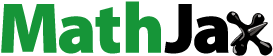
Abstract
Electronic cigarettes (ECIGs) are appealing in part because of the many flavors of the liquids used in them. Concerns have been raised that some ECIG liquid flavors, especially those that are sweet, are attracting otherwise nicotine-naïve youth to ECIGs. Sucralose is an artificial, non-caloric sweetener that is added to some ECIG liquids. In this study, we evaluated the toxicants, namely isomers of chloropropanols that can be produced when sucralose-containing ECIG liquid is aerosolized. An analytical separation method relying on solid-phase extraction (SPE) to isolate chloropropanols from the propylene glycol/glycerol matrix was developed. Chloropropanols were then derivatized by silylation before they were analyzed on GC-MS. The influence of different ECIG operating conditions on the generation of chloropropanols was studied by varying ECIG device design and power output and also the sucralose concentration of the liquid. Heated sucralose-containing ECIG liquids produce two toxic compounds that can be found in the resulting aerosols. The two chloropropanols, 3-monochloro-1,2-propanediol (3-MCPD), and 1,3-dichloropropanol (1,3-DCP) that were detected under all conditions were found to be correlated significantly with liquid sucralose content. Effective regulation of ECIGs will minimize user and bystander exposure to these and other ECIG toxicants.
Copyright © 2019 American Association for Aerosol Research
EDITOR:
Introduction
The prevalence of electronic cigarette (ECIG) use has increased tremendously since their introduction to the market (McMillen et al. Citation2015; Anic et al. Citation2018). This increased use is usually attributed to several factors including the perception of reduced risk relative to combustible cigarettes and high appeal to former and current smokers as well as to nicotine-naïve users (Majeed et al. Citation2017; Bao et al. Citation2018; Fulton et al. Citation2018). ECIGs are advertised as safer alternatives to combustible cigarettes because they contain a simpler matrix of chemicals (polyol solvents with or without nicotine and flavorings) subjected to lower temperatures during aerosolization (Breland et al. Citation2017). Moreover, there are claims that these chemicals distill almost intact to the generated aerosols that will be inhaled by the user (Margham et al. Citation2016). However, these claims were shown to be false with the detection of toxicants like aldehydes, reactive oxygen species, and metals at levels sometimes comparable or greater than those of combustible tobacco products (Jensen et al. Citation2015; Ogunwale et al. Citation2017; Wang et al. Citation2017; Haddad et al. Citation2019; Salamanca et al. Citation2018). ECIG appeal is increased by the plethora of flavorings in ECIG liquids – traditional flavors such as “tobacco” and “menthol” are available, as are nontraditional flavors that are intended to resemble fruit, desserts, candy, and other sweet foods (St.Helen et al. Citation2018; Bono et al. Citation2019). Recently, several studies have reported that flavor additives increase the toxicant profile of ECIG aerosol (Khlystov and Samburova Citation2016; Bitzer et al. Citation2018), with one report showing higher toxicity from adducts formed between flavor chemicals and propylene glycol (PG), a common ECIG base liquid (Erythropel et al. Citation2018).
In 2009, the US FDA banned the addition of characterizing flavors, other than menthol, to tobacco cigarettes. However, characterizing flavors are not banned in the US for other tobacco or nicotine delivery products, including waterpipe tobacco (shisha), smokeless tobacco products, and ECIGs (FDA Citation2018a). In the case of ECIGs, sweet flavors were found to increase product appeal among youth (Kroemer et al. Citation2018), increasing the risk of nicotine dependence among youth and probably acting as a gateway to smoking (Spindle et al. Citation2017; Morgenstern et al. Citation2018; NIDA Citation2018). A sweet flavor in ECIG liquid can be obtained by the addition of saccharides (sucrose or honey monosaccharides) or artificial non-caloric sweeteners/sweet enhancers (such as ethyl maltol, sucralose or sorbitol) (Miao et al. Citation2016; Fagan et al. Citation2018). Sucralose is a high intensity sweetener (up to 600 times sweeter than sucrose) that is marketed as an additive for Do-It-Yourself (DIY) mixing of ECIG liquids (Rosbrook et al. Citation2017; Amazon Citation2018). FDA approves it as a general sweetener for food, but it is not currently listed as “generally recognized as safe” (FDA Citation2018b).
