ABSTRACT
Biological warfare incidents generate both immediate and delayed hazards, potentially resulting from reaerosolization of deposited hazardous particles from surfaces. Understanding the causes and effects of the initial deposition method and environmental conditions on reaerosolization is important in hazard prediction and selection of mitigation approaches. This study was conducted to determine the amount of reaerosolization of various bacterial spores and 1 µm polystyrene latex microspheres deposited wet or dry and incubated at 20 or 80% relative humidity (RH). The organisms used in this study were Bacillus atrophaeus var. globigii (Bg), B. thuringiensis (Bt), B. anthracis ΔSterne (Ba-ΔSterne), Ba-ΔSterne ΔbclA mutant (BclA), and Ba-ΔSterne ΔcotE mutant (CotE). These organisms represent a range of spore types with different outer surfaces: spores with exosporium hairs and a basal layer (Ba-ΔSterne and Bt), spores with a basal layer (BclA), and spores with a spore coat only (no exosporium, Bg and CotE). A pulsed air impinging jet was used to reaerosolize particles from gridded glass surfaces. The amount of reaerosolization was determined by counting the number of particles on the gridded surface before and after applying the air jet. Results indicate that, in general, higher reaerosolization was observed when particles were deposited dry and incubated at lower RH conditions. Our results indicate that Bt (has exosporium) was reaerosolized more readily than Bg (no exosporium) in all cases studied. This method can be used in laboratory studies to compare bacterial spore behavior and to study the relative effects of different spore outer layers and surface types on reaerosolization.
© 2017 Leidos, Inc.
EDITOR:
Introduction
Chemical, biological, radiological, nuclear, and explosive (CBRNE) incidents produce both immediate and delayed hazards, the latter a result of reaerosolization of deposited hazardous particles from surfaces. Reaerosolization from outdoor surfaces is commonly caused by natural wind and vibration due to vehicular movement. For biohazard prediction modeling and risk assessment, it is important to quantify the reaerosolization behavior of bacterial spores by air flow under various environmental conditions.
In addition, building ventilation systems are vulnerable to attack by biological agents, so it is important to protect the occupants from delivery of biological warfare agents through the air handlers. Particles will differentially deposit in the ventilation system based on particle size as the aerosol flows through it. Particles may also reaerosolize after the initial deposition. Krauter and Biermann (Citation2007) evaluated reaerosolization of bacterial spores in a heating, ventilating, and air conditioning duct system. More spores reaerosolized from steel surfaces compared to plastic surfaces during the first 5 h, and the differences disappeared after more time. Pulsed flow caused an initial spike in reaerosolization followed by decreased reaerosolization over time (Krauter and Biermann Citation2007).
Reaerosolization of B. anthracis (Ba) spores is a concern because of the long-term environmental stability of spores and their potential use as biological warfare agents. The reaerosolization of Ba-Ames from surfaces in the Hart Senate Office Building has been described in a published report (Weis et al. Citation2002); however, efficient laboratory methods to carefully study reaerosolization of organisms have been lacking. Small-scale wind tunnels only reaerosolize a small percentage of particles, leading to high relative errors in particle count measurements. In addition, investigation of biological agent and simulant correlation behavior requires a method that can quantitatively determine possibly subtle reaerosolization differences among organisms with varying morphologies. Our recently tested robust, efficient experimental reaerosolization method provides more accurate quantification but has not been used until now to study biological organisms (Kesavan et al. Citation2017).
Ba is one of several species of the Gram-positive endospore-forming Bacillus genus of bacteria that has a thin and highly-deformable outer layer known as the exosporium or hairy nap. This is an array of nanometer-scale hair-like glycoprotein filaments that are believed to play a critical role in spore hydrophobicity and adhesion, both in the environment and in vivo (Faille et al. Citation2010; Faille et al. Citation2007; Turnbull Citation2008). Studies have suggested that the flexibility of the exosporium may help increase the contact area between certain spores and surfaces (e.g., B. cereus on stainless steel), thus promoting adhesion compared to inert particles (Bowen et al. Citation2002; Lequette et al. Citation2011).
Particle attachment to surfaces is affected by particle properties (shape, size, material, surface roughness), surface properties (material, roughness, contamination of the surface), duration and method of contact, relative humidity (RH), temperature, initial contact velocity and the mechanism causing the reaerosolization (Hinds Citation1999). The adhesive force between a particle and a surface is due to van der Waals, capillary, and electrostatic forces. When an external force applied to the particle is normal to the surface and is larger than the adhesive force between the particle and the surface, the particle is removed from a surface. Therefore, reaerosolization is the result of competing forces, and the removal forces are as important as adhesion forces for determining particle removal.
