Abstract
Atmospheric aerosols containing ammonium sulfate have several morphological types in the solid phase. Laboratory experiments were used to reproduce the particle shapes commonly found in atmospheric samples to elucidate factors affecting these different particle shapes. Ammonium sulfate particles and mixed particles with oxalate were prepared under different relative humidity (RH) conditions and were observed using transmission electron microscopy (TEM). Most ammonium sulfate particles dried using a diffusion dryer after generation from aqueous solution were spherical or clusters of spherical units. However, after exposure of the TEM samples to 59% RH and 75% RH at atmospheric pressure, some particles changed to a rotundate-rectangular shape with parallel straight lines as the particle perimeter. Number fractions of particles that had changed to rotundate-rectangular shape from a spherical shape at 59% RH and 75% RH were, respectively, 6% and 52% at 3 h, and 18% and 66% at 1 day. Morphological changes during the experiment suggest that ammonium sulfate crystallization occurred under metastable RH. Morphological changes to rotundate-rectangular shapes were also found for ammonium sulfate co-existing with oxalate. These results indicate that spherical or complexly shaped sulfate-containing aerosols can change gradually to shapes having more regular structures according to a particle’s experienced RH condition. Both spherical and rotundate-rectangular shapes of sulfate particles have often been found in atmospheric aerosol samples. The morphological change of ammonium sulfate found in this study will be useful to infer the optical properties of atmospheric particles and the thermodynamic process on a solid particle surface.
EDITOR:
1. Introduction
Atmospheric aerosol particles have a myriad of morphologies. Aerosol morphology, in addition to size and chemical composition, is an important factor defining the optical properties of particles (Moosmüller, Chakrabarty, and Arnott Citation2009; Adachi, Chung, and Buseck Citation2010). Morphological properties of aerosols often reflect particles’ source, composition, formation processes, and experiences in terms of meteorological and atmospheric conditions. Different light-scattering properties of spherical and nonspherical particles have been useful for classifying some species and sources of particles after remote sensing and optical measurement (Kokhanovsky Citation2012; Liu and Mishchenko Citation2020). Relations between light scattering and particle shape are used increasingly and with greater precision for developing new optical counting techniques that require distinction of particle species (Kobayashi et al. Citation2014; Nakagawa et al. Citation2016). For estimating aerosol effects on climate using climate models, complex mixing states of aerosol particles such as soot, sulfates, and organics have been increasingly considered (e.g., Ma, Yu, and Luo Citation2012; Riemer and West Citation2013; Scarnato et al. Citation2013; Matsui Citation2016a, Citation2016b; Riemer et al. Citation2019). However, because of the hygroscopicity of secondary aerosols, submicrometer aerosol particles still tend to be simplified and treated as spherical or droplet particles in many measurements and climate models. Such assumptions are not always suitable. Incorporating aspects of submicrometer aerosol morphology into optical measurements and climate modeling requires better understanding of the fundamental morphologies of atmospheric major aerosol components and the changes they undergo under general relative humidity (RH) conditions. Such knowledge remains insufficient.
For atmospheric submicrometer sulfate-rich particles, numerous studies have used electron microscopy to assess samples collected at various sites. The samples’ particle shapes were not uniform. In some studies, dome-like sulfate-rich particles have been observed on support films for electron micrographs. Results of elemental analyses of individual particles and atmospheric conditions suggest that shapes on films are related with the degree of neutralization of the sulfate (Waller, Brooks, and Cartwright Citation1963; Frank and Lodge Citation1967; Gras and Avers Citation1979; Bigg Citation1980; Ferek, Lazrus, and Winchester Citation1983) and with the mixing states with organics (Fu et al. Citation2012; Mayama et al. Citation2013; Li et al. Citation2016a, Citation2016b; Wang et al. Citation2018). However, particles can deliquesce with increased humidity in a low-pressure sampler and during treatment or retention of samples. For that reason, dome-like shapes on samples do not always indicate that the particles existed as liquid phase in the atmosphere. Multiple sulfate-rich particles collected on samples as solid-phase particles have also been reported (Li et al. Citation2003; Li, Shao, and Buseck 2010; Ueda, Osada, and Takami Citation2011; Ueda et al. Citation2016). Their shapes are roughly classifiable into three types: ball-like, rotundate-rectangular (RR, designated as cylindrical, cocoon-like or hexagonal in other studies) having direction regularity, and clustered particles of balls or RR-shaped units. The clustered particle shape suggests that it is the result of particle coagulation. For ball-like and RR-shaped sulfate-rich particles, it remains unclear why atmospheric particles have different morphology.
