ABSTRACT
The impaction well containing an oil-soaked glass fiber (GF) filter of the well impactor ninety-six (WINS) needs to be replaced every five sampling days to avoid particle overloading which affects the cutoff diameter (dpa50) and the sampling accuracy. This study developed a novel modified WINS (M-WINS) which uses water to wash the wetted GF filter substrate clean continuously to eliminate the particle overloading effect and extend the service life of the impactor without the need of impaction well cleaning and replacement of the oil-soaked GF filter. The laboratory test showed that dpa50 of the M-WINS only varied slightly from 2.44 to 2.49 µm while dpa50 of the WINS decreased from 2.44 to 2.05 µm when the loaded particle mass increased from 0–6 mg. The field test showed that dpa50 and the sampling bias of the novel M-WINS met the USEPA requirements (dpa50 = 2.5 ± 0.2 µm and sampling bias <±5%) while the WINS without regular replacement of the well showed the decrease in dpa50 down to 2.12 µm and the increase in the negative sampling bias to −20% after 17 continuous sampling days. Therefore, the current M-WINS can be used as the PM2.5 inlet for unattended, continuous sampling for a long time with a very good sampling accuracy.
Copyright © 2017 American Association for Aerosol Research
EDITOR:
1. Introduction
Inertia impactors are often used as size-selective inlets in ambient PM10 (particulate matter <10 µm in aerodynamic diameter) and PM2.5 (particulate matter <2.5 µm in aerodynamic diameter) sampling systems (Watson and Chow Citation2011). The performance of inertial impactors is characterized by the cutoff aerodynamic diameter (dpa50), which is the aerodynamic diameter corresponding to 50% collection efficiency, and the sharpness or geometric standard deviation (GSD) of the collection efficiency curve. The GSD is calculated as (Kenny et al. Citation2004):[1] where dpa84 and dpa16 are the aerodynamic diameters corresponding to the collection efficiencies of 84% and 16%, respectively. The most important parameter that governs the collection efficiency is the Stokes number (Stk), which is the ratio of the particle's stopping distance to the characteristic dimension of the nozzle. Jet Reynolds number (Re) is another parameter governing the flow field and the collection efficiency. Inertial impactors will have sharp and nearly the same collection efficiency curves if Re ranges between 500 and 3000 (Marple and Rubow Citation1986). Gravitational force of particles increases the particle collection efficiency when Re is below about 1500 (Huang and Tsai Citation2001). Other parameters influencing the collection efficiency curves are the impaction plate diameter, the particle density (Huang and Tsai Citation2002) and the design of impaction surfaces (Tsai and Cheng Citation1995; Tsai and Lin Citation2000).
The inertial impactor is known to have problems associated with particle overloading and solid particle bounce, which cause the shift of the cutoff diameter and the collection efficiency curve leading to incorrect PM measurement (Pak et al. Citation1992; Tsai and Cheng Citation1995; Kenny et al. Citation2000; Vanderpool et al. Citation2001b). To minimize solid particle bounce, the impaction plate is usually coated with vacuum grease, vacuum or silicone oil (Turner and Hering Citation1987; Pak et al. Citation1992; Tsai et al. Citation2012) or covered by a glass-fiber (GF) filter saturated in water (Dunbar et al. Citation2005). Use of rough impaction plates (Marjamäki and Keskinen Citation2004), porous substrates (Huang et al. Citation2001; Marjamäki and Keskinen Citation2004; Huang et al. Citation2005), or specially designed substrates (Tsai and Cheng Citation1995; Chang et al. Citation1999; Kim et al. Citation2013) also help reduce particle bounce. In addition, the relative humidity of incoming aerosols can be conditioned to a higher value to minimize particle bounce (Dunbar et al. Citation2005; Chen et al. Citation2011).
Solid particle bounce from the grease-coated impaction plate still occurs when the plate is loaded heavily with particles. The incident particles strike on previously deposited solid particles rather than the coated plate resulting in possible particle bounce (Tsai and Cheng Citation1995; Vanderpool et al. Citation2001b). To increase the particle loading capacity on the impaction substrate, the special designs of impaction plates (Tsai and Cheng Citation1995), oil-soaked filters (Peters et al. Citation2001a), oil-coated impaction substrate (Turner and Hering Citation1987; Pak et al. Citation1992; Tsai et al. Citation2012), or rotating substrates (Marple et al. Citation1991; Tsai et al. Citation2012) were used.
