The global COVID-19 pandemic has given rise to discussions on airborne transmission of coronaviruses such as SARS-CoV-2 (Lewis Citation2020). Asadi et al. (Citation2020) and Drossinos and Stilianakis (Citation2020) provide insightful comments on the role aerosols may play in the transmission of respiratory pathogens. As they point out, the terms “contact,” “droplet,” and “airborne” are used when describing respiratory disease transmission routes in the medical field, though their boundaries are often not clearly defined. The National Health Commission of China states that “contact” and “droplet” are the major transmission routes of SARS-CoV-2 (China NHC Citation2020). This conclusion was based mainly on clinical and epidemiological evidence. Nationwide interventions, such as maintaining social distance and wearing face masks in public places, were implemented in China. Such interventions would appear to help reduce the transmission risk during many scenarios, including face-to-face conversation discussed by Asadi et al. (Citation2020). In addition to reducing uptake by susceptible hosts, masks (including surgical ones) can also reduce the emission of human coronaviruses in both respiratory droplets and aerosols from infected individuals (Leung et al. Citation2020). The World Health Organization (WHO) supports the theory of “contact” and “droplets” as two major transmission routes of SARS-CoV-2 (WHO Citation2020). As for “airborne” transmission, NHC China considers this possible in some scenarios, such as exposure to high concentrations of virus-laden aerosols in a relatively enclosed environment over a long time. Fine aerosols (<1 μm) are too small to sediment at a significant rate, allowing them to stay airborne anywhere from a few hours up to weeks at a time.
In addition to SARS-CoV-2, there are other coronaviruses that have emerged in the human population during the last two decades, including SARS-CoV-1 in 2003 and MERS-CoV in 2012. After their first outbreaks, MERS-CoV reappeared in later years, while SARS-CoV-1 did not. Under controlled environmental conditions in a rotating chamber, the stability (viability) of these three types of coronaviruses in aerosols (<5 μm) was examined as a function of time (Pyankov et al. Citation2018; van Doremalen et al. Citation2020). As shown in , the reduction in infectious titer of SARS-CoV-2 at 20 °C and 65% RH is very similar to that of SARS-CoV-1 under the same conditions, while MERS-CoV under two different sets of conditions decays much faster. Note that aerosol size distributions might have changed during the course of these experiments, especially under higher temperatures. These controlled studies do not necessarily reflect real infectious scenarios. The fast decay of its viability under environmental conditions representing the Middle Eastern region (38 °C and 24% RH) has been argued as the reason for the lower spread of MERS in the human population (van Doremalen, Bushmaker, and Munster Citation2013). However, COVID-19 caused by SARS-CoV-2 has spread dramatically more than SARS caused by SARS-CoV-1, despite the similar stability of their aerosols. As of 8 May 2020, more than 3.7 million people were confirmed as having been infected with COVID-19. By comparison, only 8422 and 1321 cases of SARS and MERS, respectively, have been reported.
Figure 1. Viability of SARS-CoV-2, SARS-CoV-1, and MERS-CoV in aerosols. Data are from Pyankov et al. (Citation2018) and van Doremalen et al. (Citation2020). Polydisperse aerosols with a size less than 5 μm were tested. Infectious titer for SARS-CoV-2 and SARS-CoV-1 is per liter of air sampled; for MERS-CoV it is per milliliter of liquid with sampled aerosols.