Particles of these three types have been found on samples under high-concentration conditions of ammonium, sulfate, and organic materials (Ueda, Osada, and Takami Citation2011; Ueda et al. Citation2016). Ammonium sulfate, which is formed by neutralization of sulfuric acid with ammonia, is a major atmospheric aerosol component of secondary aerosols (Seinfeld and Pandis Citation2006). A solution of ammonium sulfate is metastable in 35–80%RH at 0–40 °C (Xu et al. Citation1998). Droplet particles of ammonium sulfate are known to effloresce at the efflorescent relative humidity (ERH) of 35%RH with decreased RH, but the dried particles remain as solid phase until deliquescent relative humidity (DRH) at 80%RH with increased RH. In other words, ions composing the particle in metastable RH are insufficiently stable. Consequently, such conditions might affect the particle shape. The relation between particle phase and RH for particles containing ammonium sulfate has been checked using several methods, including observation using RH-controlled environmental TEM/SEM (Ebert, Inerle-Hof, and Weinbruch Citation2002; Matsumura and Hayashi Citation2007; Hiranuma et al. Citation2008; Treuel, Pederzani, and Zellner Citation2009; Adachi, Freney, and Buseck Citation2011; Bai et al. Citation2018). Nevertheless, earlier studies have mostly emphasized examination of particle size and change around the DRH and the ERH. Moreover, the observable changing time and environment (such as temperature and pressure) have been limited for many experimental systems. Reports of earlier studies have rarely described morphological changes occurring slowly at near-atmospheric conditions.
To elucidate production mechanisms of sulfate particle shapes that are often found in atmospheric aerosol samples, this study investigated two important points: the relation between ammonium sulfate particle morphology and ambient RH, and the effects on that relation which occur in mixing with organics. Ammonium sulfate particles and mixed particles with oxalate prepared in different humidity conditions were observed using transmission electron microscopy (TEM). First, the solid particles were generated by a nebulizer and a diffusion dryer. They were subsequently exposed at RHs that prevailed in their metastable states at atmospheric pressure. Specific attention was devoted to the formation of RR-type particles from spherical particles from the perspective of effects on optical properties.
2. Reproduction target and experiment method
2.1. Setting target morphology and materials based on typical atmospheric solid sulfate particles
and present an example of atmospheric sulfate particles, which are the reproduction target examined for this study. After these particles were collected in Okinawa, Japan (Ueda, Osada, and Takami Citation2011), they were coated with a Pt/Pd alloy at a shadowing angle of 26.6° (arctan 0.5) to give stereoscopic information about the particles from 2D TEM images. Type 1 particles had a ball-like shape. Type 2 particles were RR-shaped, having parallel straight lines as the particle perimeter. Type 2 particles had various aspect ratios. Type 3 were clustered-type particles, which were connected to form numerous spherical or RR units. Strong contrast with the film and long shadows of Pt/Pd for these types 1, 2, and 3 particles indicate that they had comparable height to that of the projected area. These findings suggest that they were collected as solid particles on the film. The number fractions of 0.2–0.7 µm diameter types 1, 2, and 3 particles from the Okinawa site were, respectively, 1–67%, 0–45%, and 0–46%.
Figure 1. Electron micrographs (a, b) and SAED patterns (c, d) for particles collected at Okinawa, Japan (Ueda et al. Citation2011). Types 1, 2, and 3, respectively, show single-ball type, RR-shaped type, and clustered type. Panels (c) and (d), respectively, show typical SAED patterns for types 1 and 2 particles.
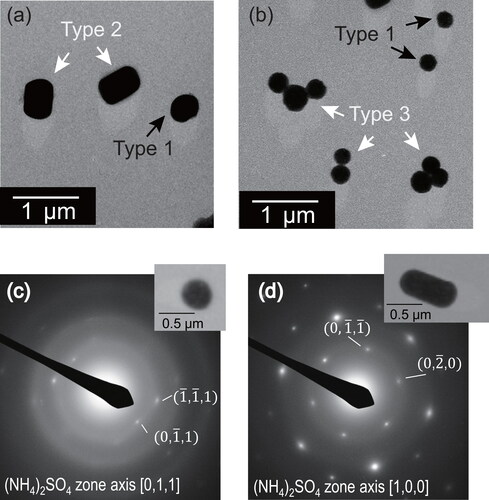
Several studies of aerosols in urban regions have shown type 2 sulfate particles (Coz et al. Citation2008; Shi et al. Citation2008; Li, Shao, and Buseck 2010). Others have found those in an Asian outflow (Ueda, Osada, and Takami Citation2011, Ueda et al. Citation2016), and from biomass burning (Li et al. Citation2003). Among the general atmospheric aerosol components, sea salt particles composed mainly of sodium chloride have also been found as crystals, but they are usually cubic and of super-micrometer size (Pósfai et al. Citation1995; Ueda et al. Citation2016, Citation2018). The type 2 sulfate particle shape differs from that of sea salt because the former usually have a longer direction with various aspect ratios. Although KCl and K2SO4 particles generated in the laboratory have also been reported as cylindrical (Freney et al. Citation2009), edges of type 2 sulfate-rich particles on atmospheric aerosols tended to be rounder.
