ABSTRACT
Conventional methods for total dust sampling from industrial high-temperature aerosols containing condensable species, e.g., in boilers of municipal solid waste incinerators (MSWI), are always influenced by condensation artifacts. Therefore, we extended the scope of a previously developed probe intended for size-fractionated aerosol sampling with reduced artifacts and employed it for total dust measurements. The dust is collected on quartz fiber filters, which are gravimetrically evaluated and chemically analyzed by energy dispersive X-ray spectroscopy and wet-chemical methods. First measurements in the boiler of an MSWI confirm that the probe is also suitable for artifact-minimized total dust sampling. The data are consistent with results from measurements with the size-fractionating method conducted in parallel. By combining the results of both sampling methods, we reveal the average chemical composition of the submicron particles in the aerosol, which is not accessible by one of the two methods alone.
© 2017 American Association for Aerosol Research
EDITOR:
1. Introduction
Total dust measurements in the flue gas of industrial plants are daily business, e.g., to control mandatory emission limits for fine dust or to validate the efficiency of dust reducing measures. Conducted at high temperatures, they furthermore allow investigating the influence of the firing conditions or the impact of chemical additives on the particulate aerosol close to the source. Of special interest in this context is the high-temperature aerosol in boilers of municipal solid waste incinerators (MSWI), which is under suspicion to be the main driver for high-temperature chlorine corrosion (Sorell Citation1997; Spiegel et al. Citation2003; Lee et al. Citation2007). Similar problems also exist for biomass-fired boilers (Michelsen et al. Citation1998; Nielsen et al. Citation2000) and to a lesser extent in coal-fired power plants (Bryers Citation1996). In contrast to size-fractioned aerosol measurements, which require a time-consuming analysis and careful interpretation, total dust measurements provide a rather straightforward means to assess changes in the particulate aerosol on a short time scale.
In Europe, total dust measurements are usually performed according to the standard EN 13284-1 (Citation2002). To achieve valid measurements, the standard requires in particular to sample in such a way that condensation and chemical reactions are avoided. However, this involves significant difficulties for high-temperature industrial aerosols which typically contain high amounts of condensable gaseous species such as alkali chlorides. There are basically two approaches to achieve a suppression of condensation: Either the aerosol is extracted from the boiler and instantaneously quenched (out-stack method) or the sampling substrate is located directly in the boiler (in-stack method). At high temperatures above approximately 600°C, all out-stack methods reported in the literature are known to introduce tremendous condensation artifacts (Valmari et al. Citation1999; Maguhn et al. Citation2003; Strand et al. Citation2004; Jiménez and Ballester Citation2005; Deuerling et al. Citation2010; Sippula et al. Citation2012; Yang et al. Citation2013, Citation2015). The reason is that the saturation vapor pressure of, e.g., NaCl and KCl drops by a factor of more than 106 when cooling from 1000 to 500°C (Ewing and Stern Citation1974). Hence, a dilution factor of the same magnitude is required to efficiently suppress condensation, which is technically not achievable.
On the other hand, there have been several approaches for in-stack sampling techniques. Valmari et al. (Citation1999) and Brunner et al. (Citation2013) used high-temperature low-pressure cascade impactors to sample particles inside the boilers of biomass-fired energy plants and MSWI. Although this sampling technique avoids condensation artifacts, particle evaporation and vapor condensation at the low pressure stages of the impactor are known to alter the particle size distributions (Valmari et al. Citation1998). Furthermore, in the case of Brunner et al. (Citation2013), the method is limited to particle sizes below about 1 µm, since the aerosol is extracted normal to the main flow which limits the aspiration efficiency for larger particles due to their inertia. In other words, the sampling is not conducted isokinetically as it would be required by EN 13284-1 (Citation2002). Valmari et al. (Citation1999) circumvented this problem by using a pre-cyclone sampling in the flow direction. The same group also collected particles directly after the pre-cyclone on quartz fiber filters, which already provides a suitable method for total dust sampling (Valmari et al. Citation1999). Nevertheless, the disadvantage remains that post-reactions during cool-down are not prevented and that the content of the pre-cyclone has to be analyzed separately, which significantly increases the effort for data evaluation.
Recently, Schumacher et al. (Citation2016) developed a novel probe that allows a size-fractionated analysis of concentration, morphology and chemical composition of the high-temperature aerosol in MSWI. The probe isokinetically samples in-stack and prevents condensation and post-reactions as well as particle deposits upstream of the sampling substrate. Thus, it is a perfect prerequisite to develop a total dust sampling probe that fulfills the basic requirements of EN 13284-1 (Citation2002). To achieve this goal, we modified the size-fractionating probe such that it collects all particles on a single fibrous filter substrate, which allows a gravimetric and chemical characterization of the collected dust. The modified probe was employed for first total dust measurements in an industrial MSWI and the results were validated by a direct comparison with the size-fractionating sampling method.
2. Methods and materials
In the following, we describe the general design of the sampling probe and the principle for total dust measurements. Furthermore, we give details on the methods applied for gravimetric and chemical analysis of the loaded filters.