The Well Impactor Ninety-Six (WINS), which is a single-jet, round-nozzle inertial impactor with dpa50 of 2.48 µm and GSD of 1.18, consists of an upper housing containing a nozzle and a lower housing supporting an impaction well (Peters et al. Citation2001a; Vanderpool et al. Citation2001a). The well, which contains a 37 mm GF soaked with 1 mL mineral oil as the substrate, is used to minimize particle overloading and subsequent particle bounce-off similar to a previous design (Tsai and Cheng Citation1995). Additionally, the well is also helpful for retaining any blow-off from the tip of the conical build-up that is typical after the collection of a large amount of particles (Peters et al. Citation2001a). The collection efficiency curve of the WINS is not affected by particle gravity as Re equals 6000 (Huang and Tsai Citation2001) and there is no particle loss at the sidewall of the well since Stk50 and the well-to-jet radius ratio equals 0.238 and 9.45, respectively (Hu et al. Citation2007). The WINS is designated as the inlet for an USEPA PM2.5 Federal Reference Method (FRM) sampler or a PM2.5 Federal Equivalent Method (FEM) monitor.
However, previous laboratory studies showed that dpa50 of the WINS shifted downward to 2.15 µm after loading aloxite powder to 4.5 mg (Kenny et al. Citation2000) and to 2.21 µm after loading Arizona test dust (ATD) to 16 mg, corresponding to 5 consecutive sampling days with a negative bias of −5% (Vanderpool et al. Citation2001b). In the filed sampling studies, dpa50 of the WINS was also shown to reduce by 12% (2.44 µm to 2.15 µm) after 4 weeks of sampling at a parking lot, corresponding to the estimated bias in PM2.5 concentrations of −8.8% (Kenny et al. Citation2000), and decrease to 2.32 µm with a maximum bias of −2.1% in PM2.5 concentrations after 120-h of sampling (Vanderpool et al. Citation2001b). This is because that oil wicks up the previously deposited particle layer and accumulates particles to form a mound which reduces the cutoff diameter.
The above studies supported USA Federal regulation that requires the replacement of the WINS impaction well every 5 days of 24-h sampling (USEPA Citation1998; Vanderpool et al. Citation2001b). In comparison, dpa50 of another PM2.5 inlet called Very Sharp Cut Cyclone (VSCC) was not changed and GSD increased only slightly from 1.16 to 1.2 after continuous sampling 90 days at an equivalent concentration of 150 µg/m3 (Kenny et al. Citation2004). This is due to a much more diffused particle deposition region in the VSCC than the single jet deposition area in the WINS. It is important to note that the “equivalent” 90 sampling days at the concentration of 150 µg/m3 was determined in the laboratory for 30 h with the ATD particle concentration of 10 mg/m3.
This study aims to develop a novel modified WINS (M-WINS), which uses water to wash the impaction substrate clean continuously, to extend the 5-day requirement to replace the impaction well of the WINS to a much longer period. The M-WINS uses a wetted GF filter as the substrate through which a 0.8 mm hole is drilled at the center as shown in . Deionized (DI) water flow is injected continuously upward at a flow rate of 0.3 mL/min by using a syringe pump to wash off deposited particles from the substrate. Washed water is drained off by using another syringe pump through a hole drilled at the edge of the well. The impaction surface is slightly inclined downward at 5 degrees from the center to the hole to ease the water flow. The wetted GF filter is used because it can combine the actions of liquid surface tension (capillary forces) and soluble particle dissolution to prevent particle bounce and overloading (Dunbar et al. Citation2005). The GF filter is relatively cheap, has good chemical resistance and dense fiber weave that retains solution effectively (Peters et al. Citation2001a).
In this article, the M-WINS was tested for the collection efficiency and the particle overloading effect in the laboratory. The original WINS was also tested for comparison. After that, two field comparison tests were conducted for 12 and 17 days, respectively, for the performance and particle loading effect of both the M-WINS and the WINS using the VSCC as the main reference.
2. Experimental method
2.1. Laboratory tests
shows the experimental setup for determining the collection efficiency curves of the M-WINS or the WINS. The experimental method is similar to that in detail in the previous study (Peters et al. Citation2001b). Solid ammonium fluorescein particles (AF particles; density is 1.35 g/cm3) with the aerodynamic diameter ranging from 1.5 to 5.0 µm were generated by a Vibrating-Orifice Aerosol Generator (VOAG, TSI model 3450, TSI Inc., Shoreview, MN, USA) (Vanderpool and Rubow Citation1998). The electrostatic charge of particles was neutralized by a neutralizer (Kr-85, TSI model 3054) before particles enter the impactors and/or were measured. The upstream and downstream particle concentrations of the impactors were measured by an Aerodynamic Particle Sizer (APS, TSI model 3321, USA) to calculate the collection efficiency, ηexp (%), as:[2] where Cin and Cout are the upstream and downstream aerosol number concentrations (#/cm3). The collection efficiency for each particle diameter was calculated from at least six upstream and downstream measurements. The APS was calibrated first with four standard PSL particles (polystyrene latex, 1, 2, 3, and 4 µm) before the tests and the accuracy was found to be approximately ±3%. The discrete collection efficiency data were fitted by following equation to obtain dpa50 and GSD (Peters et al. Citation2001a):
[3] where a, b, c, d, and e are parameters. This equation should be fitted with R2 > 0.99.