For types 1 and 2 particles collected at the Okinawa site, selected area electron diffraction (SAED) patterns obtained by electron microscopy are depicted in . Although submicrometer sulfate particles are easily volatilized by exposure to intense high-density electron beams from SAED, the SAED pattern is obtainable before evaporation if all handling is done rapidly. For type 1 particles, the patterns often matched that for (NH4)2SO4: unit cell with a = 7.78, b = 10.64, c = 5.99, orthorhombic. However, weak-intensity spots and concentric circles suggest that the particle contains crystallites having plural directions. Such type 1 particles might be aggregates of small ammonium sulfate crystallites or mixed particles with other materials. For type 2 particles, SAED patterns were usually identified as clear periodic spot patterns matching that for (NH4)2SO4. The SAED pattern and particle shape of type 2 suggest that ammonium sulfate ions had regular alignment in whole particles as crystals.
Particle shapes and the SAED analysis results imply that each type of particle contains ammonium sulfate, although they can have different structures. Therefore, this study first examined replication methods of types 1, 2, and 3 particles using only ammonium sulfate under various RH conditions. However, particles of the three types have been found in atmospheric aerosol samples when the mass of organics was comparable to those of sulfate and ammonium (Ueda, Osada, and Takami Citation2011; Ueda et al. 2016). To investigate effects of one organic component of atmospheric aerosols on the morphological features of ammonium sulfate, the morphology of mixed particles with oxalate was observed for this study. Oxalic acid, a compound that is particularly abundant among water-soluble organic compounds in atmospheric aerosols (e.g., Cao et al. Citation2017; Kawamura et al. Citation2017), reportedly has strong correlation with aerosol sulfate (Yu et al. Citation2005).
2.2. Preparation of solid particles from a liquid solution
A schematic diagram of the experimental apparatus used to collect sample particles on the TEM grid is presented in . Droplet particles were generated from an ammonium sulfate solution (1% by mass) using a nebulizer. Particles in the sample air were then collected using a two-stage cascade impactor (50% cutoff aerodynamic diameters of the two stages were 1 and 0.3 µm at a flow rate of 0.7 L min−1) on the TEM grid in line B, after drying with dry air and a diffusion dryer with silica gel. This study analyzed samples from the second stage. Aerosol samples were collected for 10–15 s. Ammonium sulfate particles and particles mixed with oxalate were collected on carbon-coated nitrocellulose (collodion) films over a Cu TEM grid (H7, 400 mesh, Reference Finder Grids; Maxtaform).
Figure 2. Schematic diagram of the experiment setup used to collect sample particles on the TEM grid.
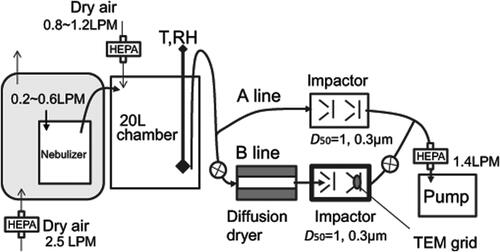
Hygroscopic particles including ammonium sulfate have different size depending on the ambient humidity. For pure ammonium sulfate, liquid particles effloresce at 35%RH at 0–40 °C (Xu et al. Citation1998). The particle diameter immediately before efflorescence is 1.1 times that of the solid particle (Seinfeld and Pandis Citation2006). The particles generated from the nebulizer include a much larger mass of liquid water than those of particles in equilibrium with the pressure of ambient water vapor. Therefore, drying of such droplet particles using a diffusion dryer is expected to alter the particle phase more rapidly than the phase change in atmospheric aerosols. For this study, with consideration that the drying speed might affect particle crystallization and morphology, the RH in a 20 L chamber before induction to the diffusion dryer was monitored using a humidity meter (TR-77Ui; T&D Corp., Japan). It was controlled by the addition of clean dry air. The residence time in the chamber was calculated as about 14 min at 1.4 L min−1. Before sampling at line B, the RH in the sampling system was kept stable by maintaining the flow using valves and line A. The TEM samples were stored under dry conditions of less than 10%RH at room temperature (ca. 27 °C) until TEM analyses or exposure under metastable humidity conditions, as explained below. Experiment conditions used analyze samples for this study are presented in .