The safety and toxicity of sucralose have become controversial (Abou-Donia et al. Citation2008; Brusick et al. Citation2009; Grotz and Munro Citation2009; Berry et al. Citation2016). Although originally thought to be thermally stable, several studies reported the degradation of sucralose at temperatures that are expected during cooking (Hutchinson, Ho, and Ho Citation1999; Dong et al. Citation2011, Citation2013). The effect of temperature on sucralose was studied by thermogravimetric analysis/differential scanning calorimetry techniques and showed that degradation of sucralose starts at temperatures as low as 120 °C yielding hydrochloric acid (HCl) (Bannach et al. Citation2009; de Oliveira, de Menezes, and Catharino Citation2015). Furthermore, it has been shown that heating sucralose in the presence of glycerol in some food products leads to the formation of chloropropanols that have considerable toxicity (Rahn and Yaylayan Citation2010; Schiffman and Rother Citation2013). Chloropropanol formation in foods raises a concern about the use of sucralose as an additive in ECIG liquids. In this study, we assessed the generation of chloropropanols upon aerosolizing a sucralose-containing liquid in two ECIG devices at different powers. To enable quantification of chloropropanols in ECIG aerosols, an analytical separation method was developed and optimized to isolate chloropropanols from propylene glycol (PG) and vegetable glycerin (VG), the primary ECIG aerosol constituents. Following isolation, chloropropanols were derivatized by silylation and then analyzed using Gas Chromatography coupled to a Mass Spectroscopy GC-MS (González et al. Citation2011; Kim et al. Citation2015; Yang et al. Citation2018).
Materials and methods
Materials
PG (99.5%) (CAS No 57-55-6), VG (99%–101%) (CAS No 56-81-5), 1,3-dichloro-2-propanol (1,3-DCP) (CAS No 96-23-1), (±)-3-Chloro-1,2-propanediol (3-MCPD) (CAS No 96-24-2), 2-bromoethanol (2-BE) (CAS No 540-51-2), and sucralose (CAS No 56038-13-2) were procured from Sigma-Aldrich. N,O-Bis(trimethylsilyl) trifluoroacetamide (BSTFA) with 1% trimethylchlorosilane (25561-30-2) used for the derivatization of chloropropanols before GC analysis was purchased from Fluka. HPLC grade solvents including ethyl acetate and methanol were purchased from Sigma-Aldrich. Quartz filters (ADVENTEC, QR-100 47 mm) were procured from Pall Corporation. TELOS neo PRP 200 mg/6 ml solid phase extraction (SPE) cartridges were purchased from TELOS. ECIG devices, including above-Ohm Vaporfi platinum II (2.2 Ω coil) and sub-Ohm Smok TFV12 (0.15 Ω coil) were purchased from online vendors.
Methods
Study design
Our approach was to evaluate the influence of electric power and sucralose content on chloropropanol yields in smoking machine generated-aerosols emitted by an above-Ohm and sub-Ohm ECIG device. Two different loadings of sucralose (1% and 5%) in ECIG liquid were prepared by dissolving pure sucralose powder in standard PG, VG, and deionized water mixture (ratio: 28:67:5, respectively). These sucralose loadings were chosen based on previously reported work investigating the influence of sucralose on the sweetness of commercial flavors (Rosbrook et al. Citation2017). Different powers were tested for each ECIG design: 100, 150, and 200 W for sub-Ohm and 5 and 11 W for above-Ohm ECIGs. These powers were consistent with the manufacturer’s recommendations for these devices. The formation of various mono- and di-chloropropanols is possible from PG and VG yielding two (1-chloro-2-propanol and 2-chloro-1-propanol) and four chloropropanols (3-chloro-1,2-propanediol (3-MCPD), 2-chloro-1,3-propanediol, 1,3-dichloro-2-propanol (1,3-DCP), and 2,3-dichloro-1-propanol), respectively. In this study, the method was optimized to detect the most toxic compounds, namely 3-MCPD and 1,3-DCP (IARC classification, group 2B).