2.1. Design and use of the sampling probe
The original probe by Schumacher et al. (Citation2016) is shown in . The body is made of the heat- and corrosion-resistant steel 1.4841 and mounted via a holder to the tip of a water-cooled lance for entering the boiler. The interior of the probe contains two different sampling stages: Larger particles are sampled on a 1 mm thick steel impaction plate. The aerodynamic cutoff diameter of the impactor increases from 16 µm at 350°C to 37 µm at 1000°C. To collect the passing smaller particles, a 15 µm thick galvanically grown nickel membrane with 10 µm pore diameter and 50 µm pore separation (3.6% porosity) is used (Gehrke Citation2008). The particle covered substrates are analyzed by scanning electron microscopy (SEM) and energy-dispersive X-ray spectroscopy (EDX).
Figure 1. Cross section of the probe for particle sampling. (a) Original design for size-fractionated sampling of individual particles on an impaction plate and a membrane. (b) Adapted approach for total dust sampling on a quartz fiber filter.
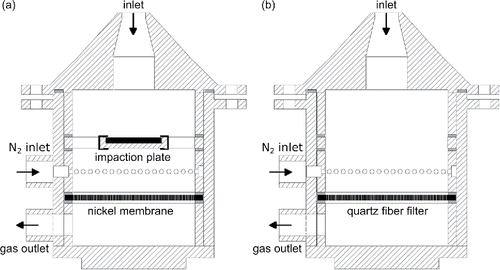
The probe can be adapted for total dust measurements by removing the impaction plate and replacing the nickel membrane by a quartz fiber filter as shown in . Since the sampling substrates can easily be exchanged by opening the flange between cap and housing, alternating measurements with both methods using the same probe are possible. The probe is designed for isokinetic sampling at a flue gas velocity of 4.5 m/s. We use the same parameters as in our previous study (Schumacher et al. Citation2016), i.e., a 3.1 mm diameter inlet nozzle and an operating flow rate of 2 l/min, which is provided by a rotary vane pump exhausting at the gas outlet and stabilized by a mass flow controller. However, for total dust measurements the probe can easily be adapted for isokinetic sampling at other velocities by using different nozzles and/or adjusting the flow rate.
To avoid artifacts by condensation, for both methods the probe is flushed with an excess of pure N2 and pre-heated in the boiler before sampling. By swiveling in the probe in the flue gas stream and switching off the N2 flux the sampling is started. After switching the N2 flux back on, the probe is extracted from the boiler and the sampling substrates cool down in the inert N2 atmosphere to avoid chemical post-reactions. After extraction from the probe, the sampling substrates are immediately transferred to conductive plastic boxes in order to avoid electrostatic charging and subsequent fiber or particle losses from the filter. The boxes themselves are stored in a desiccator.
2.2. Gravimetric determination of the dust concentration
As filter medium for the total dust measurements we used Tissuquartz 2500QAT-UP filters manufactured by Pall® Life Sciences. The filters consist of pure quartz (SiO2) and do not contain any binders, which prohibits weight losses at high temperatures. The filters are heat-treated and washed with ultra pure soft water by the manufacturer, which leads to a low level of residual ions and trace organics, which otherwise might deteriorate the chemical analysis of the sampled dust. According to the manufacturer, the filters withstand high temperatures up to 1093°C (2000°F) without decomposing and are resistant to harsh environments such as the acidic and corrosive atmosphere in the boiler of an MSWI.
Before the measurement campaign, the quartz fiber filters were pre-conditioned at (20 ± 1)°C and (50 ± 5)% relative humidity (RH) in a weighing room fulfilling the requirements of the European standard DIN EN 12341-1 (Citation2014). After conditioning for 24 h, the filters were weighed twice using a Sartorius micro balance with 24 h between the measurements. The deviation between the two measurements was typically below 10 µg for an average filter weight of about 37 mg, i.e., the relative error is below 0.1%.
The dust loaded filters were removed from the desiccator and conditioned again for 24 h in the weighing room and weighed twice with 24 h between the measurements. We note that the transfer from the desiccator with a humidity below 1% RH to the weighing room with 50% RH might in principle increase the mass of hygroscopic constituents of the dust. For example, for particles from biomass-fired boilers a slight water uptake well below 10% has been previously observed (Christensen et al. Citation1998). However, at 50% RH there is no effect expected for the most abundant components NaCl and KCl, which show hygroscopic growth only above 70% RH (Park et al. Citation2009). A slight effect might only occur for CaCl2, for which a slight growth already starts around 30% RH (Park et al. Citation2009). Nevertheless, we consider this potential inaccuracy as acceptable, especially since conditioning the samples under different conditions than used for weighing is not well defined. Furthermore, weighing at low humidity is generally not recommended since such conditions promote electrostatic charging of the specimens.
2.3. Chemical analysis of the loaded filters
We employed two different methods for analyzing the chemical composition of the particles sampled on the filters. For EDX analysis, we used a JEOL 7500F scanning electron microscope with an EDAX Apollo XL EDX-analyzer. The working distance was set to 8 mm and an acceleration voltage of 20 keV was used, which is sufficient to detect also elements with high atomic numbers such as Fe and Zn. For sample preparation, a wedge covering about 15% of the whole filter was cut out and plated with Au to avoid charging of the poorly conducting material. Since Au is not a common component of municipal waste, the plating does not negatively influence the chemical analysis by EDX. On the prepared wedge, five arbitrary positions were chosen for taking area-averaged EDX spectra.
