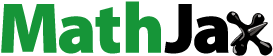
ABSTRACT
A very compact cascade impactor with 2 L/min sampling flow rate has been developed. Its dimensions are 8.5 cm L x 5.0 cm W x 11.4 cm H, and it weighs 0.27 kg, with ten impaction stages with aerodynamic cutpoints in the range of 60 nm to 9.6 μm. The top eight stages, collecting particles down to 170 nm in aerodynamic diameter, can be used as a stand-alone impactor with a portable, battery-powered pump. Particle collection efficiencies were obtained with two types of commonly used substrates, aluminum foil and glass fiber filters. Impactor cutpoints with aluminum foil substrates agree well with conventional impactor theory. The efficiency curves are sharp with minimum overlap between them. Thus, the compact impactor design does not compromise its performance, making it suitable for general purpose applications where a lower sampling flow rate provides adequate mass collection. With glass fiber filter substrates, impactor cutpoints are smaller and the efficiency curves are less steep, in particular for the last stages. Also, the collection efficiency curves do not drop to near zero at small Stokes numbers. Instead, excess particle collection efficiency of around 10% is observed for the top six stages, and becomes higher for the last four stages. This is due to the collection of particles by filtration as the impinging jets penetrate the filter substrate. Thus, using glass fiber filter substrates should generally be avoided due to the non-ideal effect on the impactor collection efficiency curves, especially for the last two stages.
Copyright © 2018 American Association for Aerosol Research
EDITOR:
Introduction
Impactors are simple mechanical devices designed to collect particles by their inertia. Cascade impactors are widely used for collecting size-fractionated samples of aerosols. The Micro-Orifice Uniform Deposit Impactor (MOUDI™) is a well-known cascade impactor initially designed by Marple et al. (Citation1986, Citation1991). This impactor has been significantly improved since its initial development. Recent versions of this impactor include the MOUDI-II™ (Marple et al. Citation2014) and the QCM real-time MOUDI (Chen et al. Citation2016), which work respectively at 30 LPM and 10 LPM inlet flow rate, both with a total pressure drop of 40 kPa. MOUDI impactors have been widely used in characterizing the aerosols from atmospheric environments (Mason et al. Citation2015), industrial processes (Csavina et al. Citation2014), and from radioactive dispersion devices (Di Lemma et al. Citation2014). Their sizable designs and considerable weights, on the other hand, limit their use in some applications like occupational personal exposure monitoring.
The understanding of the adverse effect of aerosol particles on public health emphasizes the importance of personal monitoring (Pöschl Citation2005). Recent development of personal monitors has largely benefited from the improvement of the sensor technology, allowing researchers to investigate a wide range of pollutants other than particles (Koehler and Peters Citation2015; Asbach et al. Citation2017). On the other hand, personal samplers, defined as devices that collect particles on substrates, although cannot provide real-time readings like the monitors, have the advantage of allowing the users to obtain detailed offline analysis. Several commercialized personal samplers are reviewed by Asbach et al. (Citation2017). These samplers, based on electrostatic precipitation (Miller et al. Citation2010), cyclonic separation (Cena et al. Citation2011), and thermal precipitation (Azong-Wara et al. Citation2013), collect particles in a single size channel for subsequent analysis. For more size segregated information on personal exposure to aerosol particles, some previous work has been done on personal cascade impactors (Martonen et al. Citation1982; Rubow et al. Citation1987; Demokritou et al. Citation2002; Misra et al. Citation2002). Constrained by the compact size and light weight required for personal samplers, these cascade impactors typically have low size resolution and cover particle sizes larger than a few hundred nanometers.
The cascade impactor described in this work is specifically designed to fill this gap in performance, by providing higher size resolution and measuring a wider size range. Similar to the regular MOUDI, its ten stages cover the wide particle aerodynamic diameter range of 60 nm to 9.6 μm. Its compact design and low sampling flow rate of 2 L/min are advantageous for some specific applications. The first eight stages have a low pressure drop, and can be operated as a personal impactor with a small, battery-powered personal sampling pump. Therefore, this impactor is suitable for industrial hygiene applications that require wearable samplers that can directly measure the individual exposure to hazardous aerosols. The 10-stage impactor, although it needs a small vacuum pump, can be used for the measurement of aerosols in industrial settings where higher particle concentrations are expected.