Table 1. Experimental conditions of TEM samples.
Using the same system, mixed particles with oxalate were also generated from solution. A mixed solution was formed from oxalic acid dihydrate and ammonium sulfate. According to measurement of hygroscopicity using a tandem differential mobility analyzer (Prenni, DeMott, and Kreidenweis Citation2003), the DRH at a mass ratio of 10:1 for (NH4)2SO4:(COOH)2 was 77%RH. They also reported that the DRH at the mass ratio of 3:2 was not observed because the particles could not be sufficiently dried by passage through a diffusion dryer, suggesting that oxalate has water retention properties. Considering the probabilities of different effects on the morphology by the mixing ratio, mixed particles for this study were generated from two solutions at the respective mass ratios of 9:1 and 3:2 for (NH4)2SO4:(COOH)2. The concentrations of the total solute were 3.4% (by mass) for the 9:1 mixed solution and 1.8% (by mass) for the 3:2 mixed solution.
2.3. Exposure to metastable humidity conditions at atmospheric pressure
Dried particles on TEM grids prepared using the method presented in subsection 2.2 were exposed to metastable humidity conditions to elucidate the relation between the solid particle shape and RH. The particles were observed before and after exposure using TEM. Particles collected on TEM grids were kept with the collection side of the grid upward in a 590 mL airtight box with controlled and constant RH at room temperature (ca. 27 °C) for 3 h, 1 day or 3 days. The RH, temperature, and pressure in airtight box were measured using data loggers (TR-73U; T&D Corp., Japan). Saturated solutions of NH4NO3 and NaCl were used to control RH in the box. The solution surfaces for NH4NO3 and NaCl, respectively, match water vapor at 61.8%RH and 75.3%RH at 25 °C (Tang and Munkelwitz Citation1993). The measured RHs in the airtight box with NH4NO3 and NaCl were, respectively, 59.3 ± 0.6%RH and 75.0 ± 0.8%RH at temperature and pressure of approximately 27 °C and 1003 hPa (). After exposure, TEM samples were stored under dry conditions of less than 10%RH until TEM analyses.
2.4. TEM analysis
Particles on the TEM grid were photographed using TEM (JEM 2100-plus; JEOL, Japan) at 1500× or 6000× magnification with 200 kV accelerating voltage. Under TEM observation in these settings, no clear damage or morphological change with irradiation of the electron beam and photographing was observed for the analyzed particles. Elemental mapping was done using energy-dispersive X ray spectrometry (EDS EX-24200M1G2T; JEOL Ltd., Japan) with TEM in scanning TEM (STEM) mode and 200 kV accelerating voltage. The elemental mapping data were sampled and analyzed using software (NSS 3; Thermo Fisher Scientific Inc., USA).
The TEM images were processed using image analysis software (Win Roof; Mitani Corp., Japan) to estimate shape parameters. The projection area (A), perimeter (P), maximum length passing through the center of gravity (Lmax), and minimum length passing through the center of gravity (Lmin) were measured using the image analysis software. Using these measured values, the circle equivalent diameter (D = 2(A/π)1/2), circularity factor (CF = 4πA/P2), and ratio of maximum to minimum length (Rmax/min= Lmax/Lmin) were estimated. Details of image analysis and shape parameter estimation are described in the online supplementary information (SI) (S1).
Particles of each morphological type were identified for this experiment following simple rules: ball-like particles (type 1) consist of a single unit not having a specific direction; RR shaped particles (type 2) consist of a single unit having parallel straight lines for the particle perimeter; and clustered particles (type 3) are numerous, mutually connected units of type 1 or 2. All three types have a strong contrast with the film. This simple rule is applied to particles of known composition in this experiment. For ammonium sulfate particles found in atmospheric aerosols, classifying similar-looking particles such as those described earlier in subsection 2.1 according to the type of sample air is recommended.