For wet-chemical analysis, all filter samples (excluding the wedges used for EDX analysis) were first extracted in purified water using an ultrasonic bath to analyze the water-soluble anions. The aqueous extract was filtered using an 0.45 µm polypropylene (PP) membrane filter to separate insoluble residues. The fraction of the individual anions in the aqueous extract was determined by ion chromatography (Metrohm 761 Compact IC pro) according to DIN EN ISO 10304 (Citation2009). Afterwards, the filter samples as well as the PP filters containing the insoluble residues were digested in a mixture of hydrofluoric and nitric acid in a microwave oven at 200°C. The digestions and the aqueous extracts were analyzed by inductively coupled plasma optical emission spectrometry (ICP-OES, Thermo Fisher iCAP 6500) according to DIN EN ISO 11885 (Citation2009) to determine the metallic cations. Three unused filters were analyzed to identify the blank values which were then subtracted afterwards from the data of the loaded filters.
2.4. Description of the MSWI and conducted measurements
A series of measurements was conducted at the Gemeinschaftskraftwerk Schweinfurt (GKS) GmbH. A schematic cross section of one combustion line is depicted in the online supplemental information (SI). More details about the plant can be found elsewhere (Deuerling et al. Citation2010, Schumacher et al. Citation2016). The measurement positions were located in the first pass at 950°C, the second pass at 650°C, the third pass directly behind the final superheater at 550°C, and the fourth pass behind the third economizer at 250°C. The probe was inserted 1.50 m into the boiler, which is 3 m wide. At each position, three total dust measurements were performed. To validate the results and to get additional information, after each total dust measurement also a size-fractionated particle sampling using the setup in was conducted. The interval between two consecutive measurements was about 30 min.
For both sampling methods, the probe was pre-heated in the boiler under N2 excess to bring it into thermal equilibrium with the environment. This was assumed to be reached when the temperature measured by an 1 mm diameter type K thermocouple entering the probe from the side by 2 mm just above the membrane got stable, which took approximately ten minutes. We note that accurate corrections for radiation losses and conduction along the thermocouple wires would be needed to derive the actual gas temperature inside the probe, but this is a very sophisticated task and goes beyond the scope of this work.
For total dust measurements, we employed a sampling time of four minutes, except for the second pass, where only two minutes were used due to expected higher dust concentrations. Those sampling times were shown in preliminary tests to yield a sufficient collected mass for gravimetrical evaluation without overloading the filter. The latter was always checked during the measurements by controlling that the mass flow controller was able to keep the sampling flow rate constant at 2 l/min despite the increasing pressure drop caused by filter loading. For the size-fractionating method, we collected particles for 20 s which were shown before to be a suitable sampling time (Schumacher et al. Citation2016).
3. Results and discussion
In the following, we present and discuss our measurements on concentration and chemical composition of the total dust and deduce from that the composition of the submicron particles.
3.1. Total dust concentrations
shows the total mass concentrations derived from gravimetrical analysis of the loaded quartz fiber filters. To derive airborne mass concentrations from the weight of the particles collected on the filters (typically several milligrams), the values are normalized to the sampled gas volume under normal conditions (0°C, 1013.25 hPa). All data shown are averaged over three samples for each pass; the error bars indicate the corresponding standard deviations. In comparison, the uncertainty resulting from deviations between the two consecutive weighings of the same filter (below 30 mg/m3) is negligibly small.
Figure 2. Total dust concentration derived from gravimetrical analysis of the loaded quartz fiber filters. The data are averaged over three filters for each of the four passes. Error bars indicate the corresponding standard deviations.
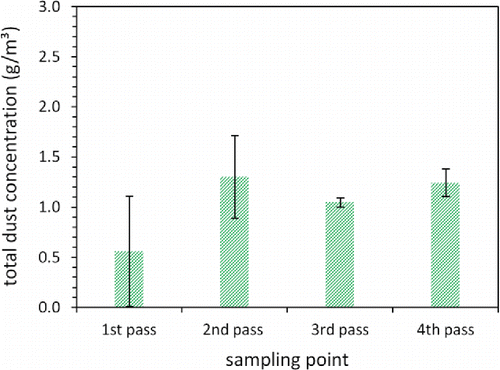
We find a low dust concentration of 0.5 g/m3 in the first pass, whereas the concentration in the remaining three passes stays rather constant at about 1 g/m3. The total dust concentrations are somewhat lower than previously reported values around 2 g/m3 for the same MSWI (Deuerling et al. Citation2010; Schumacher et al. Citation2016), although waste mass flow, primary air supply and movement of the grate, which might more or less stir the fire, did on average not significantly differ from the previous measurement campaigns. However, the difference is partially covered by the high standard deviations caused by fluctuations on short time scales.
To validate our results, we compare the values derived from the gravimetrical filter analysis to total mass concentrations determined by the size-fractionating method. The detailed approach how to derive the latter values can be found in Schumacher et al. (Citation2016) and is only briefly summarized here: The particles are counted on SEM images and sized according to their projected area diameter (diameter of a disk with the same projected area as the particle). This method is frequently applied to determine particle size distributions down to the nanometer regime (Terry Citation1995; Schmidt et al. Citation2009; Wall et al. Citation2014) and has been shown to yield reasonable agreement with other techniques (Klein et al. Citation2011; Tuoriniemi et al. Citation2014). The particle counts are weighted with the particle size dependent collection efficiencies of the sampling substrate and normalized to the sampled gas volume under normal conditions to obtain the airborne particle concentrations. In order to derive mass size distributions, the particles are assumed to be spheres having the projected area diameter and a density of 2.2 g/cm3, which is a typical average value for fly ash particles in MSWI (Deuerling et al. Citation2010). Such a transformation from number to mass size distributions has been previously shown to yield reasonable agreement with direct measurements of the mass concentration using an impactor (Christensen et al. Citation1998).
