry and Michel Attoui for their interest and help in this project, and to Drs. Rian You and Michael Zachariah for providing an exceptionally clean IgG sample solution.
References
- Chen, D. R., and Pui, D. Y. H. (1997). Numerical Modeling of the Performance of DMAs for Nanometer Aerosol Measurement. J. Aerosol Sci., 28:985–1004.
- Eichler, T. (1997). A Differential Mobility Analyzer for Ions and Nanoparticles: Laminar Flow at High Reynolds Numbers. Senior Graduation Thesis presented to Fachhochschule Offenburg, Germany ( May 1997).
- Eichler, T., de Juan, L., and Fernandez de la Mora, J. (1998). Improvement of the Resolution of TSI's 3071 DMA via Redesigned Sheath Air and Aerosol Inlets. Aerosol Sci. Technol., 29(1):39–49.
- Fernandez de la Mora, J. (2015). High-Resolution Mobility Analysis of Charge-Reduced Electrosprayed Protein Ions. Anal. Chem., 87:3729–3735.
- Fernandez de la Mora, J., and Kozlowski, J. (2013). Hand-Held Differential Mobility Analyzers of high Resolution for 1–30 nm Particles: Design and Fabrication Considerations. J. Aerosol Sci., 57:45–53.
- Kallinger, P., Weiss, V. U., Lehner, A., Allmaier, G., and Szymanski, W. W. (2013). Analysis and Handling of Bio-Nanoparticles and Environmental Nanoparticles Using Electrostatic Aerosol Mobility. Particuology, 11:14–19.
- Knutson, E. O. (1971). The Distribution of Electric Charge among the Particles of an Artificially Charged Aerosol, Ph.D. Thesis, University of Minnesota, Minneapolis, Minnesota; see appendix
- Knutson, E. O., and Whitby, K. T. (1975a). Aerosol Classification by Electric Mobility: Apparatus Theory and Applications. J. Aerosol Sci., 6:443–451.
- Knutson, E. O., and Whitby, K. T. (1975b). Accurate Measurement of Aerosol Electric Mobility Moments. J. Aerosol Sci., 6:453–460.
- Liu, B. Y. H., and Pui, D. Y. H. (1974). A Submicron Aerosol Standard and the Primary Absolute Calibration of the Condensation Nucleus. J. Colloid. Interf. Sci., 47(1):155–171.
- Liu, B. Y. H., and Pui, D. Y. H. (1975). On the Performance of the Electrical Aerosol Analyzer. J. Aerosol Sci., 6(3–4):255–254.
- Rosell-Llompart, J., Loscertales, I. G., Bingham, D., and Fernandez de la Mora, J. (1996). Sizing Nanoparticles and Ions with a Short Differential Mobility Analyzer. J. Aerosol Sci., 27(5):695–719.
- Steiner, G. (2011). High Resolution Mobility Spectrometry of Molecular Ions and Their Effect on The Charging Probabilities of Airborne Particles Under Bipolar Diffusion Charging Conditions, Ph.D. Thesis, University of Vienna.
- Stolzenburg, M. (1988). An Ultrafine Aerosol Size Distribution Measuring System. Ph.D. Thesis, University of Minnesota, Minneapolis, MN.
- Tammet, H. F. (1970). The Aspiration Method for the Determination of Atmospheric-ion Spectra. Israel Program for Scientific Translations, The original work in Russian was published in 1967, Jerusalem.
- You, R., Li, M., Guha, S., Mulholland, G. W., and Zachariah, M. R. (2014). Bionanoparticles as Candidate Reference Materials for Mobility Analysis of Nanoparticles. Anal. Chem., 86:6836–6842.