Many studies have evaluated the contribution of surface properties to reaerosolization (Goldasteh et al. Citation2012; Mei et al. Citation2016). Adhesive and removal forces depend on particle diameter, with larger particles having more adhesive force with the surface compared to smaller particles; however, larger particles stick up higher into the viscous sublayer of the boundary layer and thus experience higher removal forces (Loosmore Citation2003).
Particles deposited as a layer or clustered together are more easily removed than individual particles. van der Waals forces decrease with increased separation distance between particles and surfaces. In addition, increased surface roughness reduces particle reaerosolization (Hinds Citation1999). Furthermore, higher particle concentration on a surface increases resuspension because detached and rolling particles impact stationary particles, causing reaerosolization of the latter (Ibrahim et al. Citation2004). Biological particles may have more complex surface properties, behaviors, and variability than inert particles so it is critical to conduct such experiments with the particles of interest.
Controlled airflow is routinely used to reaerosolize particles from surfaces to experimentally quantify the percent reaerosolization (EPA-Report Citation2014; Fletcher et al. Citation2008; Keedy et al. Citation2012; Masuda et al. Citation1994; Otani et al. Citation1995; Phares et al. Citation2000a; Citation2000b; Smedley et al. Citation1999). Typically, a high-speed air jet impinging on a solid surface produces a tangential flow with a thin boundary layer that produces a high shear stress at the surface. This shear force overcomes the adhesive force between the particles and the surface, dislodging and removing particles. Two methods have been used in the past to quantify the percent of particles reaerosolized from surfaces: (1) counting the particles on a surface before and after reaerosolization and (2) sampling the gas stream to count the particles removed from the surface. The second method may not detect all the particles that were reaerosolized, underestimating the amount of reaerosolization.
In our earlier study with wet- and dry-deposited 1 to 5 μm polystyrene latex (PSL) microspheres deposited on glass surfaces and incubated in either low (20%) or high (80%) RH conditions for two hours before reaerosolization, we found that dry-deposited particles reaerosolized more than wet-deposited particles with RH held constant. With deposition method held constant, incubation at lower RH produced more reaerosolization compared to the higher RH condition (Kesavan et al. Citation2017).
Our previous publication (Kesavan et al. Citation2017) showed that a pulsed air jet directed perpendicular to a surface containing deposited particles produced the highest reaerosolization 1 mm away from the center of the air impaction on the surface. Both sides of this region have very low reaerosolization, and variability in the placement of the gridded slide under the orifice and the variability in the airflow angle may produce a significant change in the percent reaerosolization. Therefore, to further improve measurement precision, methods that produce less variability should be developed and validated.
The current study was conducted to determine whether reaerosolization amount is affected by dry and wet deposition methods and high (80%) and low (20%) RH conditions for 1 μm PSL microspheres and five different bacterial spores with various outer surface morphologies. A pulsed air jet was utilized to determine the amount of reaerosolization of these particles from gridded surfaces.
Materials and methods
The organisms used in this study were B. atrophaeus var. globigii (Bg), B. thuringiensis (Bt), B. anthracis ΔSterne (Ba-ΔSterne, or simply labeled Ba), Ba-ΔSterne ΔbclA mutant (BclA), and Ba-ΔSterne ΔcotE mutant (CotE). These organisms have various outer layers: spores with basal layer and exosporium hairs (Ba-ΔSterne and Bt), spores with basal layer and no exosporium hairs (BclA), and spores without basal layer and exosporium hairs, i.e., with spore coat only (Bg and CotE). The effect of wet vs. dry deposition and high vs. low RH on the percent reaerosolization was studied. PSL microspheres of comparable size (1 µm) were used as a control.
Bacterial strains
B. atrophaeus (ATCC 9372) and B. thuringiensis (ATCC 10792) were obtained from ATCC, Manassas, VA, USA; Ba-ΔSterne and CotE were obtained from Prof. Adam Driks, Loyola University, Chicago, IL, USA; BclA was obtained from Prof. Alison O'Brien, Uniformed Services University Health Sciences, Bethesda, MD, USA.