A spreadsheet was used to perform the numerical integration of the collection efficiency curve of the M-WINS or the WINS with the idealized ambient distributions to estimate PM2.5 mass concentrations (Csamp), which is similar to that in the previous study (Vanderpool et al. Citation2001b). The sampling bias in estimated PM2.5 concentrations of the M-WINS or the WINS and the ideal WINS is calculated by[4] where Cideal (µg/m3) is the estimated PM2.5 mass concentration of the ideal WINS. Cideal equals 13.81, 34.28 and 78.53 µg/m3 corresponding to idealized fine, typical and coarse ambient particle size distributions, respectively (USEPA Citation1998; Vanderpool et al. Citation2001b).
In order to study the particle overloading of the M-WINS or the WINS, Al2O3 powder (QF-Al-500, Sipernat, Japan) was dispersed by using a Small-Scale Powder Disperser (SSPD, TSI model 3433) at the aerosol flow rate of 18.5 L/min. Generated particles were then introduced into the 5 L stainless steel chamber and diluted by 3.2 L/min clean airflow. The upstream particle mass concentration was determined by filter cassette 01 at 5.0 L/min and remaining 16.7 L/min passed through the M-WINS or the WINS. The downstream mass concentration was determined by filter cassette 02 at 5.0 L/min. 47 mm Teflon filters (Teflo R2PL037, Pall Corp., NY, USA) installed in cassette 01 and 02 were weighed before and after sampling after being conditioned for at least 24-h in an environment conditioning room where the relative humidity and temperature were kept at 40 ± 2% and 21 ± 1°C, respectively. The electrostatic charge of the filters was eliminated by an ionizing air blower (Model CSD-0911, MEL-SEI, Japan) before the filters were weighted by a microbalance (Model CP2P-F, Startorius, Germany). The precision of weighting was determined to be 2.0 µg by repeated weighing for at least five times. The total loaded particle mass, ML (mg), in the WINS or the M-WINS was calculated as[5] where Min and Mout are the collected particles mass of filter cassette 01 and 02, respectively (mg).
Two syringe pumps were used to keep the impaction substrate of the M-WINS clean during the particle loading test at the water flow rate of 0.3 mL/min. After preliminary tests, the water flow rate was set at 0.3 mL/min to not only wash off the deposited particles effectively but also minimize the amount of water used. After each loaded particle mass of 0.5, 4.0 and 6.0 mg, the M-WINS or the WINS was tested for the particle collection efficiency curve immediately by using the APS.
2.2. Field comparison tests
The field comparison tests were conducted at National Chiao Tung University (NCTU) from Oct. 29 to Nov. 10, 2016 (period #1, 12 days) and from Nov. 15 to Dec. 3, 2016 (period #2, 17 days). The NCTU sampling site was located at the rooftop of the five-floor building of the Institute of Environmental Engineering of NCTU, which is about 1 km away from heavy-traffic roads. Samplers were set up at a sampling platform with 2-meter height inlet tubes.
One Dichot (Dichotomous PM2.5 sampler, Model 241, Andersen Inc., Georgia, USA) equipped with the M-WINS and the other Dichot equipped with the WINS were collocated with a Partisol®-FRM 2000 sampler containing the VSCC and a TEOM-FDMS (tapered element oscillating microbalance with a filter dynamic measurement systems, Model 1405-DF) as the reference samplers. Only the base-mode PM2.5 data of the TEOM-FDMS were used for comparison without correcting for the reference-mode PM2.5. The M-WINS or the WINS was used to replace the original virtual impactor in the Dichot so that only PM2.5 was sampled. A 47 mm Teflon filter (Teflo R2PL047, Pall Corp.) was used in the Partisol-VSCC and two 37 mm Teflon filters (Teflo R2PL037, Pall Corp.) were used in the Dichot-M-WINS and the Dichot-WINS, respectively. The filter conditioning, pre-weighting, and post-weighting procedures were similar to those described in the previous section.
In period #1, the WINS and the VSCC were cleaned manually every day (i.e., clean inlets) to evaluate the performance of the M-WINS. In period #2, the VSCC was cleaned while the WINS was not cleaned during the sampling period to examine the particle loading effect of both the WINS and M-WINS (i.e., uncleaned inlets). In both periods, the substrate of the M-WINS was cleaned automatically by injecting water at the flow rate of 0.3 mL/min. After the test of period #2 was over, the impaction wells of the M-WINS and the WINS were tested for the cutoff diameter in the laboratory following the method described in the previous section.