3. Results and discussion
3.1. Solid ammonium sulfate particles generated from a liquid solution
The TEM images presented in depict ammonium sulfate particles when the 20 L chamber was held at 95%RH and 37%RH before induction into a diffusion dryer using the methods described in subsection 2.2 (respectively, samples A-5 and A-1 in ). Most particles for the 95%RH in chamber were found to be ball-type (type 1) and clustered balls (type 3). Because the particles accumulated on the films were so numerous and because they could be observed with the naked eye despite the short sampling time (ca. 15 s), cluster particles were regarded as formed by coagulation in the dryer or on the TEM grid. In the sample, RR-shaped (type 2) particles were scarce (2.9% of 104 unclustered particles). As shown for atmospheric aerosols in subsection 2.1, ball-shaped ammonium sulfate particles suggest an aggregate of randomly oriented crystallites, whereas type 2 is crystalline. The considerable difference of RHs inside the 20 L chamber (95%) and in the diffusion dryer can cause rapid formation of multiple crystallites in a particle. For sample A-1, the RH change was much less with the chamber controlled to 37%RH, close to ERH (35%). However, most particles of sample A-1 were also ball-type and cluster-type (). The fraction of RR-shaped particles was also small (3.2% of 220 unclustered particles), similar to the sample for 95%RH. Although five samples exposed to different RHs in the chamber from 37% to 95% (samples A-1 to A-5 in ) were also observed, no clear trend with RH was found. Probably, changes of RH in the real atmosphere occur more slowly than those in the system. Therefore, the system might not replicate the sensitive change of morphology during drying of droplet particles in the atmosphere. However, at least, this result suggests that RR-shaped ammonium sulfate particles are not usually formed by drying with diffusion dryers.
3.2. Morphological change under metastable RH conditions at atmospheric pressure
shows morphological differences of ammonium sulfate particles found for sample regions before and after exposure at 75%RH for 1 day using the method explained in subsection 2.3. Morphological change was found for most particles. For cluster-type particles before exposure, as indicated by arrows A and C in , multiple ball units became well connected, forming an RR shape with parallel straight lines for the particle perimeter. Most double-ball clustered particles changed to RR-shaped particles having a long direction, as indicated by an arrow C in . For many ball-like particles, as indicated by an arrow B in , the parallel straight lines appeared more clearly after exposure at 75%RH. However, the aspect ratio of the particle before the exposure was mostly retained. RR-shaped particles were found after exposure at 75%RH, irrespective of areas with and without TEM observation before exposure.
Figure 4. Electron micrographs of ammonium sulfate particles before (a) and after (a’) exposure to 75%RH for one day at atmospheric pressure for the same sample region. White arrows indicate particles that were found after exposure to have changed shape.
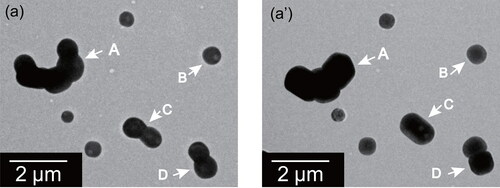
3.2.1. Relation of morphological change to exposure RH and time
portrays electron microphotographs taken at equal magnification before and after exposure at 59%RH and 75%RH for 3, 24, and 72 h. Morphological changes of particles were clear for exposed samples at 75%RH for 3 h. Although morphological changes of particles were less clear for samples exposed at 59%RH for 3 h, minor changes of ball units were found, as presented in . For double-ball clustered particles and ball-type particles, number fractions of particles that changed to RR shape were estimated for each exposure time and RH. They are depicted in . The fraction of changed double-ball clustered particles includes only particles changed to single RR or single ball; particles for which each ball changed to RR, as indicated by an arrow D in , were uncounted. Number fractions were estimated from 69 to 126 ball-type particles that had 0.2–1.2 µm equivalent circle diameter and from 34 to 52 double-ball-type particles that had 0.6–1.5 µm equivalent circle diameter. For samples exposed at 59%RH, particles that had changed to RR shape within 3 h were few (<6%), but 18% of ball-type particles and 15% of double-ball-type particles were found to have changed after one day. For samples exposed at 75%RH, about half of the particles (52% and 43%, respectively, for ball-type particles and double-ball-clustered particles) changed to RR shape within 3 h. The fractions, respectively, reached 66% and 69% at one day. Particles which changed from double-ball to single-ball decreased from 24 h to 72 h, implying that such single balls also transited to single RR under a humidified condition.
Figure 5. Electron micrographs of ammonium sulfate particles before (a–f) and after (a’–f’) exposure under metastable RH conditions at atmospheric pressure for the same sample region. Panels (a’), (b’), and (c’), respectively, show particles after exposure at 59%RH for 3 h, 1 day, and 3 days. Panels (d’), (e’), and (f’), respectively, portray particles after exposure at 75%RH for 3 h, 1 day, and 3 days. White arrows indicate examples of particles or components that change shape, as found after exposure.