Spore preparation
The bacterial strains were streaked onto tryptic soy agar plates and grown overnight at 37°C. An aliquot (0.5 mL/plate) of each strain was then spread over Lab-Lemko plates (15 cm diameter) and incubated at 37°C for seven days or until the sporulation level reached > 95% as determined by microscopic assessment. The spore crop was harvested by dislodging growth on plates using sterile distilled water (25 mL per four plates). The spore crop was filtered through a sterile filter and washed with an additional 25–50 mL of sterile distilled water. Spores were then washed by three successive resuspensions in 25 mL of sterile distilled water and heat-treated at 65°C for 30 min. The extent of sporulation was confirmed microscopically and quantified by dilution plating (Rastogi et al. Citation2009; Sagripanti et al. Citation2007; Smith et al. Citation2011). Spore hardiness was confirmed by heat-treatment and sensitivity to 2.5 M HCl treatment for 1 and 10 min, respectively (Rastogi et al. Citation2009; Sagripanti et al. Citation2007; Smith et al. Citation2011). Spore suspension was held at 4°C in 0.01% sterile Tween-80 solution until use.
Measurement of reaerosolization
Our method of measuring reaerosolization has been described previously (Kesavan et al. Citation2017) and is briefly described here. CELL-VU® hemacytometer glass coverslips with 3 mm × 3 mm laser-etched grid were used for counting particles. The smallest square size was 0.1 mm × 0.1 mm and the largest was 0.5 mm × 0.5 mm. The cover slips were cleaned with soap and water followed by isopropyl alcohol to remove all particles and oils, and air dried before particle deposition.
For wet deposition, a 6” diameter × 9” long Plexiglas® cylinder was used to deposit 1 µm fluorescent PSL microspheres or bacterial spores on the gridded cover slips. An Aeroneb® nebulizer (Aerogen, Galway, Ireland) was used to aerosolize the particles into the cylinder, in which they settled onto the gridded cover slips. PSL microspheres were aerosolized for 1 min, and bacterial spores were aerosolized for the following times: Bg and Bt spores for 5 min and Ba-ΔSterne and mutant (BclA and CotE) spores for 8 min. All bacterial spores had a concentration of approximately 108 CFU per mL. The PSL microsphere suspension obtained from the manufacturer was 1% by solids and was aerosolized without dilution. These different aerosol generation times were chosen to obtain enough bacteria and PSL microspheres on the gridded slides for counting.
For dry deposition, a 6” diameter × 24” long cylinder was used. Microspheres and spores were washed in water and suspended in ethanol and aerosolized separately, using a SonoTek aerosol generator (SonoTek Corporation, Milton, NY, USA) into the cylinder and allowed to settle onto the gridded cover slips. The use of ethanol ensured faster evaporation of the generated droplets. All aqueous suspensions of spores were diluted from 108 CFU/mL to 3.3 × 106 CFU/mL of ethanol before aerosolization in this experiment. Scanning Electron Microscopy (SEM) (JEOL 7001FLV, JEOL, Tokyo, Japan) was used to verify no difference in apparent surface morphology between water- and ethanol-deposited Bg spores. Measurements of spore dimensions from both types of deposition, using SEM at a magnification of 20k, also indicated no significant difference in Bg spore sizes within a 95% confidence interval.
The particles in each grid were individually counted using a microscope (Zeiss Axioskop, Carl Zeiss, New York, NY, USA). Both ultraviolet and visible illumination were used to identify and count the fluorescent PSL microspheres. Low intensity visible light was used to observe the grid while UV light was used to simultaneously observe the fluorescent PSL microspheres. Only visible light was used to count the bacterial spores on the gridded surface.
The time that a particle is attached to the surface is an important factor for reaerosolization (Ibrahim et al. Citation2004; Loosmore Citation2003). The environmental conditioning time of 2 h was selected so that the reaerosolization can be conducted within 24 h of deposition. The aerosol was generated for seeding the previous day and the aerosol deposition on to the gridded slides occurred overnight. The slides were counted the next morning to determine the particle concentration on slides. Following the counting, the slides were conditioned for 2 h in an environmental chamber that maintained the test temperature and RH. At the end of 2 h, the slides were positioned under the pulsed generator for the reaerosolization. The 2 h of incubation time allowed us to complete all these steps within a 24 h period.