In order to evaluate the sampling precision of M-WINS or the WINS, paired sample t tests were conducted using SPSS (Statistical Package for the Social Sciences, version 22.0, SPSS Inc., Chicago, IL, USA) to determine whether two PM2.5 data sets measured by two collocated pairs of samplers are statically the same (p > 0.05).
3. Results and discussion
3.1. Laboratory results—particle collection efficiency
shows the particle collection efficiency curve of the WINS. The cutoff diameter equals 2.44 ± 0.05 µm, which is very close to the cutoff diameters of 2.48 ± 0.05 µm by Peters et al. (Citation2001a) and 2.44 ± 0.03 µm by Kenny et al. (Citation2000). The GSD of the collection efficiency curve of the WINS is 1.2, indicating that the particle collection efficiency curve is less sharp than that in Peters et al. (Citation2001a) (GSD = 1.18) but sharper than that in Kenny et al. (Citation2000) (GSD = 1.23). The particle collection efficiencies of the coarse particles (2.5 to 4 µm) are lower than those obtained by Peters et al. (Citation2001a) while the particle collection efficiencies of the fine particles are slightly lower than those obtained by Kenny et al. (Citation2000). The difference in GSDs between the present study and that of Peters et al. (Citation2001a) is mainly due to more data points acquired for coarse particles and hence better data fitting in the current study, although the same kind of test particles (monodisperse AF particles) were used. The test particles used in Kenny et al. (Citation2000) were solid, polydisperse spherical glass microspheres with the number median diameter around 1.5 µm. These different types of solid particles may create the differences in the GSDs. Despite the small differences in the cutoff diameters and GSDs, the estimated biases of the WINS in the present study are less than ±1.1%, which still meet the criteria specified in the USEPA FRM regulation (<±5%) (USEPA Citation2001).
shows the collection efficiency curve of the M-WINS with the wetted GF filter substrate. The cutoff diameter of the M-WINS equals 2.46 ± 0.05 µm, which agrees with the theoretical cutoff diameter of 2.4 µm (Marple and Willeke Citation1976; Peters et al. Citation2001a), is similar to that of the WINS and meets the criteria specified in the USEPA FRM regulation (2.5 ± 0.2 µm) (USEPA Citation2001). The dimensions of the nozzle, which are not changed in the M-WINS, were designed to achieve dpa50 of ∼2.5 µm (Peters et al. Citation2001a). The GSD of the collection efficiency curve of the M-WINS is 1.24, indicating that the collection efficiency curve of the M-WINS is slightly less sharp than that of the WINS when using water-saturated GF filter instead of oil-soaked GF filter, especially in the particle diameter range of 2.5 to 4 µm. This is because the particle collection characteristics of the impactor is notably influenced by the nature of impaction surface (Marjamäki and Keskinen Citation2004). In this study, the silicon oil (KF96SP, Shin-Etsu Chemical Co. Ltd., Taiwan) with the kinetic viscosity of 100 mm2/s used in the WINS could retain particles on the impaction substrate more effectively as compared to water with a lower kinetic viscosity of 0.89 mm2/s used in the M-WINS.
In , it is apparent that the M-WINS with a dry impaction plate without adhesive coating has low particle collection efficiency for coarse particles due to particle bounce. It is observed that particles deposit in the inner wall and some are trapped in the antispill ring of the impaction well after bouncing from the plate. This particle loss is considered a part of the collection efficiency determined by the APS, which explains the steady-state collection efficiency of 80% for particles larger than 4.0 µm. When the plate is wetted by water, solid particle bounce is suppressed, dpa50 reduces to 2.5 µm which still satisfies the acceptance criteria of the USEPA. However, GSD increases to 1.37 indicating a lower coarse particle collection efficiency and less sharp efficiency curve compared to that of the WINS with the wetted GF filter substrate (). Since Re of the M-WINS is 6000, a large fraction of the particles will impact on the substrate within a spot whose radius equals to the nozzle radius (Sethi and John Citation1993; Huang and Tsai Citation2002). With the GF filter substrate, injected water could trap and wash off deposited particles effectively. In comparison, part of the injected water can be blown away without trapping particles. This explains a lower collection efficiency of the wetted plate than that of the wetted GF filter substrate. Therefore, the wetted GF filter in the M-WINS improves the collection efficiency of coarse particles over the wet impaction plate since it makes the impaction surface rough and the filter media retains water keeping it from being blown off by the aerosol jet. This tells why the wetted GF filter has to be used in the M-WINS.