Aerosol generation and sampling
The AUB Aerosol Lab Vaping Instrument (ALVIN) was used to generate ECIG aerosols (Talih et al. Citation2015), using a puffing regimen consisting of 15 puffs for above-Ohm and three puffs for the sub-Ohm device, 4-s puff duration, 10-s inter-puff interval, and 1 L/min flow rate. The generated aerosols were trapped on 47 mm quartz filters, using the same setup previously described in Talih et al. (Citation2015).
Separation method
A clean-up analytical method using solid phase extraction (SPE) cartridges was developed and optimized ().
Figure 1. A stepwise scheme of the optimized analytical method for the separation of chloropropanols.
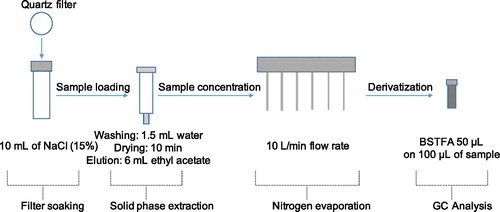
As shown in , aerosols trapped on the quartz filters were extracted in 10 mL NaCl aqueous solution (15% w/w) and then loaded onto an SPE cartridge. The SPE was pre-conditioned with ethyl acetate, methanol, and water (4 mL each). After loading the sample, the SPE was washed with 1.5 mL of water and then air-dried for 10 min to remove any remaining water on the stationary phase. The analytes were then eluted with 6 mL of ethyl acetate using a vacuum manifold at a rate of 1 drop/s. The organic layer was concentrated under nitrogen flow (10 L/min) down to 100 µL and spiked with Bromoethanol as an internal standard for quantification. The mixture was reacted with N, O-Bistrifluoroacetamide (BSTFA) (50 µL) for derivatization of the hydroxyl groups while heating at 70 °C for 30 min. An aliquot was injected into GC-MS for analysis. summarizes the analytical parameters of the optimized method.
Table 1. Method validation parameters.
GC-MS method
The GC-MS analysis was performed on a Thermo Trace GC-2000 POLARIS QMS instrument equipped with a thermostatically controlled AI 3000 auto-sampler and a TG-5MS Thermo Scientific fused silica capillary GC column (30 m × 0.25 mm × 0.25 µm). The mobile phase was helium delivered at 1 mL/min flow rate. The injector temperature was 250 °C, with a split-less injection mode of 1 µL. The oven temperature program was: 50 °C for 1 min, 5 °C/min ramp to 90 °C, and another ramp of 10 °C/min to 240 °C. The total run time was 25 min, and the solvent delay time was 6.6 min. The quantification was performed using selected ion mass (SIM) mode with two qualifiers and one quantifier peak for each analyte, as shown in .
Table 2. Qualifiers and quantifier peaks in GC-MS (m/z) of the selected analytes.
Results and discussion
The optimized separation method allowed the isolation of chloropropanols from PG and VG matrix due to the salting-out effect of NaCl that retained the chloropropanols on the stationary phase of the SPE. Both chloropropanols, 3-MCPD, and 1,3-DCP, were identified only in aerosols and not in the gas phase (Figures S3–S5 in the online supplementary information [SI]) in all conditions at levels ranging from zero to 25 µg/puff. Chloropropanol yields were strongly dependent on sucralose concentration (p < 0.05) and were found to be unrelated to the power level (p < 0.899 and 0.528 for 3-MCPD and 1,3-DCP, respectively). Sub-ohm and above-Ohm devices had similar levels of 3-MCPD, while sub-Ohm devices exhibited greater levels of DCP, although not significant (p < 0.147). Results are illustrated in .
Figure 2. A bar graph of 3-MCPD (a) and 1,3-DCP (b) concentration versus the different ECIG operating parameters. %Su, P(W), and ECIG refer to sucralose content (%), power output (W), and ECIG design, respectively.