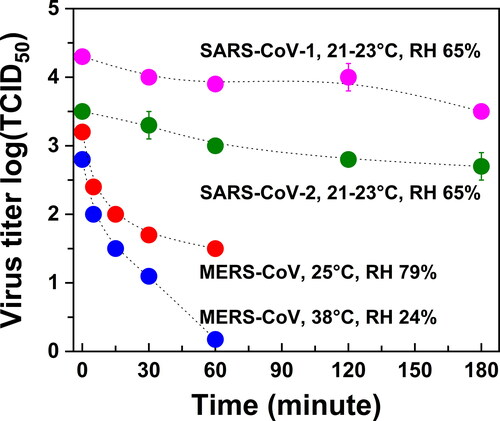
Air sampling in hospitals with infected individuals shows some differences among the three types of coronaviruses. For SARS-CoV-1, a number of studies in China have confirmed the widespread presence of viral RNA in both total suspended particles and size-separated particles in indoor hospital air (Du et al. Citation2005; Wei et al. Citation2005; Xu et al. Citation2005). As shown in , viable SARS-CoV-1 was also successfully isolated in the collected particles of various sizes using a cascade impactor (Xu et al. Citation2005). In addition, viral RNA was detected in filters and condenser water from air conditioners in hospitals. Viable SARS-CoV-1 in size-separated aerosols supports the theory of possible transmission via the “airborne” route in these scenarios. For MERS-CoV, both viral RNA and viable viruses were detected in total suspended particles (Kim et al. Citation2016). However, neither a pre-classifier nor a size separator was used for collecting these MERS-CoV air samples. Without removing or separating large particles, potential interferences of respiratory droplets cannot be ruled out.
Figure 2. Number of samples isolated with viable SARS-CoV-1 for various size particles collected in indoor hospital air. Data are from Xu et al. (Citation2005). Aerosols were separated into nine size ranges using a cascade impactor. For the smallest size range (<0.43 μm), particles were collected on filters.
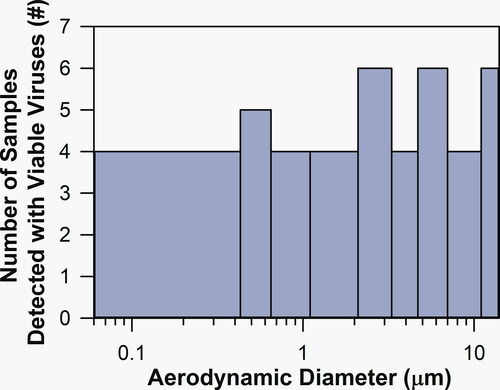
For SARS-CoV-2, the presence of viral RNA in total suspended particles was confirmed in indoor hospital air in China (Guo et al. Citation2020; Jiang et al. Citation2020; Liu et al. Citation2020), but with lower positive rates and lower abundance than for SARS-CoV-1 and MERS-CoV. Exceptions include some cases in intensive care units, where medical procedures such as tracheostomy and intubation can generate a significant amount of droplets and aerosols. The rather low abundance of SARS-CoV-2 in air makes size-separated sampling and analysis very challenging. A study using a cascade impactor has shown low abundance of viral RNA in size-separated particles (Liu et al. Citation2020). To date, no viable SARS-CoV-2 has been reported in real air samples. A study in Singapore reported that hospital air samples had no detectable viral RNA, despite the high abundance of viral RNA on personal protective equipment and surfaces in the hospital (Ong et al. Citation2020). However, a follow-up study in the same facility successfully detected viral RNA in the air using an improved sampling method (Chia et al. Citation2020). One study in the United States reported high positive rates and high abundance of viral RNA in both room air samples and hallway air samples from a medical center (Santarpia et al. Citation2020). However, in follow-up cell culture experiments, viable—that is, potentially infectious—virus was not yet detected in these air samples. Further complicating these studies is ongoing uncertainty about the exact extent to which face masks are able to reduce transmission of the virus. During the outbreak of COVID-19 in China, people were obligated to wear face masks when they were in public places, especially hospitals. However, the US study mentions that the effect of masks on their results can neither be assessed nor discounted because of inconsistent wearing patterns. In contrast to COVID-19, wearing face masks was not a nationwide intervention in China during the outbreak of SARS. For the time-being these real-world samplings indicate that “airborne” transmission of SARS-CoV-2, while plausible, is still far from proven, especially given the lack of viable viruses isolated from the collected particle samples.
It should be noted that in addition to the technical difficulties and time-consuming procedures involved in cell culture experiments to verify viability, the challenges to preserve natural viral states during sampling and analysis add additional difficulties in detecting infectious SARS-CoV-2. Such challenges underscore the need for new methods to in-situ examine viability in air. Experiments using animal models are helpful in testing “airborne” transmission of SARS-CoV-2 (Shi et al. Citation2020); however, caution should be taken to incorporate mechanistic aerosol-thinking during the experiment design. In addition to the long-range feature when traditionally defining “airborne” transmission (Lewis Citation2020), short-range person-to-person transmission of virus-containing aerosols also demands attention (Chen et al. Citation2020; Liu et al. Citation2017).