The sampling biases of the M-WINS with the wetted GF filter substrate are shown in . There is a small positive bias of +2.25% for the idealized coarse distribution, and small negative biases of −0.58% and −0.15% for the idealized fine and idealized typical distributions, respectively. That is, the M-WINS can be used as the PM2.5 inlet to measure the PM2.5 mass concentration as it meets the performance FRM requirements (dpa50 = 2.5 ± 0.2 µm and sampling bias <±5%).
Table 1. Estimated bias of the M-WINS in PM2.5 concentrations for three ambient aerosol size distributions.
Besides, it was observed that AF particles, which are water soluble, were dissolved completely in DI water after striking on the substrate, indicating that washed water could be further analyzed for determining the chemical constituents for soluble coarse particles.
3.2. Laboratory results—particle loading effect
The laboratory test results of the cutoff diameters and the collection efficiency curves of the WINS at different loaded particle masses are shown in and , respectively. It can be seen that dpa50 of the WINS decreases from 2.44 to 2.05 µm and the collection efficiency curve shifts to the left as the loaded particle mass increases from 0.0 to 6.0 mg. When striking on the oil-soaked GF substrate, the deposited particles retain and pile up on the substrate, resulting in the change of the flow field in the impaction region and the increase in a likelihood of particle collection. Thereby the cutoff diameter decreases and the collection efficiency curve shifts to the left.
Figure 5. (a) Cutoff aerodynamic diameter and (b) collection efficiency curve of the loaded WINS. (Note: the data of Vanderpool et al. (Citation2001b) at loaded particle mass of 16 mg are not shown.)
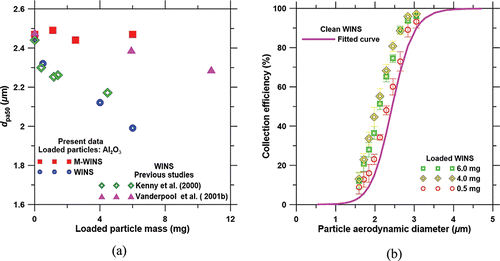
In , the trend of decreasing cutoff diameter is similar to the results in previous studies (Kenny et al. Citation2000; Vanderpool et al. Citation2001b) but the decrease in dpa50 is more significant with increasing loaded particle mass in this study. This is because the loaded particles (Al2O3 powder: 0–4 µm) with the Mass Median Aerodynamic Diameter (MMAD) of ∼2 µm and GSD of ∼1.93 are smaller than those in Kenny et al. (Citation2000) (Aloxite, MMAD = 6 µm and GSD = 1.4) and Vanderpool et al. (Citation2001b) (ARD, MMAD = 5 µm and GSD = 3). Previous studies also found that smaller loaded particles cause greater overloading effect and more shift in dpa50 than larger particles at the same loaded mass (Chen and Huang Citation1998; Kenny et al. Citation2000). Smaller loaded particles form a steady mound underneath the nozzle, which can grow gradually while bigger loaded particles form an unsteady mound, which can be broken off. The type of loaded particles also contributes to the differences in in different studies of the WINS (Chen and Huang Citation1998).
In , a slight decrease in the sharpness of collection efficiency curve (i.e., GSD slightly increases from 1.2 to 1.25) was observed as the loaded particle mass increases from 0.0 to 6.0 mg since the increase in the collection of particles smaller than the cutoff diameter occurs. It is due to the formation of the mound which enhances the collection efficiency of fine particles (Jurcik and Wang Citation1995). The consistent relationship of the decrease in the sharpness and the cutoff diameter is similar to that in Vanderpool et al. (Citation2001b) and Kenny et al. (Citation2000). The estimated negative biases of the loaded WINS increase (−1.1% to −6.9%) with the increase in loaded mass (0.0–6.0 mg), indicating that particle overloading causes the underestimation in PM2.5 mass concentrations. When the loaded mass is greater than 4 mg, the estimated bias of the loaded WINS exceeds the FRM requirement of ±5%. For comparison, Kenny et al. (Citation2000) estimated the mass loading for the WINS performance to deteriorate significantly is less than 3 mg.
In contrast, dpa50 of the M-WINS only varies slightly from 2.44 to 2.49 µm with the loaded particle mass ranging from 0 to 6 mg. That is, the cutoff diameter of the M-WINS is not affected by the loaded particle mass. No particle mound was observed at the center of the impaction substrate in the laboratory study and in the field study to be discussed in the following section. It was observed that the Al2O3 test particles, which are indissoluble, were washed off by continuous injected water preventing the accumulation of the deposited particles. The pictures of the substrates with the mound (WINS) and without the mound (M-WINS) taken after 17 sampling days can be seen in for comparison. That is, it is demonstrated that the M-WINS is able to eliminate the particle loading effect on the cutoff characteristics under a high loading condition.