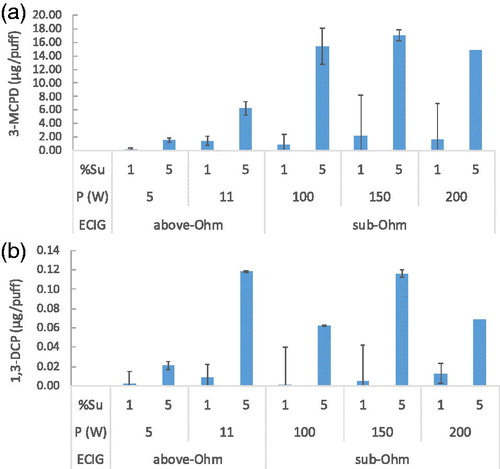
The chloropropanols are produced from the halogenation reaction of polyols like PG and VG with HCl. This latter is generated from sucralose degradation upon heating (R1 and R2). Thermo-gravimetric analysis (TGA) of sucralose confirmed, as reported in the literature, that the emission of HCl initiates at 130 °C (SI Figure S1) and that aerosolizing ECIG liquid containing sucralose dissolved in PG leads to the formation of gaseous acid-catalyzed products as depicted by infrared (IR) and nuclear magnetic resonance (NMR) spectroscopy analysis (SI Figures S3–S5). Products found in the gas phase like acetals were detected only in the absence of nicotine in the ECIG liquid. Acetal is known to be an acid transformation catalyzed in this case by HCl that is produced from the sucralose degradation reaction. In the presence of nicotine, an HCl-nicotine scavenging reaction is most likely to take place, eliminating, thereby the formation of the acetal.
The influence of different ECIG operating parameters including device type, power, and sucralose liquid content on the generation of 3-MCPD and 1,3-DCP is summarized in . 3-MCPD and 1,3-DCP result from the reactions of HCl with VG and 3-MCPD, respectively. 1,3-DCP concentration seems to be limited by the formation of 3-MCPD, and as such, it is observed at much lower concentrations. Both 3-MCPD and 1,3-DCP exhibit carcinogenic and genotoxic properties in animals (Eder and Weinfurtner Citation1994; Cho et al. Citation2008; Baer, de la Calle, and Taylor Citation2010). Also, 3-MCPD has been reported to have male antifertility effects and is commonly used as a rat chemosterilant (Kwack et al. Citation2004). They are classified as possibly carcinogenic to humans (group 2B) by the International Agency for Research on Cancer (IARC) (IARC Citation2013a, Citation2013b). As per IARC classification, the toxicity of these compounds is similar to acetaldehyde reported as the most harmful carbonyl in ECIGs after formaldehyde (Bekki et al. Citation2014).
summarizes the multiple variable analysis of the correlation between the formation of 3-MCPD and 1,3-DCP and the different ECIG operating conditions. Only sucralose concentration in the ECIG liquid significantly affected the emission of chloropropanols (p < 0.05). The fact that power has no significant effect on the generation of chloropropanols (p value is 0.528 for 1,3-DCP and 0.899 for 3-MCPD) could be explained by the low stability of sucralose, degrading at relatively low temperatures that can be attained by all the tested powers.
Table 3. Statistical analysis (p value) of the effect of different ECIG operating parameters on chloropropanols formation.
Our results show that the addition of sucralose as a sweetener in ECIG liquid yields chloropropanols that are classified as possibly carcinogenic to humans (Group 2B). There is limited information about the toxicity of chloropropanols (Andres, Appel, and Lampen Citation2013). The only toxicity level reported for chloropropanols is a provisional maximum tolerable daily intake (PMTDI) of 2 µg/kg of body weight estimated from a long-term study of toxicity and carcinogenicity in rats (FSANZ Citation2003). If we consider a rough estimation of ECIG use at 150 puffs/day, a 70 kg user will be exposed to 0–18 times more than PMTDI depending on the operating conditions, keeping in mind the difference between inhalation and ingestion routes. The detection of these possible carcinogens in the aerosols of sweet flavored liquids adds to the risk of carcinogenic carbonyls emitted from the degradation of the base liquid. Therefore, policymakers worldwide should consider banning the use of sucralose as an additive in ECIG liquids.