The results from the measurement campaign averaged over two samples per pass are shown in . The number size distributions shown in are governed by particles having a modal diameter of about 300 nm. The total concentration is very low in the first pass, which confirms once more that an artificial condensation of salt vapors, which would lead to high number concentrations of small particles, is efficiently suppressed. In the course of the boiler, the amount of submicron particles continuously increases to reach its maximum in the fourth pass. The strongest increase of the number concentration is observed between the measurement positions in the first and second pass. This is in contrast to the results previously reported by Schumacher et al. (Citation2016), where the primary condensation zone was located between the measurement positions in the second and third pass. However, because of the very steep vapor pressure curve of alkali chlorines in the temperature range around 650°C (Ewing and Stern Citation1974), the exact point at which supersaturation is reached is very sensitive to the available vapor concentration and to the temperature profile in the boiler. Since both factors always underlie certain fluctuations, it is not surprising that the onset of condensation might shift by a few meters in the course of the boiler either before or behind the measurement position in the second pass.
Figure 3. Number (a) and mass (b) size distributions of particles in the four passes of the boiler measured with the size-fractionating method. Error bars indicate the standard deviations between different positions of the sampling substrates.
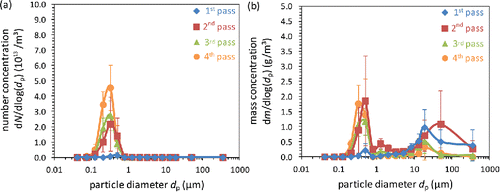
The corresponding mass size distribution curves in show the typical bimodal characteristic of the aerosol with a fine and a coarse mode also reported before for the same MSWI (Deuerling et al. Citation2010; Schumacher et al. Citation2016) and typical also for biomass-fired plants (Valmari et al. Citation1998; Valmari et al. Citation1999; Jiménez and Ballester Citation2005). The growing fine mode corresponds to the particles already discussed in the context of . In contrast, the mass concentration of the coarse mode gradually decreases in the course of the boiler, especially at the right flank of the peak, which has also been observed in biomass-fired boilers (Valmari et al. Citation1998, Citation1999). This is presumably caused by particle losses in the bends of the boiler and on the superheater packages, where particles in the micron range are precipitated with high efficiency (Haugen et al. Citation2013).
To compare the results of the size-fractionating method with the total dust measurements in , we integrate the mass distribution curves in . The resulting total dust concentrations are shown as light hatched (green) bars in . As additional information, the dark hatched (yellow) and filled (orange) bars represent the mass fractions of the fine and the coarse mode, respectively. According to the minimum around 2 µm in the bimodal mass distributions in , the dividing line between fine and coarse mode was drawn at 2 µm.
Figure 4. Total dust concentration (light hatched [green]) and partial concentration of the coarse mode (>2 µm, dark hatched [yellow]) and the fine mode (<2 µm, filled [orange]) derived from the size-fractionating method. Error bars indicate the standard deviation between different positions on the sampling substrates.
![Figure 4. Total dust concentration (light hatched [green]) and partial concentration of the coarse mode (>2 µm, dark hatched [yellow]) and the fine mode (<2 µm, filled [orange]) derived from the size-fractionating method. Error bars indicate the standard deviation between different positions on the sampling substrates.](/cms/asset/5f3ec994-e65b-4e4b-8900-381bc42b50cf/uast_a_1330534_f0004_oc.gif)
We find total dust concentrations of about 1 g/m3 in the first, third and fourth pass, whereas the value of about 2 g/m3 is higher in the second pass. The mass of the fine mode apparently slightly decreases from the second over the third to the fourth pass, although the number concentration increases (compare ). This effect is caused by a slight shift of the modal diameter of the number size distributions to smaller particle sizes.
In the third and first pass, we find a reasonable agreement with the total dust masses derived gravimetrically from the quartz fiber filters (compare ), both showing a total dust concentration around 1 g/m3. Also in the first two passes the results of the two methods agree within the measurement uncertainty (see the error bars), but the deviation between the average values as well as the standard deviations are considerably larger than for the third and fourth pass.
This observation can be explained by the different composition of the particles: In the last two passes, the aerosol is dominated by submicron particles, which usually have a compact shape (see the SI) as it has also been reported in other field studies (Christensen et al. Citation1998; Valmari et al. Citation1999). For such particles the calculation of the volume by assuming a sphere with the same projected area diameter is a good approximation. Furthermore, the submicron particles form by condensation and are therefore presumably composed of the main constituents of the condensable gas, which are NaCl and KCl. Since the density of these salts (ρNaCl = 2.16 g/cm3 and ρKCl = 1.98 g/cm3) is rather close to the assumed average particle density of 2.2 g/cm3, the calculation of the mass from the volume is also expected to be a reasonable approximation. Furthermore, the rather homogenous composition of the aerosol leads to small standard deviations.