shows the experimental apparatus used for removing particles via air impingement. House air supply, which contains a filter system to remove particles from the air, was connected to a solenoid to deliver a single puff of air through an orifice. A pressure of 358.5 kPa between the orifice and solenoid and a puff duration of 0.56 s was used. The distance between the orifice and the surface was maintained at 1.5 cm. Markings on the surface were used to align the cover slide so that the gridded counting region was placed 1 mm off-center under the orifice to avoid the stagnant zone. This 1 mm offset yielded the highest reaerosolization at the measurement location as optimized through our earlier aerosol experiments (Kesavan et al. Citation2017). Particles on cover slips were counted using a microscope before and after the pulsed air jet. A representative scanning electron photomicrograph of PSL microspheres and Bg spores on a gridded cover slip is shown in . Only single spores were counted; doublets, triplets, and larger clusters were not counted. Particles on the grid line were also not counted. Eight replicates of each experiment were performed. A t-test was conducted to determine the statistical differences between the groups.
Results
(left) shows the percent reaerosolization of wet- vs. dry-deposited particles incubated for 2 h at 20% RH. Similarly, (right) shows the percent reaerosolization of wet- vs. dry-deposited particles incubated for 2 h at 80% RH. The statistical differences between groups determined using a t-test are provided in and . Dry deposition of PSL particles at 20% RH produced the most reaerosolization, and this was higher than the wet deposition and 80% RH conditions for PSL and the biological spores. Reaerosolization of PSL under other conditions was closer to that of the bacterial spores. In general, dry-deposited particles were more readily reaerosolized than wet-deposited particles, and the effect of deposition condition (i.e., wet or dry) had a stronger effect on reaerosolization than did humidity. With the exception of the CotE mutant, all particles were more readily reaerosolized when deposited dry vs. wet. In the case of CotE, the deposition method did not seem to have a significant impact on the tendency for reaerosolization at 80% RH, and its impact at 20% RH seemed to barely satisfy the commonly used p < 0.05 significance criteria.
Figure 3. Wet vs. dry deposition effects on average reaerosolization efficiency of 1 μm PSL, Bt, Ba-ΔSterne, BclA, CotE, and Bg at 20% RH (left) and at 80% RH (right); error bars indicate ± 1 standard deviation (n = 8). Statistically significant differences in reaerosolization for the wet and dry deposition are marked on the graph by * (p < 0.05), ** (p < 0.01), and *** (p < 0.001).
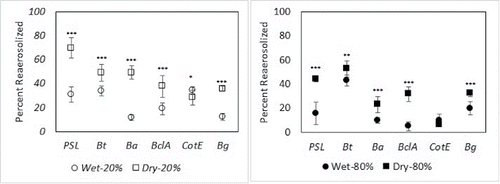
Figure 4. Low vs. high (20% vs. 80%) RH effects on average reaerosolization efficiency of 1 μm PSL, Bt, Ba-ΔSterne, BclA, CotE, and Bg deposited wet (left) and dry (right); error bars indicate ± 1 standard deviation (n = 8). Statistically significant differences in reaerosolization for the high and low RH conditions are marked on the graph by * (p < 0.05), ** (p < 0.01), and *** (p < 0.001).
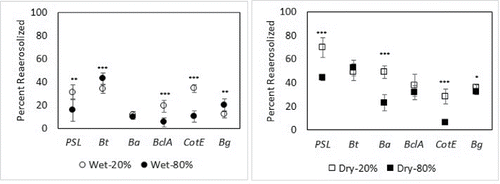
(left) shows the percent reaerosolization of wet-deposited particles incubated for 2 h at 20% vs. 80% RH conditions. Similarly, (right) shows the percent reaerosolization of dry-deposited particles incubated for 2 h at 20% vs. 80% RH conditions. The results indicated that, in general, lower RH conditions produced higher reaerosolization. In most cases, the effect of incubation humidity was smaller than the effect of deposition moisture. Again, the outlier was the CotE mutant, for which the effect of incubation humidity was significant, while there was no appreciable effect of deposition method. Wet-deposited BclA mutant spores incubated at 80% RH resulted in the lowest reaerosolization (strongest adhesion) over all particles and conditions studied.