Declaration of interest
Drs. Eissenberg and Shihadeh are paid consultants in litigation against the tobacco industry and are named on a patent for a device that measures the puffing behavior of electronic cigarette users. All other authors have no potential conflict of interest to be disclosed.
Supplemental Material
Download MS Word (514 KB)Additional information
Funding
References
- Abou-Donia, M. B., E. M. El-Masry, A. A. Abdel-Rahman, R. E. McLendon, and S. S. Schiffman. 2008. Splenda alters gut microflora and increases intestinal P-Glycoprotein and cytochrome P-450 in male rats. J. Toxicol. Environ. Health Part A 71 (21):1415–29. doi:10.1080/15287390802328630.
- Amazon. 2018. NuSweet liquid sucralose, concentrated liquid sweetener, 4 Oz. Accessed November 6, 2018. https://www.amazon.com/NuSweet-Liquid-Sucralose-Concentrated-Sweetener/dp/B00I28OQ7K.
- Andres, S., K. E. Appel, and A. Lampen. 2013. Toxicology, occurrence and risk characterisation of the chloropropanols in food: 2-Monochloro-1,3-propanediol, 1,3-dichloro-2-propanol and 2,3-dichloro-1-propanol. Food Chem. Toxicol. 58:467–8. doi:10.1016/j.fct.2013.05.024.
- Anic, G. M., E. Holder-Hayes, B. K. Ambrose, B. L. Rostron, B. Coleman, A. Jamal, and B. J. Apelberg. 2018. E-cigarette and smokeless tobacco use and switching among smokers: Findings from the national adult tobacco survey. Am. J. Prevent. Med. 54 (4):539–51. doi:10.1016/j.amepre.2017.12.010.
- Baer, I., B. de la Calle, and P. Taylor. 2010. 3-MCPD in food other than soy sauce or hydrolysed vegetable protein (HVP). Anal. Bioanal. Chem. 396 (1):443–56. doi:10.1007/s00216-009-3177-y.
- Bannach, G., R. R. Almeida, L. G. Lacerda, E. Schnitzler, and M. Ionashiro. 2009. Thermal stability and thermal decomposition of sucralose. Eclet. Quím. 34 (4):21–6. doi:10.1590/S0100-46702009000400002.
- Bao, W., G. Xu, J. Lu, L. G. Snetselaar, and R. B. Wallace. 2018. Changes in electronic cigarette use among adults in the United States, 2014-2016. J. Am. Med. Assoc. 319 (19):2039–41. doi:10.1001/jama.2018.4658.
- Bekki, K., S. Uchiyama, K. Ohta, Y. Inaba, H. Nakagome, and N. Kunugita. 2014. Carbonyl compounds generated from electronic cigarettes. Int. J. Environ. Res. Public Health 11 (11):11192–200. doi:10.3390/ijerph111111192.
- Berry, C., D. Brusick, S. M. Cohen, J. F. Hardisty, V. L. Grotz, and G. M. Williams. 2016. Sucralose non-carcinogenicity: A review of the scientific and regulatory rationale. Nutr. Cancer 68 (8):1247–61. doi:10.1080/01635581.2016.1224366.
- Bitzer, Z. T., R. Goel, S. M. Reilly, R. J. Elias, A. Silakov, J. Foulds, J. Muscat, and J. P. Richie. 2018. Effect of flavoring chemicals on free radical formation in electronic cigarette aerosols. Free Radic. Biol. Med. 120:72–9. doi:10.1016/j.freeradbiomed.2018.03.020.
- Bono, R. S., A. J. Barnes, R. C. Lester, and C. O. Cobb. 2019. Effects of electronic cigarette liquid flavors and modified risk messages on perceptions and subjective effects of E-cigarettes. Health Educ. Behav. 46 (2):197–203. doi:10.1177/1090198118806965.