In contrast, in the first two passes the total mass is dominated by the contribution of coarse particles, which are emitted as primary particles from the grate. Their shape is in general much more irregular (see the SI), which is also typical for particles in biomass-fired boilers (Christensen et al. Citation1998; Valmari et al. Citation1999). For example, flat particles are frequently observed, for which the assumption of an equivalent sphere with the same projected area diameter yields an overestimation of the particle volume and therefore also of the particle mass. Furthermore, often large agglomerates composed of individual smaller particles (see the SI) are observed. Due to their porous structure they have a lower effective density than the assumed average bulk density, which also leads to an overestimation of the particle mass. Finally, the chemical composition of the primary particles is much more versatile than for the secondary condensed submicron salt particles, so that also the bulk density might differ much more from the average value of 2.2 g/cm3 assumed. Those effects may explain why we calculate in the first two passes lower total masses from the size-fractionating method than found on the quartz fiber filters. Moreover, they make the high standard deviations comprehensible. Nevertheless, the results show that the assumptions made are reasonable within a factor of two.
Finally, we find that the increase of the total dust mass from the first to the second pass, which accounts for about 0.7 g/m3 for the quartz fiber filters and 0.9 g/m3 for the size-fractionating method, is similar in both cases. This is consistent with our previous interpretation since this increase is almost exclusively formed by the newly condensed salt particles, for which the calculation of the mass is more reliable.
3.2. Chemical composition of the collected dust
The chemical composition of the collected dust was determined taking area-averaged EDX spectra over five images with a size of 480 × 360 µm2 for each pass. The image size was chosen such that a sufficient amount of large particles is considered. Characteristic SEM images of the quartz fiber filters covered by particles after total dust sampling are shown in the SI. Since the filter material consists of SiO2, the elements Si and O were not taken into account for the analysis. However, the fraction of O was calculated from the mass fractions of the other elements under the thermodynamically reasonable assumption that they are fully oxidized or halogenated (Schlumberger and Bühler Citation2013).
The results of the EDX analysis are shown in for all four passes. The most obvious evolution in the course of the boiler is a strong increase of the alkali chloride fraction. Whereas it is negligible in the first pass, in the second pass already a substantial amount of about 40% is found. The mass fraction further increases in the third and fourth pass to more than 60%. The remaining fraction of the particles on the one hand consist of Al and Ca (and Si not detected here, but by the size-fractionating method), which are known to form complex aluminosilicates (Williams Citation2005). Further substantial contributions come from the metallic cations Mg, Fe, Cr and Ti, whose oxides are also typical components of fly ash (Lam et al. Citation2010). Furthermore, about 5% of Zn are found, which indicates the presence of Zn salts (Li and Spiegel Citation2004; Bankiewicz et al. Citation2009). On the anionic side, we find besides Cl and O preferentially S, whereas P and F occur only in traces over the whole boiler.
Figure 5. Average chemical composition of the total dust collected on the quartz fiber filters in all four passes of the boiler. (a) EDX analysis of five randomly selected areas on one filter per pass. (b) Wet-chemical analysis of three complete filters per pass.
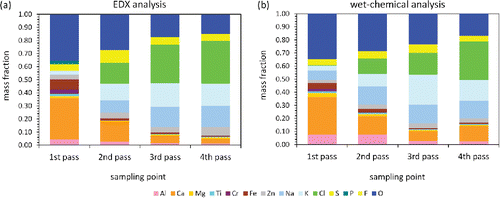
We note that our approach to determine the chemical composition of the particles underlies certain limitations. First, there is a depth profile of the particle deposition on the filter (large particles on the filter surface, small particles preferentially inside the filter), which may bias the chemical analysis due to the finite penetration depth of the electron beam. For the same reason, EDX is in principle more sensitive to the surface composition of large particles than to the average bulk composition. If particles consist of a core with a surrounding shell, which is typical for condensation on solid nuclei, this might lead to noticeable deviations. Finally, the EDX analysis takes into account only a very small fraction of the whole filter. In order to evaluate how representative our EDX approach nevertheless is, we performed a wet-chemical analysis of the whole filters. This method should circumvent all the disadvantages of the EDX analysis discussed above, since all particles are taken into account with the correct mass fractions, the bulk and not only the surface composition is investigated, and the whole filter material is taken as statistical basis.
The results of the wet-chemical analysis are presented in . They are in reasonable agreement with the EDX analysis shown in , which leads to the conclusion that the EDX analysis of a small fraction of the whole filter already yields a good approximation to the chemical composition of the total dust. Thereby, the great advantage of the EDX analysis is that it is much less time consuming than a comprehensive wet-chemical analysis. We suspect that the agreement can be further improved by performing EDX on more areas distributed over the whole filter surface. The most prominent difference in absolute numbers between the two methods is that we find lower chlorine contents by the wet-chemical analysis. A possible explanation might be that the amount of large particles typically having lower salt fractions is underrepresented in the images investigated by EDX.
For comparison, the results of the chemical analysis of the particles collected by the size-fractionating method are presented in the SI. They also show a steady increase of the alkali chlorine fraction over the four passes and are in excellent agreement with previously reported results (Schumacher et al. Citation2016).