Virological assessment of infected individuals with COVID-19 shows that SARS-CoV-2 replicates in both upper and lower respiratory tracts, while SARS-CoV-1 and MERS-CoV develop infections only in the lower respiratory tract (Tellier et al. Citation2019; Zou et al. Citation2020). This difference explains why viable SARS-CoV-2 was successfully isolated in throat swabs whereas viable SARS-CoV-1 and MERS-CoV were not (Wölfel et al. Citation2020). The cleavage site at the S1-S2 junction in the SARS-CoV-2 spike protein might be responsible for this difference between SARS-CoV-2 and SARS-CoV-1 (Andersen et al. Citation2020; Walls et al. Citation2020). Furthermore, the peak concentration of SARS-CoV-2 shedding has been found to be over 1000 times higher than that of SARS-CoV-1 (Wölfel et al. Citation2020). This peak shedding concentration is reached much earlier in individuals with COVID-19 than it is in those with SARS—even before any symptoms or when they are still mild. These differences may have profound effects on the generation and the transmission of virus-containing respiratory droplets and aerosols. The presence or absence of mask intervention may add additional complexity.
Multidisciplinary collaborations are urgently needed to elucidate whether these emerging coronaviruses are aerosol-transmissible or not. Aerosol scientists have a vital role to play in such collaborations. Once airborne, viral aerosols will participate in typical atmospheric aerosol processes like dilution, evaporation, coagulation, and deposition. The exact dynamics will depend on the background air conditions and air movement. The role of aerosols in the spread of COVID-19 is unknown, but the potential for airborne transmission has been demonstrated (Liu et al. Citation2020; van Doremalen et al. Citation2020). The capacity and the threat posed by aerosols as vehicles for short- and long-range transmission of COVID-19 infection should therefore be carefully evaluated. The main research questions to be answered are: How long can SARS-CoV-2 aerosols stay airborne? What is the infectious potential of airborne SARS-CoV-2 aerosols? Particularly, what is the estimated infectivity of aerosols of different sizes emitted by individuals (including asymptomatic carriers) with different viral loads in their upper respiratory tract (Wölfel et al. Citation2020)? What is the minimum infectious dose for COVID-19? By making clearer the extent to which “airborne” transmission may or may not play a role in the spread of COVID-19, the answers to these critical questions will inform future measures to contain the pandemic.
State Key Laboratory of Environment Simulation and Pollution Control, School of Environment, Tsinghua University, Beijing, China
[email protected]
Yu Vincent Fu
State Key Laboratory of Microbial Resources, Institute of Microbiology, Chinese Academy of Sciences, Beijing, China
Li Liu
Department of Building Science, Tsinghua University, Beijing, China
Markku Kulmala
Institute for Atmospheric and Earth System Research/Physics, Faculty of Science, University of Helsinki, Helsinki, Finland
Acknowledgments
We would like to thank Dongbin Wang, Xue Li, and Jianguo Deng for preparing the figures and formatting the references.
References
- Andersen, K. G., A. Rambaut, W. I. Lipkin, E. C. Holmes, and R. F. Garry. 2020. The proximal origin of SARS-CoV-2. Nat. Med. 26 (4):450–2. doi: https://doi.org/10.1038/s41591-020-0820-9.
- Asadi, S., N. Bouvier, A. S. Wexler, and W. D. Ristenpart. 2020. The coronavirus pandemic and aerosols: Does COVID-19 transmit via expiratory particles? Aerosol. Sci. Technol. 54 (6):635–8. doi: https://doi.org/10.1080/02786826.2020.1749229.
- Chen, W., N. Zhang, J. Wei, H.-L. Yen, and Y. Li. 2020. Short-range airborne route dominates exposure of respiratory infection during close contact. Build. Environ. 176:106859. doi: https://doi.org/10.1016/j.buildenv.2020.106859.