The outer surfaces of the spores are markedly different and we expected this to impact the tendency for reaerosolization. Our results indicate that Bt (has exosporium) was reaerosolized more readily than Bg (no exosporium, only has spore coat) in all cases studied. For dry deposition with incubation at 20% RH, similar reaerosolization values were obtained for Bt and Ba-ΔSterne; however, Ba-ΔSterne was reaerosolized much less than Bt for other deposition method and RH combinations. There was a clear trend for the dry-deposited particles at low (20%) RH condition: organisms with hair (Bt and Ba-ΔSterne) had more reaerosolization compared to organisms without hair (BclA mutant, CotE mutant, and Bg). BclA mutant (has basal layer but no exosporium hairs) reaerosolized more compared to CotE mutant (no basal layer or hairs, only has spore coat) for both dry deposition (low and high RH) conditions; the opposite trend seemed to occur at both wet deposition (low and high RH) conditions. There were no clear trends observed between Ba-ΔSterne and the mutants at high RH conditions.
Discussion
Spores of Ba-Ames have been documented to be reaerosolized from surfaces by wind and human activities (EPA Report, Citation2014). Specialized facilities and trained personnel are required to conduct tests using biological agents such as Ba-Ames; therefore, simulants such as Bg and Bt are commonly used in BSL 1 and 2 laboratories and outdoor scenarios. Ba-ΔSterne, which is a BSL 2 organism, is used in some laboratory studies since its properties more closely resemble those of Ba-Ames than do other simulants. Subtle differences in various growing and processing methods may affect such studies; therefore, this study was conducted using similarly (consistently) grown and processed organisms to evaluate the percent reaerosolization of various spores maintained at either 20% or 80% RH on glass surfaces. Experiments were also conducted with 1 μm PSL microspheres to compare these results with the spore results. Our results indicate that, in general, higher reaerosolization was observed in dry-deposited particles compared to wet-deposited particles, and lower RH incubation conditions resulted in higher reaerosolization compared to higher RH conditions. Substrate surface effects may also play a strong role in particle adhesion, but comparing results among various surfaces is outside the scope of this study.
To overcome potential challenges in comparing hairy vs. non-hairy spores across different spore species, which may exhibit possible differences in surface properties, mutant Ba-ΔSterne spores with deleted genes (ΔcotE and ΔbclA) were prepared for a more direct exosporium hair vs. no exosporium hair comparison within the same species. Since the CotE mutant spores lack the crystalline basal layer of the exosporium as well as the exosporium hairs, comparing these spores to the BclA mutant spores should provide a direct comparison of basal layer vs. spore coat effects. Bt (hairy) and Bg (non-hairy) spores were also used for comparison and to provide important insights, especially given the routine use of Bg and Bt as simulants for B. anthracis.
In the literature, reaerosolization of Ba-Ames spores and surrogates Bg and Bt from various surfaces has been assessed, and it has been shown that Ba can be reaerosolized from surfaces and Bt can be used as a surrogate for Ba for wet and dry deposition (EPA-Report Citation2014). Our study also evaluated the effect of wet vs. dry deposition on reaerosolization and our results agree with previous results (EPA-Report Citation2014; Layshock et al. Citation2012) and show that more reaerosolization is observed for dry vs. wet deposition. Bt and Ba-ΔSterne, which have the exosporium hair, had higher reaerosolization compared to other organisms at dry deposition and 20% RH conditions. Similar results were reported in the EPA study (2014). BclA mutant does not have exosporium hair and had less reaerosolization compared to Ba-ΔSterne at dry deposition and 20% RH conditions; CotE and Bg do not have the exosporium (no basal layer or hairs) and had even less reaerosolization at dry deposition and 20% RH conditions. This same trend was not seen with wet deposition or higher RH conditions, which have significant capillary forces produced by the water between the spore and the surface; these capillary forces may overwhelm the effect of the exosporium. However, the general trend of higher reaerosolization of Bt vs. Bg was observed in all of the conditions (wet or dry, low or high RH) that we have studied and is in agreement with the results of the EPA Report.