3.3. Field comparison results
shows field comparison results for the M-WINS and the clean WINS with the VSCC () and with the TEOM-FDMS () as the references at the NCTU sampling site. The results of paired sample t test show that the PM2.5 concentrations of the M-WINS ranging from 3.7 to 34.1 µg/m3 agree well with those of the VSCC (period #1: p = 0.142 > 0.05; period #2: p = 0.886 > 0.05) and the TEOM-FDMS (period #1: p = 0.220 > 0.05; period #2: p = 0.413 > 0.05). Similarly, the PM2.5 concentrations of the clean WINS ranging from 7.9 to 34.8 µg/m3 have no significant difference with those of the clean VSCC (period #1: p = 0.058 > 0.05) and the TEOM-FDMS (period #1: p = 0.059 > 0.05). This indicates that the Dichot-M-WINS is not statistically different from the other samplers for PM2.5 sampling over a wide concentration range.
Figure 6. Field comparison of clean WINS and M-WINS (a) with clean VSCC and (b) with TEOM-FDMS as the references.
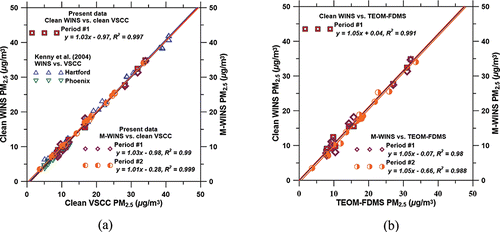
The slope, intercept, and correlation coefficient (R2) of linear regression lines of pairwise comparisons are shown in . It shows a good comparability between the performance of the M-WINS and that of the VSCC (R2 > 0.99), and the TEOM-FDMS (R2 > 0.98). The data of the WINS correlates well with those of the VSCC (R2 > 0.997) and the TEOM-FDMS (R2 > 0.991). The WINS data show a better linear fit to the reference data with higher R2 values than those of the M-WINS, indicating that the WINS outperforms the M-WINS. This is because the sampling effectiveness (dpa50 and GSD) of the WINS is quite similar to the VSCC. Besides, the linear regression coefficients of the M-WINS and the references meet the USEPA performance requirements for comparability of PM2.5 candidate equivalent method samplers (i.e., slope = 1.0 ± 0.05, intercept = 0.0 ± 1.0 µg/m3, and R2 > 0.97) (Kenny et al. Citation2004).
shows that the uncleaned WINS under-samples PM2.5 concentrations compared to the VSCC with the sampling biases increasing from −3.0 to −5.7% (day 1 to day 5), −9.0 to −17.1% (day 6 to day 11, if the data at day 9 and day 11 with the sampling biases of ∼30% were disregarded), and −15.7 to −21.3% (day 12 to day 17). Because of low PM2.5 concentrations (<11.0 μg/m3) at day 9 and day 11, biases become high. During period #2, the PM2.5/PM10 ratio is 0.62 ± 0.19 and the measured PM2.5 concentration range of the VSCC is 3.7–33.5 µg/m3. The deposited particle mound was observed on the WINS substrate as shown in at the end of sampling. The cutoff diameter of the uncleaned WINS shifts downward from 2.48 to 2.12 µm as the estimated loaded mass is increased to 4.1 mg and the total sampled PM2.5 is calculated to be 5.8 mg after 17 sampling days.
The trend is similar to the results in previous studies as described in the introduction (Kenny et al. Citation2000; Vanderpool et al. Citation2001b). However, the present sampling bias of the uncleaned WINS (<−20%) is higher than the estimated sampling bias by Kenny et al. (Citation2000) (<−8.8%) in which the similar total sampled PM2.5 (∼6 mg) and PM2.5/PM10 ratios (0.5–0.75) were reported. The present sampling bias of the uncleaned WINS is −19.6% as the calculated loaded mass is 2.3 mg after 12 continuous sampling days but a small estimated sampling bias of < −1.8% was reported by Vanderpool et al. (Citation2001b) at the same estimated loaded mass (∼2.3 mg) and PM2.5/PM10 ratio (0.62). In addition, after 5 days of sampling, the sampled PM2.5 concentration of the uncleaned WINS is ∼6% lower than that of the clean VSCC, which is beyond the acceptance criteria of the estimated PM2.5 concentration (sampling bias <±5%). This agrees well with USA Federal regulation that requires replacing the well of the WINS every 5 days of 24-hr sampling.