- Breland, A., E. Soule, A. Lopez, C. Ramôa, A. El-Hellani, and T. Eissenberg. 2017. Electronic cigarettes: What are they and what do they do? Ann. N. Y. Acad. Sci. 1394 (1):5–30. doi:10.1111/nyas.12977.
- Brusick, D., J. F. Borzelleca, M. Gallo, G. Williams, J. Kille, A. Wallace Hayes, F. Xavier Pi-Sunyer, C. Williams, and W. Burks. 2009. Expert panel report on a study of splenda in male rats. Regul. Toxicol. Pharmacol. 55 (1):6–12. doi:10.1016/j.yrtph.2009.06.013.
- Cho, W.-S., B. S. Han, K. T. Nam, K. Park, M. Choi, S. H. Kim, J. Jeong, and D. D. Jang. 2008. Carcinogenicity study of 3-monochloropropane-1,2-diol in Sprague-Dawley rats. Food Chem. Toxicol. 46 (9):3172–7. doi:10.1016/j.fct.2008.07.003.
- de Oliveira, D. N., M. de Menezes, and R. R. Catharino. 2015. Thermal degradation of sucralose: A combination of analytical methods to determine stability and chlorinated byproducts. Sci. Rep. 5 (1):9598. doi:10.1038/srep09598.
- Dong, S., G. Liu, B. Zhang, L. Gao, and M. Zheng. 2013. Formation of polychlorinated naphthalenes during the heating of cooking oil in the presence of high amounts of sucralose. Food Control 32 (1):1–5. doi:10.1016/j.foodcont.2012.11.001.
- Dong, S., J. Wu, G. Liu, B. Zhang, and M. Zheng. 2011. Unintentionally produced dioxin-like polychlorinated biphenyls during cooking. Food Control 22 (11):1797–802. doi:10.1016/j.foodcont.2011.04.022.
- Eder, E., and E. Weinfurtner. 1994. Mutagenic and carcinogenic risk of oxygen containing chlorinated C-3 hydrocarbons: Putative secondary products of C-3 chlorohydrocarbons and chlorination of water. Chemosphere 29 (9-11):2455–66. doi:10.1016/0045-6535(94)90413-8.
- Erythropel, H. C., S. V. Jabba, T. M. DeWinter, M. Mendizabal, P. T. Anastas, S. E. Jordt, and J. B. Zimmerman. 2018. Formation of flavorant–propylene glycol adducts with novel toxicological properties in chemically unstable E-cigarette liquids. Nicotine Tob. Res. nty192. doi:10.1093/ntr/nty192.
- Fagan, P., P. Pokhrel, T. A. Herzog, E. T. Moolchan, K. D. Cassel, A. A. Franke, X. Li, I. Pagano, D. R. Trinidad, K-L. K. Sakuma, et al. 2018. Sugar and aldehyde content in flavored electronic cigarette liquids. Nicotine Tob. Res. 20 (8):985–92. doi:10.1093/ntr/ntx234.
- FDA. 2018a. Menthol and other flavors in tobacco products. Accessed November 5, 2018. https://www.fda.gov/tobaccoproducts/labeling/productsingredientscomponents/ucm2019416.htm#reference.
- FDA. 2018b. Additional information about high-intensity sweeteners permitted for use in food in the United States. Accessed November 6, 2018. https://www.fda.gov/food/food-additives-petitions/additional-information-about-high-intensity-sweeteners-permitted-use-food-united-states.
- FSANZ. 2003. Food standards Australia New Zealand technical report: Chloropropanols in food: An analysis of the public health risk. Accessed November 8, 2018. https://www.foodstandards.gov.au/publications/Documents/Chloropropanol%20Report%20(no%20appendices)%20-%2011%20Sep%2003b-2.pdf.
- Fulton, E., K. Gokal, S. Griffiths, and S. Wild. 2018. More than half of adolescent E-cigarette users had never smoked a cigarette: findings from a study of school children in the UK. Public Health 161:33–5. doi:10.1016/j.puhe.2018.04.014.