Studies have evaluated the effects of particle and surface types on the amount of adhesive force using atomic force microscopy (AFM). Kweon et al. (Citation2011) studied Bt adhesion to mica, glass, and gold surfaces at various RH conditions (0–80%). RH was observed to play an important role in adhesion because of capillary forces and the influence of water on surface properties. As RH increases, the surface coverage and thickness of the water layer increases, resulting in increased capillary force. On the other hand, electrostatic forces between the particle and the surface are reduced by the moisture. Similar to our study, Kweon et al. (Citation2011) showed increased adhesive force (analogous to less reaerosolization) with increased RH conditions for Bt on a glass surface. Although the electrostatic force can be an important component in spore adhesion to a surface (Chung et al. Citation2010), our study did not attempt to distinguish this contribution from the overall result, due to the complexity of measuring the charge of spores and surfaces under high velocity air flow conditions. Although it is possible to correlate adhesive force between surfaces and particles measured by AFM to reaerosolization measured by air flow studies in a wind tunnel, there are some important differences that make such a comparison challenging and thus require care: reaerosolization in the wind tunnel is related to the force on a particle parallel to the surface, whereas spore-probe AFM typically determines the force normal to the surface. The amount of reaerosolization will depend on the direction of force applied to the spore, and spores attached to a surface are significantly more vulnerable to detachment by hydrodynamic shear or lateral forces than by forces applied in a normal direction (Bowen et al. Citation2002). Therefore an adhesive force measurement method needs to be selected with this in mind.
Our previous test indicated that the highest reaerosolization occurred at 1 mm from the center of the impacting jet (Kesavan et al. Citation2017). The amount of reaerosolization decreases significantly away from this region. Small positioning differences will affect the amount of reaerosolization significantly. Therefore, other methods that produce uniform reaerosolization across a surface should be investigated. In addition, microscopic counting is time consuming and requires a light penetrating surface. Other methods that can quantify the amount of reaerosolization from surfaces such as concrete, leaves, and sand need to be investigated.
Size of the particles affects the adhesive force between the particle and the surface. Bg spores are smaller than Bt and Ba spores (Carrera et al. Citation2007). A Bg spore is 1.22 ± 0.12 µm long (mean) and has a mean diameter of 0.65 ± 0.05 µm. A Bt spore is 1.61 ± 0.18 µm long (mean) and has a mean diameter of 0.80 ± 0.07 µm. Ba-ΔSterne has a length (1.55 ± 0.15 µm) and diameter (0.86 ± 0.07) similar to that of Bt. In addition to the size of the particle, the hydrophobic properties of the spore may also affect the amount of reaerosolization; however the hydrophobic properties of the spores were not determined or analyzed in this study. Bg spores are less hydrophobic than Bt or Ba (Greenberg et al. Citation2010). The smaller size and reduced hydrophobicity of Bg compared to the other spores may have influenced our observation of less Bg reaerosolization while attached to hydrophilic glass. Although Bg has also been observed to reaerosolize less than Bt or Ba on more hydrophobic surfaces such as concrete (EPA-Report Citation2014), future work should study the relative importance of these effects compared to spore surface morphology. Based on our study of Bt, Ba-ΔSterne, and mutants, all of which have very similar sizes yet different reaerosolization behavior depending on conditions, it seems that spore size alone cannot explain the results. Indeed, no single spore parameter will likely be able to adequately explain such diverse phenomena.
Environmental RH conditions and surface properties are known to affect reaerosolization of particles from surfaces (Ibrahim et al. Citation2004; Kassab et al. Citation2013; Kim et al. Citation2016). Higher RH conditions increase water condensation and water contact between a particle and surface, resulting in reduced reaerosolization. A critical RH level, which depends on the particle and surface properties, is required for capillary forces to be observed, and below this RH condition the adhesive force is not affected (Hubbard et al. Citation2012; Kim et al. Citation2016). Hydrophobic particles on hydrophobic surfaces were affected the least by increasing RH conditions; on the other hand, hydrophilic particles on hydrophilic surfaces were affected the most (Kim et al. Citation2016). Our study determined the amount of reaerosolization of spores on glass surfaces which are smoother and more hydrophilic compared to other surfaces such as tile and concrete. The average surface roughness for glass is 6.2 nm and the water contact angle is 10.6° (Kim et al. Citation2016). Studies support the notion that adhesive forces are the major determinant in predicting reaerosolization and have shown that there is less reaerosolization of particles deposited from slurries compared to dry deposition methods (Layshock et al. Citation2012). Our study also confirmed this same finding of less reaerosolization of particles generated from slurries (wet deposition) compared to dry deposition. Our study used a conditioning time of 2 h before reaerosolization. Longer contact time between the particle and the surface will increase the adhesive force and may reduce the amount of reaerosolization. Longer contact time will also increase the amount of water between the particle and the surface in high RH conditions. This will also result in higher adhesive force due to the capillary effects.