The sampling biases of the novel M-WINS are <±3.6% when PM2.5 concentration is greater than 7.1 µg/m3 (day 1 to 8 and 11 to 17). The biases are increased slightly to −6.4% when PM2.5 concentration is low: 3.7µg/m3 at day 9 and 4.6 µg/m3 at day 10. These results are still in good agreement with the results of the previous studies which found that the sampling biases of PM2.5 FRM could be 2–6% (Peters et al. Citation2001c) or ∼10% (Chow et al. Citation2008) for determining the mass. The cutoff diameter of the M-WINS only shifts from 2.46 to 2.51 µm, which not only meets the accuracy of dpa50 ± 0.07 (Kenny et al. Citation2000) but also falls well in the range of the USEPA requirement of 2.5 ± 0.2 µm. The water injected through the wetted GF filter of the M-WINS not only washes off the deposited particles but can also dissolves most of the inorganic species dominant at the NCTU sampling site. Black color was seen at the central spot on the substrate due to the deposition of un-dissolvable particles such as soot, which were not washed off completely. However, no particles piled up on the substrate were observed after 17 continuous sampling days as shown in .
4. Conclusion
The novel M-WINS using the wetted GF filter as the substrate was developed in this study. In the M-WINS, the injected water captures and washes off the deposited particles on the wetted GF filter continuously, preventing the particle overloading effect. The laboratory tests showed no shift in the cutoff diameter with the loaded particle mass ranging from 0.0 to 6.0 mg and the field comparison study showed accurate PM2.5 concentration measurement during 17 continuous sampling days. The study demonstrates that the M-WINS has a great potential to be used as the PM2.5 inlet for continuous PM2.5 sampling and monitoring for a long period with the sampling bias less than the USEPA requirement of ±5%. In the future, the collected liquid sample can also be coupled with an Ion Chromatograph for the real time measurement of water-soluble ions in coarse particles.
Funding
The final support of this work by the Taiwan Ministry of Science and Technology via the contracts MOST 105-2622-8-009-007-TE4 and MOST 105-2221-E-009-005-MY3 is gratefully acknowledged.
References
- Chang, M., Kim, S., Sioutas, C. (1999). Experimental Studies on Particle Impaction and Bounce: Effects of Substrate Design and Material. Atmos. Environ., 33:2313–2322.
- Chen, C. C., Huang, S. H. (1998). Shift of Aerosol Penetration in Respirable Cyclone Samplers. Am. Ind. Hyg. Assoc. J., 60:720–730.
- Chen, S. C., Tsai, C. J., Chen, H. D., Huang, C. Y., Roam, G. D. (2011). The Influence of Relative Humidity on Nanoparticle Concentration and Particle Mass Distribution Measurements by the MOUDI. Aerosol Sci. Technol., 45:596–603.
- Chow, J., Doraiswamy, P., Watson, J., Chen, L., Ho, S., Sodeman, D. (2008). Advances in Integrated and Continuous Measurements for Particle Mass and Chemical Composition. J Air Waste Manag. Assoc., 58:141–163.
- Dunbar, C., Kataya, A., Tiangbe, T. (2005). Reducing Bounce Effects in the Andersen Cascade Impactor. Inter. J. Pharmaceutics., 301:25–32.
- Hu, S., Seshadri, S., McFarland, A. R. (2007). CFD Study on Compound Impaction in a Jet-in-Well Impactor. Aerosol Sci. Technol., 41:1102–1109.
- Huang, C. H., Tsai, C. J. (2001). Effect of Gravity on Particle Collection Efficiency of Inertial Impactors. J. Aerosol Sci., 35:375–387.
- Huang, C. H., Tsai, C. J. (2002). Influence of Impaction Plate Diameter and Particle Density on Collection Efficiency of Round-Nozzle Inertial Impactors. Aerosol Sci. Technol., 36:714–720.
- Huang, C. H., Tsai, C. J., Shih, T. S. (2001). Particle Collection Efficiency of an Impactor with Porous Metal Substrates. J. Aerosol Sci., 32:1035–1044.
- Huang, C. H., Chang, C. S., Chang, S. H., Tsai, C. J., Shih, T. S., Tang, D. T. (2005). Use of Porous Foam as the Substrate of an Impactor for Respirable Aerosol Sampling. J. Aerosol Sci., 36:1373–1386.
- Jurcik, B., Wang, H. (1995). On the Shape of Impactor Efficiency Curve. J Aerosol Sci., 26:1139–1147.
- Kenny, L. C., Gussman, R., Meyer, M. (2000). Development of a Sharp-cut Cyclone for Ambient Aerosol Monitoring Applications. Aerosol Sci. Technol., 32:338–358.