- Chia, P. Y., K. K. Coleman, Y. K. Tan, S. W. X. Ong, M. Gum, S. K. Lau, S. Sutjipto, P. H. Lee, T. T. Son, B. E. Young, et al. 2020. Detection of air and surface contamination by Severe Acute Respiratory Syndrome Coronavirus 2 (SARS-CoV-2) in hospital rooms of infected patients. medRxiv. doi: https://doi.org/10.1101/2020.03.29.20046557.
- China NHC. 2020. COVID-19 prevention and control plan. 6th ed. Beijing, China: Disease Control and Prevention Bureau. http://www.nhc.gov.cn/jkj/s3577/202003/4856d5b0458141fa9f376853224d41d7.shtml.
- Drossinos, Y., and N. I. Stilianakis. 2020. What aerosol physics tells us about airborne pathogen transmission. Aerosol Sci. Technol. 54 (6):639–43. doi: https://doi.org/10.1080/02786826.2020.1751055.
- Du, Z., X. Wang, E. Dai, H. Liu, Y. Han, X. Pang, J. Zhai, R. Yang, Q. Wu, J. Li, et al. 2005. Examination of SARS coronavirus in air and air conditioner samples. Chin. J. Disinfect. 02:156–8. (in Chinese).
- Guo, Z.-D., Z.-Y. Wang, S.-F. Zhang, X. Li, L. Li, C. Li, Y. Cui, R.-B. Fu, Y.-Z. Dong, X.-Y. Chi, et al. 2020. Aerosol and surface distribution of Severe Acute Respiratory Syndrome Coronavirus 2 in hospital wards, Wuhan, China, 2020. Emerg. Infect. Dis. 27. doi: https://doi.org/10.3201/eid2607.200885.
- Jiang, Y., H. Wang, Y. Chen, J. He, L. Chen, Y. Liu, X. Hu, A. Li, S. Liu, P. Zhang, et al. 2020. Clinical data on hospital environmental hygiene monitoring and medical staffs protection during the coronavirus disease 2019 outbreak. medRxiv. doi: https://doi.org/10.1101/2020.02.25.20028043.
- Kim, S.-H., S. Y. Chang, M. Sung, J. H. Park, H. B. Kim, H. Lee, J.-P. Choi, W. S. Choi, and J.-Y. Min. 2016. Extensive viable Middle East Respiratory Syndrome (MERS) coronavirus contamination in air and surrounding environment in MERS isolation wards. Clin. Infect. Dis. 63 (3):363–9. doi: https://doi.org/10.1093/cid/ciw239.
- Leung, N. H. L., D. K. W. Chu, E. Y. C. Shiu, K.-H. Chan, J. J. McDevitt, B. J. P. Hau, H.-L. Yen, Y. Li, D. K. M. Ip, J. S. M. Peiris, et al. 2020. Respiratory virus shedding in exhaled breath and efficacy of face masks. Nat. Med. 26:676–80 doi: https://doi.org/10.1038/s41591-020-0843-2.
- Lewis, D. 2020. Is the coronavirus airborne? Experts can't agree. Nature 580 (7802):175. doi: https://doi.org/10.1038/d41586-020-00974-w.
- Liu, L., Y. Li, P. V. Nielsen, J. Wei, and R. L. Jensen. 2017. Short-range airborne transmission of expiratory droplets between two people. Indoor Air 27 (2):452–62. doi: https://doi.org/10.1111/ina.12314.
- Liu, Y., Z. Ning, Y. Chen, M. Guo, Y. Liu, N. K. Gali, L. Sun, Y. Duan, J. Cai, D. Westerdahl, et al. 2020. Aerodynamic analysis of SARS-CoV-2 in two Wuhan hospitals. Nature doi: https://doi.org/10.1038/s41586-0202271-3.
- Ong, S. W. X., Y. K. Tan, P. Y. Chia, T. H. Lee, O. T. Ng, M. S. Y. Wong, and K. Marimuthu. 2020. Air, surface environmental, and personal protective equipment contamination by Severe Acute Respiratory Syndrome Coronavirus 2 (SARS-CoV-2) from a symptomatic patient. JAMA 323 (16):1610. doi: https://doi.org/10.1001/jama.2020.3227.