Conclusions
In this study, we developed a laboratory reaerosolization method for comparing reaerosolization of various organisms under realistic environmental conditions. Comparative reaerosolization efficiencies (percent) were evaluated for several species of bacterial spores from glass surfaces using a pulsed air jet. The results indicate that, in general, more reaerosolization was observed in dry-deposited particles compared to wet-deposited particles. Also, lower RH incubation conditions produced higher reaerosolization compared to higher RH conditions. Our results indicate that Bt (with exosporium) was reaerosolized more readily than Bg (no exosporium) in all cases studied. There was a clear trend for the dry-deposited particles at low (20%) RH condition: organisms with hair (Bt and Ba-ΔSterne) had more reaerosolization compared to organisms without hair (BclA mutant, CotE mutant, and Bg). BclA mutant (has basal layer but no exosporium hairs) was reaerosolized more compared to CotE mutant (no basal layer or hairs, only has spore coat) for the dry deposition at 20% RH condition. Our test methodology and results may help inform selection of appropriate biological simulants for use in future reaerosolization studies. This study only evaluated reaerosolization from gridded glass slides, so future studies should develop methods to efficiently and accurately determine reaerosolization of biological materials from common indoor and outdoor surfaces under various environmental conditions.
Funding
This research was funded by the Edgewood Chemical Biological Center, Surface Science Initiative.
References
- Bowen, W. R., Fenton, A. S., Lovitt, R. W. and Wright, C. J. (2002). The measurement of Bacillus mycoides spore adhesion using atomic force microscopy, simple counting methods, and a spinning disk technique. Biotechnol. Bioeng 79:170–179.
- Carrera, M., Zandomeni, R. O. and Sagripanti, J. L. (2007). Differences between the spore sizes of Bacillus anthracis and other Bacillus species. J. Appl. Microbiol. 102:303–312.
- Chung, E., Yiacoumi, S., Lee, I. and Tsouris, C. (2010). The role of the electrostatic force in spore adhesion. Environ. Sci. Technol. 44:6209–6214.
- EPA-Report (2014). Determination of the difference in reaerosolization of spores off outdoor materials, United States Environmental Protection Agency. Available for download from https://cfpub.epa.gov/si/si_public_file_download.cfm?p_download_id=520250.
- Faille, C., Sylla, Y., Le Gentil, C., Bénézech, T., Slomianny, C. and Lequette, Y. (2010). Viability and surface properties of spores subjected to a cleaning-in-place procedure: Consequences on their ability to contaminate surfaces of equipment. Food Microbiol. 27:769–776.
- Faille, C., Tauveron, G., Le Gentil-Lelièvre, C. and Slomianny, C. (2007). Occurrence of bacillus cereus spores with a damaged exosporium: Consequences on the spore adhesion on surfaces of food processing lines. J. Food Prot. 70:2346–2353.
- Fletcher, R., Briggs, N., Ferguson, E. and Gillen, G. (2008). Measurement of air jet removal efficiencies of spherical particles from cloth and planar surfaces. Aerosol Sci. Technol. 42:1052–1061.
- Goldasteh, I., Ahmadi, G. and Ferro, A. R. (2012). A model for removal of compact, rough, irregularly shaped particles from surfaces in turbulent flows. J. Adhesion 88:766–786.
- Greenberg, D. L., Busch, J. D., Keim, P. and Wagner, D. M. (2010). Identifying experimental surrogates for Bacillus anthracis spores: a review. Investigative Genetics 1:1–12.
- Hinds, W. C. (1999). Aerosol Technology: Properties, Behavior, and Measurement of Airborne Particles. John Wiley & Sons, Inc., New York.
- Hubbard, J. A., Brockmann, J. E., Rivera, D. and Moore, D. G. (2012). Experimental study of impulse resuspension with laser Doppler vibrometry. Aerosol Sci. Technol. 46:1303–1312.
- Ibrahim, A. H., Dunn, P. F. and Brach, R. M. (2004). Microparticle detachment from surfaces exposed to turbulent air flow: Effects of flow and particle deposition characteristics. J. Aerosol Sci. 35:805–821.
- Kassab, A. S., Ugaz, V. M., King, M. D. and Hassan, Y. A. (2013). High resolution study of micrometer particle detachment on different surfaces. Aerosol Sci. Technol.:351–350.
- Keedy, R., Dengler, E., Ariessohn, P., Novosselov, I. and Aliseda, A. (2012). Removal rates of explosive particles from a surface by impingement of a gas jet. Aerosol Sci. Technol. 46:148–155.