- Kenny, L. C., Merrifield, T., Mark, D., Gussman, R., Thorpe, A. (2004). The Development and Designation Testing of a New USEPA-Approved Fine Particle Inlet: A Study of the USEPA Designation Process. Aerosol Sci. Technol., 38(S2):15–22.
- Kim, W. G., Yook, S. J., Ahn, K. H. (2013). Collection Efficiency of Rectangular Slit-Nozzle Inertial Impactors with Impaction Plates of Elliptical Concave Curvature. Aerosol Sci. Technol., 47:99–105.
- Marjamäki, M., Keskinen, J. (2004). Effect of Impaction Plate Roughness and Porosity on Collection Efficiency. J. Aerosol Sci., 35:301–308.
- Marple, V. A., Willeke, K. (1976). Impactor Design. Atmos. Environ., 10:891–896.
- Marple, V. A., and Rubow, K. L. (1986). Theory and Design Guidelines, in Cascade Impactor, P. J. Lodge, T. L. Chan, and T. L. Chan Jr, eds., Am. Ind. Hyg. Assoc., Ohio, pp. 79–101.
- Marple, V. A., Rubow, K. L., Behm, S. M. (1991). A Microorifice Uniform Deposit Impactor (MOUDI): Description, Calibration and Use. Aerosol Sci. Technol., 14:434–446.
- Pak, S. S., Liu, B. Y. H., Rubow, K. L. (1992). Effect of Coating Thickness on Particle Bounce in Inertial Impactors. Aerosol Sci. Technol., 16:141–150.
- Peters, T. M., Vanderpool, R. W., Wiener, R. W. (2001a). Design and Calibration of the EPA PM2.5 Well Impactor Ninety-Six (WINS). Aerosol Sci. Technol., 34:389–397.
- Peters, T. M., Vanderpool, R. W., Wiener, R. W. (2001b). Methodology for Measuring PM2.5 Separator Characteristics Using an Aerosizer. Aerosol Sci. Technol., 34:398–406.
- Peters, T., Norris, G., Vanderpool, R., Gemmill, D., Wiener, R., Murdoch, R., McElroy, F., Pitchford, M. (2001c). Field Performance of PM2.5 Federal Reference Method Samplers. Aerosol Sci. Technol., 34:433–447.
- Sethi, V., John, W. (1993). Particle Impaction Patterns from A Circular Jet. Aerosol Sci. Technol., 18:1–10.
- Tsai, C. J., Cheng, Y. H. (1995). Solid Particle Collection Characteristics on Impaction Surfaces of Different Designs. Aerosol Sci. Technol., 23:96–106.
- Tsai, C. J., Lin, T. I. (2000). Particle Collection Efficiency of Different Impactor Designs. Sep. Sci. Technol., 35(16):2639–2650.
- Tsai, C. J., Liu, C. N., Hung, S. M., Chen, S. C., Uang, S. N., Cheng, Y. S., Zhou, Y. (2012). Novel Active Personal Nanoparticle Sampler for the Exposure Assessment of Nanoparticles in Workplaces. Environ. Sci. Technol., 46:4546–4552.
- Turner, J. R., Hering, S. V. (1987). Greased and Oiled Substrates as Bounce-free Impaction Surfaces. J. Aerosol Sci., 18:215–224.
- USEPA. (1998). Monitoring PM2.5 in Ambient Air Using Designated Reference or Class I Equivalent Methods, in Quality Assurance Handbook for Air Pollution Measurement Systems, Volume II, Part II, Section 2.12. Office of Air Quality Planning and Standards, Air Quality Assessment Division, RTP, NC27711.
- USEPA. (2001). Ambient Air Monitoring Reference and Equivalent Methods. Federal Register 40CFR Parts 53, Subpt. F, Table F-1.
- Vanderpool, R. W., Rubow, K. L. (1998). Generation of Large, Solid, Monodisperse Calibration Aerosols. Aerosol Sci. Technol., 9:65–69.
- Vanderpool, R. W., Peters, T. M., Natarajan, S., Tolocka, D. B., Gemmill, D. B., Wiener, R. W. (2001a). Sensitivity Analysis of the USEPA WINS PM2.5 Separator. Aerosol Sci. Technol., 34:465–476.
- Vanderpool, R. W, Peters, T. M, Natarajan, S., Gemmill, D. B., Wiener, R. W. (2001b). Evaluation of the Loading Characteristics of the EPA WINS PM2.5 Separator. Aerosol Sci. Technol., 34:444–456.
- Watson, J. G., Chow, J. C. (2011). Ambient aerosol sampling, in Aerosol Measurement: Principles, Techniques and Applications, P. Kulkarni, P. A. Baron, and K. Willeke, eds., John Wiley & Sons, Inc., New Jersey, pp. 821–844.