- Pyankov, O. V., S. A. Bodnev, O. G. Pyankova, and I. E. Agranovski. 2018. Survival of aerosolized coronavirus in the ambient air. J. Aerosol. Sci. 115:158–63. doi: https://doi.org/10.1016/j.jaerosci.2017.09.009.
- Santarpia, J. L., D. N. Rivera, V. Herrera, M. J. Morwitzer, H. Creager, G. W. Santarpia, K. K. Crown, D. Brett-Major, E. Schnaubelt, M. J. Broadhurst, et al. 2020. Transmission potential of SARS-CoV-2 in viral shedding observed at the University of Nebraska Medical Center. medRxiv. doi: https://doi.org/10.1101/2020.03.23.20039446.
- Shi, J., Z. Wen, G. Zhong, H. Yang, C. Wang, B. Huang, R. Liu, X. He, L. Shuai, Z. Sun, Z. Bu, et al. 2020. Susceptibility of ferrets, cats, dogs, and other domesticated animals to SARS–Coronavirus 2. Science eabb7015. doi: https://doi.org/10.1126/science.abb7015.
- Tellier, R., Y. Li, B. J. Cowling, and J. W. Tang. 2019. Recognition of aerosol transmission of infectious agents: A commentary. BMC Infect. Dis. 19 (1):101. doi: https://doi.org/10.1186/s12879-019-3707-y.
- van Doremalen, N., T. Bushmaker, D. H. Morris, M. G. Holbrook, A. Gamble, B. N. Williamson, A. Tamin, J. L. Harcourt, N. J. Thornburg, S. I. Gerber, et al. 2020. Aerosol and surface stability of SARS-CoV-2 as compared with SARS-CoV-1. N. Engl. J. Med. 382 (16):1564–7. doi: https://doi.org/10.1056/NEJMc2004973.
- van Doremalen, N., T. Bushmaker, and V. J. Munster. 2013. Stability of Middle East Respiratory Syndrome Coronavirus (MERS-CoV) under different environmental conditions. Eur. Surveill. 18 (38), pii: 20590. doi: https://doi.org/10.2807/1560-7917.ES2013.18.38.20590.
- Walls, A. C., Y.-J. Park, M. A. Tortorici, A. Wall, A. T. McGuire, and D. Veesler. 2020. Structure, function, and antigenicity of the SARS-CoV-2 spike glycoprotein. Cell 181 (2):281–92. doi: https://doi.org/10.1016/j.cell.2020.02.058.
- Wei, W., J. Li, W. Xiao, M. Wang, J. Wang, and Y. Xin. 2005. Detection of SARS coronavirus RNA in air samples from the sickroom and out-sickroom of Xiaotang-hill Hospital. Chin. J. Health Lab. Technol. 15:648–51. (in Chinese).
- WHO. 2020. Q&A on coronaviruses (COVID-19). Accessed May 1, 2020. https://www.who.int/news-room/q-a-detail/q-a-coronaviruses.
- Wölfel, R., V. M. Corman, W. Guggemos, M. Seilmaier, S. Zange, M. A. Müller, D. Niemeyer, T. C. Jones, P. Vollmar, C. Rothe, et al. 2020. Virological assessment of hospitalized patients with COVID-2019. Nature. doi: https://doi.org/10.1038/s41586-020-2196-x.
- Xu, Q., Q. Fan, N. Duan, W. Wang, and J. Chen. 2005. Airborne spread pathway in intensive care unit (ICU) of specialized SARS hospital. Chin. J. Nosoconmiol. 12:1380–2. (in Chinese).
- Zou, L., F. Ruan, M. Huang, L. Liang, H. Huang, Z. Hong, J. Yu, M. Kang, Y. Song, J. Xia, et al. 2020. SARS-CoV-2 viral load in upper respiratory specimens of infected patients. N. Engl. J. Med. 382 (12):1177–9. doi: https://doi.org/10.1056/NEJMc2001737.