- Kesavan, J., Humphreys, P., Nasr, B., Goodarz, A., Knox, C., Valdes, E., Rastogi, V. K. and Dhaniyala, S. (2017). Experimental and computational study of reaerosolization of 1 to 5 μm PSL microspheres using jet impingement. Aerosol Sci. Technol. 51:377–387.
- Kim, Y., Wellum, G., Mello, K., Strawhecker, K. E., Thoms, R., Giaya, A. and Wyslouzil, B. E. (2016). Effects of relative humidity and particle and surface properties on particle resuspension rates. Aerosol Sci. Technol. 50:339–352.
- Krauter, P. and Biermann, A. (2007). Reaerosolization of fluidized spores in ventilation systems. Appl. Environ. Microbiol. 73:2165–2172.
- Kweon, H., Yiacoumi, S. and Tsouris, C. (2011). Friction and adhesion forces of Bacillus thuringiensis spores on planar surfaces in atmospheric system. Langmuir 27:14975–14981.
- Layshock, J. A., Pearson, B., Crockett, K., Brown, M. J., Cuyk, S. V., Daniel, W. B. and Omberg, K. M. (2012). Reaerosolization of bacillus spp. in outdoor environments: A review of the experimental literature. Biosecurity and Bioterrorism: Biodefense Strategy, Practice, ad Sci. 10:299–303.
- Lequette, Y., Garenaux, E., Tauveron, G., Dumez, S., Perchat, S., Slomianny, C., Lereclus, D., Guerardel, Y. and Faille, C. (2011). Role played by exosporium glycoproteins in the surface properties of Bacillus cereus spores and in their adhesion to stainless steel. Appl. Environ. Microbiol. 77:4905–4911.
- Loosmore, G. A. (2003). Evaluation and development of models for resuspension of aerosols at short times after deposition. Atmos. Environ. 37:639–647.
- Masuda, H., Gotoh, K., Fukada, H. and Banba, Y. (1994). The removal of particles from flat surfaces using a high-speed air jet. Adv. Powder Technol. 5:205–217.
- Mei, X., Zhang, T. and Wang, S. (2016). Experimental investigation of jet-induced resuspension of indoor deposited particles. Aerosol Sci. Technol. 50:230–241.
- Otani, Y., Namiki, N. and Emi, H. (1995). Removal of fine particles from smooth flat surfaces by consecutive pulse air jets. Aerosol Sci. Technol. 23:665–673.
- Phares, D. J., Smedley, G. T. and Flagan, R. C. (2000a). Effect of particle size and material properties on aerodynamic resuspension from surfaces. J. Aerosol Sci. 31:1335–1353.
- Phares, D. J., Smedley, G. T. and Flagan, R. C. (2000b). The wall shear stress produced by the normal impingement of a jet on a flat surface. J. Fluid Mech. 418:351–375.
- Rastogi, V. K., Wallace, L., Smith, L. S., Ryan, S. P. and Martin, B. (2009). Quantitative method to determine sporicidal decontamination of building surfaces by gaseous fumigants, and issues related to laborator-scale studies. Appl. Environ. Microbiol. 75:3688–3694.
- Sagripanti, J. L., Carrera, M., Insalaco, J., Ziemski, M., Rogers, J. and Zandomeni, R. (2007). Virulent spores of Bacillus anthracis and other Bacillus species deposited on solid surfaces have similar sensitivity to chemical decontaminants. J. Appl. Microbiol. 102:11–21.
- Smedley, G. T., Phares, D. J. and Flagan, R. C. (1999). Entrainment of fine particles from surfaces by gas jets impinging at normal incidence. Exp. Fluids 26:324–334.
- Smith, L. S., Wallace, L. and Rastogi, V. K. (2011). Studies on sporulation optimization and characterization of Bacillus subtilis spore quality, Edgewood Chemical Biological Center, U.S. Army Research, Development and Engineering Command, Aberdeen Proving Ground, MD.
- Turnbull, P. (2008). Anthrax in humans and animals, P. Turnbull, ed., World Health Organization, Geneva, Switzerland.
- Weis, C. P., Intrepido, A. J., Miller, A. K., Cowin, P. G., Durno, M. A., Gebhardt, J. S. and Bull, R. (2002). Secondary aerosolization of viable Bacillus anthracis spores in a contaminated US senate office. JAMA 288:2853–2858.