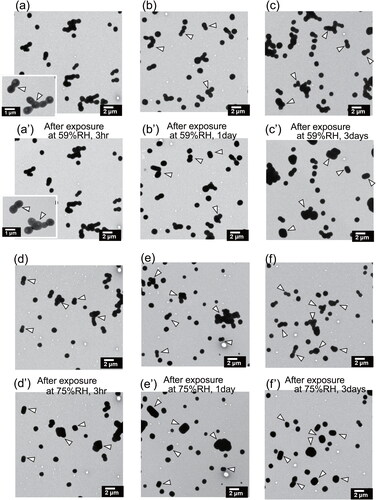
3.2.2. Change of shape parameters
exhibits scatter plots of shape parameters (circle equivalent diameter D, circularity factor CF, and the ratio of maximum length to minimum length Rmax/min) before and after exposure at 75%RH for 1 day, for ball-type particles and double-ball-clustered particles before exposure. The D values before exposure were almost equal to those after exposure (). For ball-type particles before exposure, both CF and Rmax/min () were approximately 1.0 before exposure (respectively, 0.95 and 1.14 on average), and were the same after exposure (respectively, 0.95 and 1.14 on average). For particles changed to RR-type from ball-type after exposure, the Rmax/min tended to increase slightly along with the morphological change (respectively, 1.13 before the exposure and 1.16 after the exposure, on average). Although some change of CF and Rmax/min by the morphological change to the nonspherical shape was expected, the changed shapes of ball-like particles were rotundate square rather than rotundate rectangular. The CF and Rmax/min of the rotundate square are close to 1. Therefore, the expected changes of their parameters were slight unless the shape before exposure was completely spherical. For double-ball clustered particles before exposure, the CF values for particles that changed to the RR-shape approached 1.0 after exposure, with good connection of the balls (). Although the Rmax/min for particles that had changed to become RR-shaped () decreased after exposure, the values remained at around 1.2–1.5 (1.3 on average) because elongate crystal particles were formed. The Rmax/min values after exposure of particles that had changed from double-ball to RR-shaped were larger than those of particles that had changed from ball-like particles to RR-shaped, suggesting that the aspect ratio of particles after morphological change depends on the particle shape before the change.
Figure 7. Shape parameters before and after exposure at 75%RH for one day for ball-type particles and double-ball clustered particles before exposure: (a) circle-equivalent diameters D, (b) circularity factor CF, and (c) ratio of maximum length to minimum length Rmax/min. Numbers in brackets in the legend are the numbers of analyzed particles.
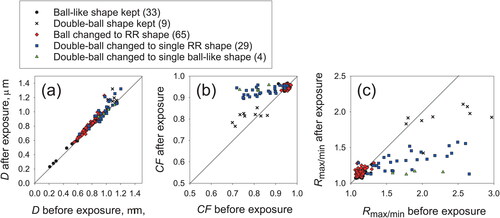
3.2.3. Inference of how morphology changes
Both 59%RH and 75%RH are lower than the DRH of ammonium sulfate (80%RH) but higher than its ERH (35%RH). Morphological change in this experiment indicates that crystallization of ammonium sulfate occurred at metastable RHs less than the DRH. Solid ammonium sulfate particles are generally regarded as remaining in the solid phase under metastable RH. However, particles examined for this study were exposed under metastable RH in a box over a long time. To explain the morphological change phenomena occurring in the box, one must consider the particle phase carefully. Under metastable RH conditions, hygroscopic particles have two conditions for equilibrium to water vapor, as liquid and solid. First, an assumption made for this study is that the morphologically changed particles have reached equilibrium as liquid during long exposure at metastable conditions in the box. At 75%RH, the particle diameter is increased by 1.4 times compared to that in a dried condition; its volume is increased 2.7 times (Tang and Munkelwitz Citation1994). The collodion film on the TEM grid used for this study is a semipermeable membrane. Some traces of droplets are expected to remain on the film if the droplet size has increased. However, dome-shaped particles and particles having any other trace on the film were not found in samples after exposure. Therefore, it is difficult to infer that particles have reached a condition in equilibrium as liquid. As explained in subsection 3.1, RR-shaped particles were difficult to form by drying from droplets. Clustered particles changed after exposure, but they retained a certain shape, as particles indicated by an arrow A in . These results also suggest that the conditions of particles in the box were close to those for equilibrium as a solid rather than as a liquid. However, CF and Rmax/min values of changed double-ball cluster particles (blue squares and green triangle in ) clearly differed from those of particles retaining a double-ball shape (black x). Absence of the intermediate CF and Rmax/min values between them suggests that the morphological change of the former particles occurred rather rapidly. This change is inexplicable solely by the solid phase. Morphological changes proceeded for some fraction of particles rather than for all particles. That fraction increased with exposure time. Such probabilistic occurrence of changes implies that the changes might be related to equilibrium fluctuation. Water vapor absorbs and evaporates continuously onto the particle surface to create overall balance, but temporary disequilibrium. With slight absorption of water vapor on the particle surface, ammonium sulfate might be able to transfer ions in the high-concentration solution. Although the layers of liquid saturated solution might revert to a solid state by evaporation, some particles can also change gradually to a preferred crystalline structure by repeat temporary water absorption. The fraction of morphologically changed particles in the sample exposed at 75%RH was much higher than in the sample exposed at 59%RH for the same duration (). Higher RH means higher water vapor pressure on the solid particle surface, making frequent water absorption and consequent morphological change occur readily.