In this article, the design and experimental calibration of this cascade impactor are presented and discussed. The efficiency curve and the corresponding cutpoint of each stage were measured experimentally for two commonly used impaction substrates, aluminum foils and glass fiber filters. The issue of solid particle bounce of this impactor was not explicitly investigated. Nevertheless, since this impactor has the same nominal jet velocities as those in a conventional MOUDI impactor, the conditions for particle bounce are expected to be similar to those in the MOUDI, which has been extensively studied (Stein et al. Citation1994; Chen et al. Citation2011).
Impactor design and experimental calibration
The diagram of the inlet, two impaction stages, and the base is shown in . (This figure is for illustration purposes only.) Each stage consists of an impaction surface (11.4 mm in diameter) and a small multi-nozzle plate section. Merging the impaction surface and the nozzle plate into one part makes the impactor shorter and lighter than a conventional impactor design. All the nozzles are round and are distributed within a diameter of 6.4 mm to ensure the particles are collected on the impaction substrates below the nozzles. Aluminum rings are used to hold the substrates in place, so that the new impactor can be operated in typical worker motion conditions regardless of its orientation. One O-ring is used on each stage to seal it with the stage above. In the base, there is a stainless steel support screen for a 13-mm diameter final filter. All the particles smaller than the cutpoint of the last stage are collected by this filter. Two long screws on the sides of the impactor are used to hold all the stages together by tightening two thumb nuts near the inlet of the impactor. shows a picture of a fully assembled 10-stage low flow impactor.
Figure 1. Schematic diagram of the inlet, two stages, and the impactor base. The dashed lines illustrate the sampled aerosol flow path.
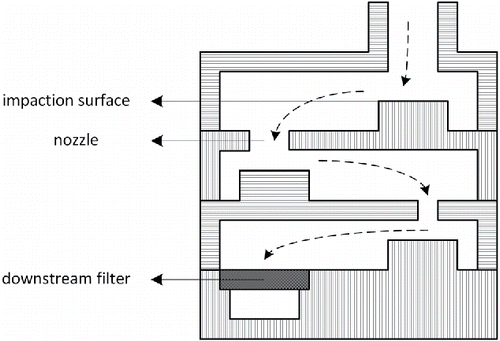
Figure 2. Picture of 10-stage low flow personal cascade impactor. The height is 11.4 cm. The weight is 0.27 kg.
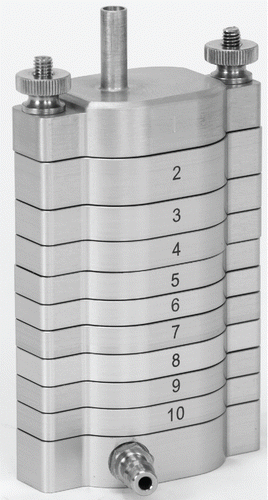
The new impactor design parameters and the summary of the calibration results with aluminum foil substrates are listed in . The impactor was designed to have the same cutpoints as the MOUDI (Marple et al. Citation1991). For the 2 L/min sampling flow rate, the pressure drop is very small for all but the last two stages. As a result, stages 1 to 8 can be used in any combination desired, but stages 9 and 10 need to be used with the other eight stages to bring the pressure to the typical operating value at which the calibration was performed.
Table 1. Design and operating parameters of the new low flow cascade impactor.
The new cascade impactor was calibrated with similar experimental methods as those described by Chen et al. (Citation2016). Two different techniques were used. The top five stages (the inlet is considered as stage 1) were calibrated with monodisperse oleic acid particles tagged with uranine (0.8 μm to 11.8 μm in diameter) generated by a flow-focusing monodisperse aerosol generator (Duan et al. Citation2016) combined with a single stage impactor to remove doublets. The collection efficiency curves of these stages were determined using the fluorometric method by calculating the mass fraction of the uranine collected on the substrate at each particle size. The detailed experimental procedure can be found in Chen et al. (Citation2016). For the lower stages (i.e., stages 6 to 10), an atomizer, a differential mobility analyzer (DMA) and a single stage impactor were used to generate monodisperse liquid DEHS (Di (2-ethylhexyl) sebacate) particles as the calibration aerosol.