- González, P., I. Racamonde, A. M. Carro, and R. A. Lorenzo. 2011. Combined solid-phase extraction and gas chromatography–mass spectrometry used for determination of chloropropanols in water. J. Sep. Sci. 34 (19):2697–704. doi:10.1002/jssc.201100312.
- Grotz, V. L., and I. C. Munro. 2009. An overview of the safety of sucralose. Regul. Toxicol. Pharmacol. 55 (1):1–5. doi:10.1016/j.yrtph.2009.05.011.
- Haddad, C., R. Salman, A. El-Hellani, S. Talih, A. Shihadeh, and N. A. Saliba. 2019. Reactive oxygen species emissions from supra- and sub-Ohm electronic cigarettes. J. Anal. Toxicol. 43 (1):45–50. doi:10.1093/jat/bky065.
- Hutchinson, S. A., G. S. Ho, and C. T. Ho. 1999. Stability and degradation of the high‐intensity sweeteners: Aspartame, alitame, and sucralose. Food Rev. Int. 15 (2):249–61. doi:10.1080/87559129909541189.
- IARC. 2013a. 1,3-dichloro-2-propanol. Accessed February 7, 2019. https://monographs.iarc.fr/wp-content/uploads/2018/06/mono101-011.pdf.
- IARC. 2013b. 3-monochloro-1,2-propanediol. Accessed February 7, 2019. https://monographs.iarc.fr/wp-content/uploads/2018/06/mono101-010.pdf.
- Jensen, R. P., W. Luo, J. F. Pankow, R. M. Strongin, and D. H. Peyton. 2015. Hidden formaldehyde in E-cigarette aerosols. N. Engl. J. Med. 372 (4):392–394. doi:10.1056/NEJMc1413069.
- Khlystov, A., and V. Samburova. 2016. Flavoring compounds dominate toxic aldehyde production during E-cigarette vaping. Environ. Sci. Technol. 50 (23):13080–5. doi:10.1021/acs.est.6b05145.
- Kim, W., Y. A. Jeong, J. On, A. Choi, J.-Y. Lee, J. G. Lee, K.-G. Lee, and H. Pyo. 2015. Analysis of 3-MCPD and 1,3-DCP in various foodstuffs using GC-MS. Toxicol. Res. 31 (3):313–9. doi:10.5487/TR.2015.31.3.313.
- Kroemer, N. B., M. G. Veldhuizen, R. Delvy, B. P. Patel, S. S. O'Malley, and D. M. Small. 2018. Sweet taste potentiates the reinforcing effects of e-cigarettes. Eur Neuropsychopharmacol. 28 (10):1089–102. doi:10.1016/j.euroneuro.2018.07.102.
- Kwack, S. J., S. S. Kim, Y. W. Choi, G. S. Rhee, R. D. Lee, J. H. Seok, S. Y. Chae, Y. H. Won, K. J. Lim, K. S. Choi, et al. 2004. Mechanism of antifertility in male rats treated with 3-monochloro-1,2-propanediol (3-MCPD). J. Toxicol. Environ. Health Part A 67 (23–24):2001–4. doi:10.1080/15287390490514651.
- Majeed, B. A., S. R. Weaver, K. R. Gregory, C. F. Whitney, P. Slovic, T. F. Pechacek, and M. P. Eriksen. 2017. Changing perceptions of harm of E-cigarettes among U.S. Adults, 2012–2015. Am. J. Prevent. Med. 52 (3):331–8. doi:10.1016/j.amepre.2016.08.039.
- Margham, J., K. McAdam, M. Forster, C. Liu, C. Wright, D. Mariner, and C. Proctor. 2016. Chemical composition of aerosol from an E-cigarette: A quantitative comparison with cigarette smoke. Chem. Res. Toxicol. 29 (10):1662–78. doi:10.1021/acs.chemrestox.6b00188.
- McMillen, R. C., M. A. Gottlieb, R. M. W. Shaefer, J. P. Winickoff, and J. D. Klein. 2015. Trends in electronic cigarette use among U.S. Adults: Use is increasing in both smokers and nonsmokers. Nicotine Tob. Res. 17 (10):1195–202. doi:10.1093/ntr/ntu213.