3.3. Chemical composition of the submicron particles
We already implicitly assumed that the submicron particles are mainly composed of alkali chlorides since they originate from condensation of the most abundant condensable gases. However, a direct chemical analysis of those particles is not possible with the methods presented above since the single particle analysis does not yield a sufficient signal-to-background ratio for small particles, whereas the total dust measurements only yield average values over all particle sizes. Nevertheless, we can combine both techniques to get an estimate for the average composition of the submicron particles in order to validate our assumption.
We use the fact that the analysis of the quartz fiber filters yields an average composition of all particles, whereas we can determine the average composition of the large particles from the size-fractionating method. If we additionally know the relative mass fractions of small and large particles, the average composition of the small particles can be calculated as follows: For each chemical element , the elemental fraction
found on the quartz fiber filter can be regarded as a linear combination of a certain size fraction
of small particles with an average elemental fraction
and a size fraction
of coarse particles with a different elemental fraction
:
[1]
The size fractions of the small and large particles can be directly determined from as share of the light hatched (orange) bars (dust mass <2 µm) and dark hatched (yellow) bars (dust mass >2 µm) compared to the filled (green) bars (total dust mass). The corresponding values are listed in .
Table 1. Mass size fractions of the particles smaller and larger than 2 µm in all four passes.
Furthermore, the elemental fractions found on the filters are known from the chemical analysis. In the following, we use the EDX results from instead of the wet-chemical data from in order not to intermix two different methods.
The elemental fractions of the larger particles can be approximated by taking the mass weighted average over the size-fractionated chemical compositions shown in the SI. The results are presented in for all four passes; since the highest mass contribution comes from the largest particles, the mass weighted average is similar to the composition in the highest size classes. To make the data directly comparable to the filter measurements, for which Si was not taken into account, Si was arithmetically removed so that again
is fulfilled. Since the Si fraction is typically below 5%, this correction leads only to small deviations for the other elements.
Figure 6. (a) Mass-averaged chemical composition of the particles >2 µm from measured data. (b) Best fit for the average composition of the particles <2 µm.
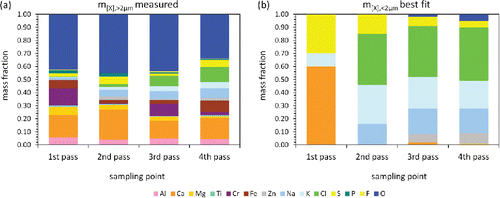
In principle, now is the only unknown left in Equation (Equation1
[1] ) and can be calculated. However, sometimes the results break the boundary condition
. This means concretely that for those cases the measured chemical difference between the particles > 2 µm and the average over all particles is too large to be explained by the available size fraction
of small particles. This is especially the case in the first pass, where the size fraction of 6% of small particles can explain only small differences between the two measurements.
Therefore, we fitted the elemental fractions of the small particles () such that they minimize the deviations between the compositions calculated by Equation (Equation1
[1] ) and measured on the quartz fiber filters and thereby fulfill
and
(see the SI for more details). In the second to fourth pass, the approach shows that the particles smaller than 2 µm consist to more than 80% of alkali chlorides confirming our previous assumption. The next most common element is S, indicating a certain fraction of sulfates. In the third and fourth pass, we additionally find Zn, which is known to form eutectic mixtures with other metal chlorides (Li and Spiegel Citation2004; Bankiewicz et al. Citation2009). Due to the low melting points of those compositions they condense only in the rear of the boiler. In the first pass, the best fit yields a completely different composition than for the other three passes. The particles do not contain any chlorides, but seem to be mainly composed of Ca, K and S indicating the presence of CaSO4 and K2SO4. We point out that for a completely consistent picture the fit should yield also O as component, but this inconsistency is covered by the inaccuracy of the approach.
Our findings are in line with the general mechanisms described in the literature for K compounds in biomass-fired plants: At high temperatures, the contents of KCl, K2SO4, HCl and SO2 in the gas phase are determined by the equilibrium of the corresponding sulfation reaction at about 800°C, since the vanishing reaction rate at lower temperatures prevents further sulfation, although it would be thermodynamically favored (“quenched equilibrium”, Christensen et al. Citation1998). The underlying reactions have been further elaborated by Glarborg and Marshall (Citation2005) and Jiménez and Ballester (Citation2007). From this gas phase, K2SO4 condensates first during cool down because of its low vapor pressure compared to KCl, which condenses at lower temperatures to eventually form the main part of the fine mode (Glarborg and Marshall Citation2005; Jiménez and Ballester Citation2007). The same mechanism has also been proposed by Brunner et al. (Citation2013) for Na2SO4 and NaCl after investigating the particles smaller than 1 µm in an MSWI aerosol using an in-stack high-temperature cascade impactor. A similar mechanism is also reasonable for the system CaSO4/CaCl2.
4. Conclusions
In this work, we modified a probe previously developed for size-fractionated sampling of individual particles to make it suitable for total dust sampling at high temperatures without artifacts due to condensation of salt vapors. The dust is collected on filter substrates applicable for gravimetrical and chemical characterization. The probe was employed in a first measurement campaign in the four passes of the boiler of an industrial MSWI yielding total dust concentration on the order of 1 g/m3. In the third and fourth pass, we find a reasonable agreement with the dust masses derived from the size-fractionating method, whereas we conclude that the latter systematically slightly overestimates the mass concentrations in the first two passes. By combining the results of both sampling methods, we show that the submicron particles in the first pass presumably consist of condensed sulfates, whereas in the remaining passes almost exclusively alkali chlorides are found.