shows the experimental setup for calibrating stages 6 to 10. Impactor 1 ran at about 2.1 LPM flow rate, and was selected to have a cutpoint slightly smaller than the diameter of the doubly charged particles existing the DMA. It was used to remove the small fraction of larger multiply charged particles (with the same electrical mobility of the singly charged particles) from the DMA. The filter and the rotameter after impactor 1 ensured the inlet of the impactor being calibrated was always at ambient pressure. The larger cutpoint stages were always installed above the test stage. For example, if stage 10 was under calibration, stages 1 to 9 were installed upstream of stage 10. This ensures that the stage being calibrated is at the typical pressure condition during normal use of the impactor. The three-way valve before the Faraday cage electrometer (FCE) could be turned toward the inlet (upstream) or the exit (downstream) of the test stage, so that the FCE could measure the particle concentrations upstream and downstream of the test stage. The ratio of the two measured currents was used to determine the collection efficiency of the test stage. The pressure drop through the needle valve was adjusted until the downstream volumetric flow rate was the same as the flow rate downstream of the test stage. It is possible that the needle valve could introduce some particle losses, which could lower the measured upstream particle concentration. This effect, however, should be insignificant since the efficiency curves never exceed 100% even for particles with sufficiently large Stokes number. Two different substrates, aluminum foils and glass fiber filters, were used for the impactor stages calibration.
Experimental calibration results and discussion
Collection efficiency curves with the aluminum foil substrates are shown in . The impactor design parameters and experimental calibration results are summarized in . The Reynolds number and the Stokes number are defined in Equations Equation(1)[1]
[1] and (Equation2
[2]
[2] ).
[1]
[1]
[2]
[2]
Figure 4. Efficiency curves of the low flow personal cascade impactor with aluminum foil substrates. Lines are the cumulative lognormal distribution function curves fitted to the data points.
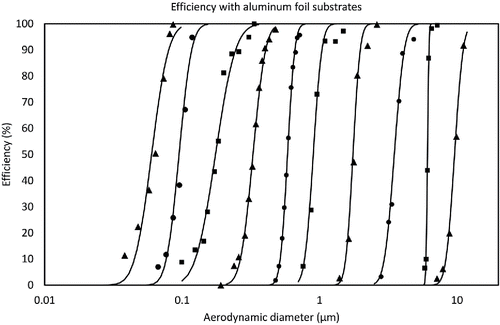
In above equations, ρg is the air density, W is the nozzle diameter, U is the average air velocity, μ is the air absolute viscosity, Q is volumetric flow rate, ρp is the particle density, Cc is the slip correction factor, dp is the particle diameter, and n is the number of nozzles on each stage. The square root of the Stokes number at 50% efficiency point, (St50)1/2, is in the range of 0.43 to 0.54. These values are very close to the theoretical values of 0.48 to 0.50 from numerical simulations for single nozzle impactors by Rader and Marple (Citation1985). The geometric standard deviations of the cumulative lognormal curves fitted to the experimental data are in the range of 1.02 to 1.25, demonstrating very good performance with sharp efficiency curves and minimal overlap. The cutpoints and the sharpness of the curves of the new impactor are very similar to the calibration curves of more conventional micro-orifice impactors that operate at much larger flow rates (Marple et al. Citation1991; Chen et al. Citation2016).
Experimental collection efficiency curves for the glass fiber substrates are shown in . The filter substrates are PALL Type A/E borosilicate glass fiber with a nominal thickness of 0.33 mm. The collection efficiency curves with glass fiber filter substrates are less steep and the cutpoints are smaller (), when compared to the curves and cutpoints for aluminum foil substrates. Another important difference is that the collection efficiency curves do not go to zero for small Stokes numbers. This additional particle collection is due to the filtration effect related to the penetration of the air jets into the filter media. For the top seven stages the collection efficiency curves go to a minimum of about 10% collection for small values of Stokes number. For the last three stages the curves are too flat and the filtration effect is even more pronounced. This phenomenon was first described by Rao and Whitby (Citation1978). They concluded that it was “attributed to the roughness of the filter surface, to penetration of filter fibers into the aerosol stream above the filter surface, and to aerosol jet penetration into the filter surface”. Huang et al. (Citation2001) numerically studied the performance of an impactor with porous metal substrate and proposed a semi-empirical equation to quantify the additional collection efficiency. Based on their work, Marjamäki and Keskinen (Citation2004) proposed an equation to estimate the change of the cutpoints. The influence of glass fiber filters on the impactor collection efficiency curves is more complex than that of porous metal substrates, since the filter can be deformed by the impinging high-speed jets. No previous work was found on quantifying this effect.
Table 2. Calibration results of the low flow cascade impactor for glass fiber substratesFootnotea.