- Miao, S., E. S. Beach, T. J. Sommer, J. B. Zimmerman, and S.-E. Jordt. 2016. High-intensity sweeteners in alternative tobacco products. Nicotine Tob. Res. 18 (11):2169–73. doi:10.1093/ntr/ntw141.
- Morgenstern, M., A. Nies, M. Goecke, and R. Hanewinkel. 2018. E-cigarettes and the use of conventional cigarettes. Dtsch. Ärztebl. Int. 115 (14):243–8.
- NIDA. 2018. Electronic cigarettes (E-cigarettes). Accessed November 5, 2018. https://www.drugabuse.gov/publications/drugfacts/electronic-cigarettes-e-cigarettes.
- Ogunwale, M. A., M. Li, M. V. Ramakrishnam Raju, Y. Chen, M. H. Nantz, D. J. Conklin, and X.-A. Fu. 2017. Aldehyde detection in electronic cigarette aerosols. ACS Omega 2 (3):1207–14. doi:10.1021/acsomega.6b00489.
- Rahn, A., and V. Yaylayan. 2010. Thermal degradation of sucralose and its potential in generating chloropropanols in the presence of glycerol. Food Chem. 118 (1):56–61. doi:10.1016/j.foodchem.2009.04.133.
- Rosbrook, K., H. C. Erythropel, T. M. DeWinter, M. Falinski, S. O’Malley, S. Krishnan-Sarin, P. T. Anastas, J. B. Zimmerman, and B. G. Green. 2017. The effect of sucralose on flavor sweetness in electronic cigarettes varies between delivery devices. PLoS One 12 (10):e0185334. doi:10.1371/journal.pone.0185334.
- Salamanca, J. C., J. Meehan-Atrash, S. Vreeke, J. O. Escobedo, D. H. Peyton, and R. M. Strongin. 2018. E-cigarettes can emit formaldehyde at high levels under conditions that have been reported to be non-averse to users. Sci. Rep. 8 (1):7559. doi:10.1038/s41598-018-25907-6.
- Schiffman, S. S., and K. I. Rother. 2013. Sucralose, a synthetic organochlorine sweetener: Overview of biological issues. J. Toxicol. Environ. Health Part B 16 (7):399–451. doi:10.1080/10937404.2013.842523.
- Spindle, T. R., M. M. Hiler, M. E. Cooke, T. Eissenberg, K. S. Kendler, and D. M. Dick. 2017. Electronic cigarette use and uptake of cigarette smoking: a longitudinal examination of U.S. college students. Addict. Behav. 67:66–72. doi:10.1016/j.addbeh.2016.12.009.
- St.Helen, G., M. Shahid, S. Chu, and N. L. Benowitz. 2018. Impact of e-liquid flavors on e-cigarette vaping behavior. Drug Alcohol Depend. 189:42–8. doi:10.1016/j.drugalcdep.2018.04.032.
- Talih, S., Z. Balhas, T. Eissenberg, R. Salman, N. Karaoghlanian, A. El Hellani, R. Baalbaki, N. Saliba, and A. Shihadeh. 2015. Effects of user puff topography, device voltage, and liquid nicotine concentration on electronic cigarette nicotine yield: Measurements and model predictions. Nicotine Tob. Res. 17 (2):150–7. doi:10.1093/ntr/ntu174.
- Wang, P., W. Chen, J. Liao, T. Matsuo, K. Ito, J. Fowles, D. Shusterman, M. Mendell, and K. Kumagai. 2017. A device-independent evaluation of carbonyl emissions from heated electronic cigarette solvents. PLoS One 12 (1):e0169811. doi:10.1371/journal.pone.0169811.
- Yang, S., K. Kwon, J. Choi, and C.-H. Jo. 2018. Improvement of a GC-MS analytical method for the simultaneous detection of 3-MCPD and 1,3-DCP in food. Food Sci. Biotechnol. 27 (3):859–66. doi:10.1007/s10068-018-0312-6.