UAST_1330534_Supplemental_File.zip
Download Zip (6.4 MB)Funding
The authors thank the German Federal Ministry of Education and Research (BMBF) for financial support within the project VOKos (Grant No 03 × 3589).
References
- Bankiewicz, D., Yrjas, P., and Hupa, M. (2009). High-Temperature Corrosion of Superheater Tube Materials Exposed to Zinc Salts. Energy Fuels, 23:3469–3474.
- Brunner, T., Fluch, J., Obernberger, I., and Warnecke, R. (2013). Investigations of Aerosol Formation Pathways During MSW Combustion Based on High-Temperature Impactor Measurements. Fuel Process. Technol., 105:154–160.
- Bryers, R. W. (1996). Fireside Slagging, Fouling, and High-Temperature Corrosion of Heat-Transfer Surface Due to Impurities in Steam-Raising Fuels. Prog. Energy Combust. Sci., 22:29–120.
- Christensen, K. A., Stenholm, M., and Livbjerg, H. (1998). The Formation of Submicron Aerosol Particles, HCl and SO2 in Straw-Fired Boilers. J. Aerosol Sci., 29:421–444.
- Deuerling, C., Maguhn, J., Nordsieck, H., Warnecke, R., and Zimmermann, R. (2010). Measurement System for Characterization of Gas and Particle Phase of High Temperature Combustion. Aerosol Sci. Technol., 44:1–9.
- DIN EN ISO 10304. (2009). Water quality - Determination of Dissolved Anions by Liquid Chromatography of Ions - Part 1: Determination of Bromide, Chloride, Fluoride, Nitrate, Nitrite, Phosphate and Sulfate. https://www.beuth.de/de/norm/din-en-iso-10304-1/117316025
- DIN EN ISO 11885. (2009). Water Quality - Determination of Selected Elements by Inductively Coupled Plasma Optical Emission Spectrometry (ICP-OES). https://www.beuth.de/de/norm/din-en-iso-11885/118931490
- DIN EN 12341-1 (2014). Ambient Air - Standard Gravimetric Measurement Method for the Determination of the PM10 or PM2.5 Mass Concentration of Suspended Particulate Matter. https://www.beuth.de/de/norm/din-en-12341/195550191
- EN 13284-1. (2002). Stationary Source Emissions - Determination of Low Range Mass Concentration of Dust - Part 1: Manual Gravimetric Method. https://www.beuth.de/de/norm/din-en-13284-1/46953658
- Ewing, C. T., and Stern, K. H. (1974). Equilibrium Vaporization Rates and Vapor Pressures of Solid and Liquid Sodium Chloride, Potassium Chloride, Potassium Bromide, Cesium Iodide, and Lithium Fluoride. J. Phys. Chem., 78:1998–2005.
- Gehrke, I. (2008). Metallische Mikrosiebe - Mikrotechnische Herstellung und filtertechnische Charakterisierung. Logos Verlag Berlin GmbH, Berlin.
- Glarborg, P., and Marshall, P. (2005). Mechanism and Modeling of the Formation of Gaseous Alkali Sulfates. Comb. Flame, 141:22–39.
- Haugen, N. E. L., Kragset, S., Bugge, M., Warnecke, R., and Weghaus, M. (2013). MSWI Super Heater Tube Bundle: Particle Impaction Efficiency and Size Distribution. Fuel Process. Technol., 106:416–422.
- Jiménez, S., and Ballester, J. (2005). A Comparative Study of Different Methods for the Sampling of High Temperature Combustion Aerosols. Aerosol Sci. Technol., 39:811–821.
- Jiménez, S., and Ballester, J. (2005). Influence of Operating Conditions and the Role of Sulfur in the Formation of Aerosols from Biomass Combustion. Combust. Flame, 140:346–358.
- Jiménez, S., and Ballester, J. (2007). Formation of Alkali Sulphate Aerosols in Biomass Combustion. Fuel, 86:486–493.
- Kawahara, Y. (2002). High Temperature Corrosion Mechanisms and Effect of Alloying Elements for Materials Used in Waste Incineration Environment. Corros. Sci., 44:223–245.
- Klein, C. L., Comero, S., Stahlmecke, B., Romazanov, J., Kuhlbusch, T. A. J., Van Doren, E., De Temmerman, P.-J., Mast, J., Wick, P., Krug, H., Locoro, G., Hund-Rinke, K., Kördel, W., Friedrichs, S., Maier, G., Werner, J., Linsinger, T., and Gawlik, B. M. (2011). NM-Series of Representative Manufactured Nanomaterials; NM-300 Silver Characterisation, Stability, Homogeneity. Publications Office of the European Union, Luxembourg, EUR 24693 EN, JRC 60709. http://publications.jrc.ec.europa.eu/repository/handle/JRC60709
- Lam, C. H., Ip, A. W., Barford, J. P., and McKay, G. (2010). Use of Incineration MSW Ash: A Review. Sustainability, 2:1943–1968.
- Lee, S.-H., Krumb, H., Themelis, N. J., and Castaldi, M. J. (2007). High-Temperature Corrosion in Waste-to-Energy Boilers. J. Therm. Spray Technol., 16:104–110.