Particle losses inside the impactor were also measured for the top five stages by quantifying the fraction of the uranine mass on the walls, nozzle plate surfaces, and the inlet (i.e., all surfaces other than the impaction plates). Particle losses for liquid particles were about 6% for 11.8 μm and decreased to below 4% for particles between 5 μm and 1 μm. For the lower stages, the losses were not measured directly. Instead, particle losses were estimated by checking if the efficiency curves went to zero during the calibration with aluminum foil substrates. It was found that losses were smaller than 2% for particles between 100 nm and 1 μm, and smaller than 10% for particles below 100 nm.
Conclusions
A compact, low-flow rate personal cascade impactor has been developed and experimentally calibrated in this work. This new personal impactor has a 2 L/min sample flow rate. The first eight stages of the impactor have a low pressure drop, so that it can be operated with a battery-powered sampling pump. The impactor was calibrated with aluminum foil and glass fiber filter substrates. The efficiency curves with aluminum foil substrates were sharp. Cutpoints and sharpness of cut were very similar to those of more conventional higher flow rate impactors (e.g., MOUDI). The ten stages of the new low flow rate impactor covers the aerodynamic diameter range of 60 nm to 9.6 μm. With glass fiber filter substrates, the collection efficiency curves are less steep, have smaller cutpoints and show a non-ideal small collection efficiency of about 10% at small Stokes numbers. When using glass fiber substrates it is recommended to only use the upper 8 stages of the impactor followed by a final filter.
The new low flow cascade impactor is suitable for personal as well as for general purpose sampling applications where aerosol concentrations are sufficiently high for collecting size-fractionated particle samples with a low flow rate.
References
- Asbach, C., Alexander, C., Clavaguera, S., Dahmann, D., Dozol, H., Faure, B., Fierz, M., Fontana, L., Iavicoli, I., Kaminski, H., MacCalman, L., Meyer-Plath, A., Simonow, B., Tongeren, M., and Todea, A. M. (2017). Review of Measurement Techniques and Methods for Assessing Personal Exposure to Airborne Nanomaterials in Workplaces. Sci. Total Environ., 603:793–806. https://doi.org/10.1016/j.scitotenv.2017.03.049
- Azong-Wara, N., Asbach, C., Stahlmecke, B., Fissan, H., Kaminski, H., Plitzko, S., Bathen, D., and Kuhlbusch, T. A. (2013). Design and Experimental Evaluation of a New Nanoparticle Thermophoretic Personal Sampler. J. Nanopart. Res., 15(4):1530.
- Cena, L. G., Anthony, T. R., and Peters, T. M. (2011). A Personal Nanoparticle Respiratory Deposition (NRD) Sampler. Environ. Sci. Technol., 45(15):6483–6490. https://doi.org/10.1021/es201379a
- Chen, M., Romay, F. J., Li, L., Naqwi, A., and Marple, V. A. (2016). A Novel Quartz Crystal Cascade Impactor for Real-Time Aerosol Mass Distribution Measurement. Aerosol Sci. Technol., 50(9):971–983.
- Chen, S.-C., Tsai, C.-J., Chen, H.-D., Huang, C.-Y., and Roam, G.-D. (2011). The influence of Relative Humidity on Nanoparticle Concentration and Particle Mass Distribution Measurements by the MOUDI. Aerosol Sci. Technol., 45(5):596–603.
- Csavina, J., Taylor, M. P., Félix, O., Rine, K. P., Eduardo Sáez, A., and Betterton, E. A. (2014). Size-Resolved Dust and Aerosol Contaminants Associated with Copper and Lead Smelting Emissions: Implications for Emission Management and Human Health. Sci. Total Environ., 493:750–756. https://doi.org/10.1016/j.scitotenv.2014.06.031
- Demokritou, P., Gupta, T., Ferguson, S., and Koutrakis, P. (2002). Development and Laboratory Performance Evaluation of a Personal Cascade Impactor. J. Air Waste Manage. Assoc., 52(10):1230–1237. https://doi.org/10.1080/10473289.2002.10470855
- Di Lemma, F. G., Colle, J. Y., Ernstberger, M., Rasmussen, G., Thiele, H., and Konings, R. J. M. (2014). RADES an Experimental Set-up for the Characterization of Aerosol Release from Nuclear and Radioactive Materials. J. Aerosol Sci., 70:36–49. https://doi.org/10.1016/j.jaerosci.2013.12.010
- Duan, H., Romay, F. J., Li, C., Naqwi, A., Deng, W., and Liu, B. Y. (2016). Generation of Monodisperse Aerosols by Combining Aerodynamic Flow-Focusing and Mechanical Perturbation. Aerosol Sci. Technol., 50(1):17–25.