3.3. Mixed particles of ammonium sulfate and oxalate
, respectively, present mixed particles at mass ratios of 9:1 and 3:2 for (NH4)2SO4:(COOH)2, as prepared using the method described in subsection 2.2. Ball-like and clustered particles were often found in the samples. Some thin parts often having lath-like shape enclosed in ball-like particles were found as indicated by black arrows. Based on results of EDS analysis, the detection signal of carbon for such thin parts is stronger than the background value, but that of sulfur was weaker ( of SI), suggesting that such parts are oxalate-rich. Some researchers have used theory and experimentation to examine the morphology and liquid–liquid phase separation in internal mixed particles of ammonium sulfate and organics (Zelenay et al. Citation2011; Song et al. Citation2012; Veghte, Bittner, and Freedman Citation2014; Altaf, Zuend, and Freedman Citation2016). Song et al. (Citation2012) used optical microscopy and Raman spectroscopy to observe complex mixtures of liquid-phase atmospherically relevant dicarboxylic acids mixed with ammonium sulfate. They reported different occurrences of phase separation for different ratios of carbon, ammonium sulfate, and water by samples exposed to cycles of drying and moistening. Veghte, Bittner, and Freedman (Citation2014) used cryo-TEM to characterize the morphology of dried submicrometer particles of ammonium sulfate mixed with carboxylic acids. Their mixed particles were generated from aqueous solution and were subsequently dried rapidly using a diffusion dryer. The results of their study suggest a tendency by which the particle morphology is dependent on the water solubility of organics and the particle size. Homogeneous morphologies were found for mixed particles with highly soluble organic and partially engulfed structure for mixed particles with less soluble organic or larger particles. Results of this study revealed particles of two types (containing the thin lath-like part or homogeneous) on samples of mixed particles of ammonium sulfate and oxalate. The fractions of particles having thin parts were larger in the 3:2 mixed particles (29% of particles smaller than 1 µm) than in the 9:1 mixed particles (7% of particles smaller than 1 µm).
Figure 8. Electron micrographs of mixed particles of ammonium sulfate and oxalate at weight ratios of (a) 9:1 and (b) 3:2. Black arrows indicate thin parts having lath-like parts, regarded as oxalate.
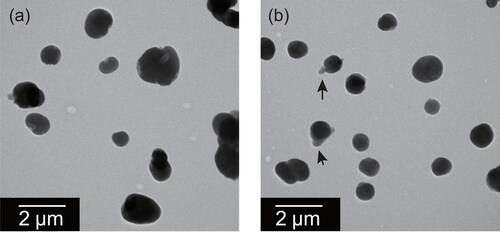
present mixed particles after exposure at 75%RH for one day. To avoid electron-beam damage to the oxalate, no sample was observed before exposure. Compared to samples without exposure at 75%, RR-shaped units were often found in particles after exposure at 75%RH. Particles composed of an RR-shaped unit and a thin part were also found, such as particles indicated by black and white arrows in .
Figure 9. Electron micrographs of mixed particles of ammonium sulfate and oxalate at the weight ratio of (a) 9:1 and (b) 3:2 after exposure at 75%RH for one day. White arrows indicate parts having RR shape. Black arrows indicate thin parts, regarded as oxalate-rich.
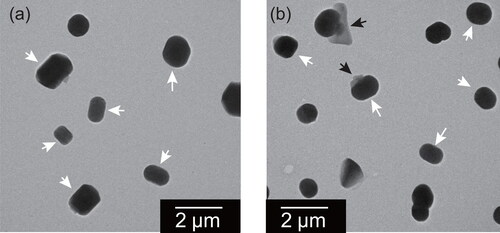
presents the number fractions of particles of the respective types for samples without exposure and after exposure for smaller particles (<1 µm, for which clustered particles were fewer). The fraction of particles having a thin part (ball + thin part and RR + thin part) after exposure (C-2 and D-2) was similar to that of particles without exposure (C-1 and D-1). The fraction of particles having RR shapes tended to be larger in samples after exposure (C-2 and D-2), irrespective of whether they had or did not have thin parts. The total fraction of particles containing RR-shaped parts (RR and RR + thin part) for 9:1 mixed particles (59%) was larger than that (46%) for 3:2 mixed particles. However, these fractions did not decrease greatly compared to the fraction (66%) of changed ammonium sulfate particles from a single ball to single RR, as shown in subsection 3.2.