- Li, Y. S, and Spiegel, M. (2004). Models Describing the Degradation of FeAl and NiAl Alloys Induced by ZnCl2–KCl Melt at 400–450°C. Corros. Sci. 46:2009–2023.
- Maguhn, J., Karg, E., Kettrup, A., and Zimmermann, R. (2003). On-Line Analysis of the Size Distribution of Fine and Ultrafine Aerosol Particles in Flue and Stack Gas of a Municipal Waste Incineration Plant: Effects of Dynamic Process Control Measures and Emission Reduction Devices. Environ. Sci. Technol., 37:4761–4770.
- Michelsen, H. P., Frandsen, F., Dam-Johansen, K., and Larsen, O. H. (1998). Deposition and High Temperature Corrosion in a 10 MW Straw Fired Boiler. Fuel Process. Technol., 54:95–108.
- Nielsen, H. P., Frandsen, F. J., Dam-Johansen, K., and Baxter, L. L. (2000). The Implications of Chlorine-Associated Corrosion on the Operation of Biomass-Fired Boilers. Prog. Energy Combust. Sci., 26:283–298.
- Park, K., Kim, J. S., and Miller, A. L. (2009). A Study on Effects of Size and Structure on Hygroscopicity of Nanoparticles Using a Tandem Differential Mobility Analyzer and TEM. J. Nanopart. Res., 11:175–183.
- Schmidt, F., Stahlmecke, B., Kaminski, H., and Finger, H. (2009). Characterisation of Soot as a Test Dust for Air Filters. Filtr. Sep. Int. Ed., 9:24–28.
- Schlumberger, S., and Bühler, J. (2013). Metallrückgewinnung aus Filterstäuben der thermischen Abfallbehandlung nach dem FLUREC-Verfahren. Aschen–Schlacken–Stäube aus Abfallverbrennung und Metallurgie, TK Verlag Karl Thomé-Kozmiensky, Neuruppin.
- Schumacher, S., Lindermann, J., Stahlmecke, B., Khot, A., Zeiner, T., van der Zwaag, T., Nordsieck, H., Warnecke, R., and Asbach, C. (2016). Particle Sampling in Boilers of Waste Incineration Plants for Characterizing Corrosion Relevant Species. Corros. Sci., 110:82–90.
- Sippula, O., Koponen, T., and Jokiniemi, J. (2012). Behavior of Alkali Metal Aerosol in a High-Temperature Porous Tube Sampling Probe. Aerosol Sci. Technol., 46:1151–1162.
- Sorell, G. (1997). The Role of Chlorine in High Temperature Corrosion in Waste-to-Energy Plants. Mater. High Temp., 14:207–220.
- Spiegel, M., Zahs, A., and Grabke, H. J. (2003). Fundamental Aspects of Chlorine Induced Corrosion in Power Plants. Mater. High Temp., 20:153–159.
- Strand, M., Bohgard, M., Swietlicki, E., Gharibi, A., and Sanati, M. (2004). Laboratory and Field Test of a Sampling Method for Characterization of Combustion Aerosols at High Temperatures. Aerosol Sci. Technol., 38:757–765.
- Terry, K. W. (1995). Particle Size Distribution of Airborne Dusts Using a Scanning Electron Microscope. Aerosol Sci. Technol., 23:475–478.
- Tuoriniemi, J., Johnsson, A. C. J., Holmberg, J. P., Gustafsson, S., Gallego-Urrea, J. A., Olsson, E., Pettersson, J. B. C., and Hassellöv, M. (2014). Intermethod Comparison of the Particle Size Distributions of Colloidal Silica Nanoparticles. Sci. Technol. Adv. Mater., 15:035009.
- Valmari, T., Kauppinen, E. I., Kurkela, J., Jokiniemi, J. K., Sfiris, G., and Revitzer, H. (1998). Fly Ash Formation and Deposition During Fluidized Bed Combustion of Willow. J. Aerosol Sci., 29:445–459.
- Valmari, T., Lind, T. M., Kauppinen, E. I., Sfiris, G., Nilsson, K., and Maenhaut, W. (1999). Field Study on Ash Behavior During Circulating Fluidized-Bed Combustion of Biomass. 1. Ash Formation. Energy Fuels, 13:379–389.
- Valmari, T., Lind, T. M., Kauppinen, E. I., Sfiris, G., Nilsson, K., and Maenhaut, W. (1999). Field Study on Ash Behavior During Circulating Fluidized-Bed Combustion of Biomass. 2. Ash Deposition and Alkali Vapor Condensation. Energy Fuels, 13:390–395.
- Wall, C., Pohl, A., Knapp, M., Hahn, H., and Fichtner, M. (2014). Production of Nanocrystalline Lithium Fluoride by Planetary Ball-Milling. Powder Technol., 264:409–417.
- Williams, P. T. (2005). Waste Treatment and Disposal. (2nd ed.). John Wiley & Sons, Chichester.
- Yang, J., Gebremedhin, A., and Strand, M. (2013). Characterization of Particles and Inorganic Vapors Through High-Temperature Extraction in a Biomass-Fired Grate Boiler. Energy Fuels, 27:5915–5922.
- Yang, J., Lin, L., Morgalla, M., Gebremedhin, A., and Strand, M. (2015). High-Temperature Characterization of Inorganic Particles and Vapors in a Circulating Fluidized Bed Boiler Cofiring Wood and Rubber Waste. Energy Fuels, 29:863–871.