- Huang, C.-H., Tsai, C.-J., and Shih, T.-S. (2001). Particle Collection Efficiency of an Inertial Impactor with Porous Metal Substrates. J. Aerosol Sci., 32(9):1035–1044.
- Koehler, K. A., and Peters, T. M. (2015). New Methods for Personal Exposure Monitoring for Airborne Particles. Curr. Environ. Health Rep., 2(4):399–411.
- Marjamäki, M., and Keskinen, J. (2004). Estimation of the Cutpoint of an Impactor with Porous Substrates. J. Aerosol Sci., 35(5):657–663. https://doi.org/10.1016/j.jaerosci.2003.11.006
- Marple, V. A., Rubow, K. L., and Behm, S. M. (1991). A Microorifice Uniform Deposit Impactor (MOUDI): Description, Calibration, and Use. Aerosol Sci. Technol., 14(4):434–446.
- Marple, V., Olson, B., Romay, F., Hudak, G., Geerts, S. M., and Lundgren, D. (2014). Second Generation Micro-Orifice Uniform Deposit Impactor, 120 MOUDI-II: Design, Evaluation, and Application to Long-Term Ambient Sampling. Aerosol Sci. Technol., 48(4):427–433.
- Marple, V., Rubow, K., Ananth, G., and Fissan, H. J. (1986). Micro-Orifice Uniform Deposit Impactor. J. Aerosol Sci., 17(3):489–494.
- Martonen, T., Clark, M., Nelson, D., Willard, D., and Rossignol, E. (1982). Evaluation of a Mini-Cascade Impactor for Sampling Exposure Chamber Atmospheres. Fundam. Appl. Toxicol., 2(4):149–152.
- Mason, R. H., Chou, C., McCluskey, C. S., Levin, E. J. T., Schiller, C. L., Hill, T. C. J., … Bertram, A. K. (2015). The Micro-Orifice Uniform Deposit Impactor–Droplet Freezing Technique (MOUDI-DFT) for Measuring Concentrations of Ice Nucleating Particles as a Function of Size: Improvements and Initial Validation. Atmos. Meas. Tech., 8(6):2449–2462. https://doi.org/10.5194/amt-8-2449-2015
- Miller, A., Frey, G., King, G., and Sunderman, C. (2010). A Handheld Electrostatic Precipitator for Sampling Airborne Particles and Nanoparticles. Aerosol Sci. Technol., 44(6):417–427. https://doi.org/10.1080/02786821003692063
- Misra, C., Singh, M., Shen, S., Sioutas, C., and Hall, P. M. (2002). Development and Evaluation of a Personal Cascade Impactor Sampler (PCIS). J. Aerosol Sci., 33(7):1027–1047. https://doi.org/10.1016/S0021-8502(02)00055-1
- Pöschl, U. (2005). Atmospheric Aerosols: Composition, Transformation, Climate and Health Effects. Angew. Chem. Int. Ed., 44(46):7520–7540. https://doi.org/10.1002/anie.200501122
- Rader, D. J., and Marple, V. A. (1985). Effect of ultra-Stokesian Drag and Particle Interception on Impaction Characteristics. Aerosol Sci. Technol., 4(2):141–156.
- Rao, A. K., and Whitby, K. T. (1978). Non-Ideal Collection Characteristics of Inertial Impactors—I. Single-Stage Impactors and Solid Particles. J. Aerosol Sci., 9(2):77–86. https://doi.org/10.1016/0021-8502(78)90069-1
- Rubow, K. L., Marple, V. A., Olin, J., and McCawley, M. A. (1987). A Personal Cascade Impactor: Design, Evaluation and Calibration. Am. Ind. Hyg. Assoc. J., 48(6):532–538. https://doi.org/10.1080/15298668791385174
- Stein, S. W., Turpin, B. J., Cai, X., Huang, P.-F., and McMurry, P. H. (1994). Measurements of Relative Humidity-Dependent Bounce and Density for Atmospheric Particles using the DMA-Impactor Technique. Atmos. Environ., 28(10):1739–1746.