Figure 10. Number fractions of the respective morphological types for samples of mixed particles without exposure and after exposure. The fraction was estimated for particles smaller than 1 µm in circle-equivalent diameters. Numbers above the columns are numbers of analyzed particles.
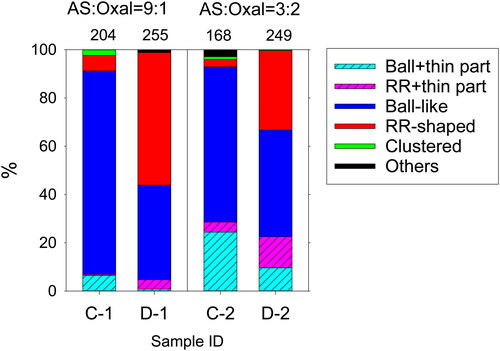
These results obtained for mixed particles with oxalate revealed that ammonium sulfate in such mixed particles can leave properties of change almost unaltered, giving a preferred structure under metastable RH conditions. Although atmospheric aerosols are expected to have more complex composition, similar RR-shaped sulfur-rich units attached with organics or soot have often been found in atmospheric aerosol samples (Li, Shao, and Buseck 2010; Ueda, Osada, and Takami Citation2011; Li et al. Citation2016b; Ueda et al. Citation2016; Xu et al. Citation2017). The morphology of spherical or complexly shaped atmospheric sulfate-containing aerosols might change to a more regular shape when experiencing metastable conditions. This finding is particularly important for evaluating the optical properties of particles and for evaluating the thermodynamics on a particle surface.
4. Summary and conclusions
To reproduce the particle shapes which are often found in atmospheric aerosol samples, ammonium sulfate particles and mixed ammonium sulfate-oxalate particles prepared at different RH conditions were observed using TEM. Most solid particles dried with a diffusion dryer were ball-type or cluster-type. However, after their exposure in their metastable states at 59%RH and 75%RH, ammonium sulfate particles changed to RR shapes from ball and cluster shapes. That morphological change suggests that ammonium sulfate crystallization can occur under metastable RH. Morphological change occurring below a 75%RH condition was noticeable even during 3 h exposure. Differences in the number fractions of particles that had changed to RR shapes found at 59%RH and 75%RH suggest that morphological change occurs more easily at RH closer to the DRH. Such morphological change was also found for ammonium sulfate mixed with oxalate.
Although RR-shaped ammonium sulfate particles have long been found in atmospheric samples, the reasons for their different shapes were insufficiently clarified. This reproduction experiment revealed that RR-shaped ammonium sulfate can be formed at atmospheric pressure through morphological change occurring at metastable RHs. Additionally, they formed only to a slight degree by drying droplet particles in this study, even from sample air of 37%RH, suggesting a lower probability that RR-shaped particles form by rapid drying in a diffusion drier. The observed change of ammonium sulfate to RR shape on samples first suggests that the sample for morphological evaluation must be kept in a dry condition and treated under suitable RH conditions until morphological observations are made. It is recommended that treatment outside of the well-dried storage container be completed quickly under RH that is sufficiently lower than 59% because this experiment showed that morphological changes occurring under 59%RH within 3 h were minor. If RR-shaped particles occur in a sample that has been stored and treated appropriately under dry conditions, then the RR-shaped particles can be expected to be present in the atmosphere. Conditions of 59–75%RH, for which morphological changes of ammonium sulfate were observed, often occur in the atmosphere. Results obtained from this study suggest that spherical or complexly shaped sulfate-containing aerosols can change gradually to more regular structures in the atmosphere according to a particle’s experienced RH condition. Mixing states, including morphological features, must be increasingly considered for optical properties, climate effect evaluation, and atmospheric aerosol measurements. Morphological changes of solid ammonium sulfate in particles must be noted as a record of experienced phases of the particles in the atmosphere.
Supplemental Material
Download MS Word (602.6 KB)Acknowledgments
I am indebted to Prof. K. Osada of Nagoya University for support and technical advice related to experimentation and composition of this report. I also extend my gratitude for technical support from the High Voltage Electron Microscope Laboratory of Nagoya University.
Additional information
Funding
References
- Adachi, K., S. H. Chung, and P. R. Buseck. 2010. Shapes of soot aerosol particles and implications for their effects on climate. J. Geophys. Res. 115 (D15): D15206. doi:https://doi.org/10.1029/2009JD012868.
- Adachi, K., E. J. Freney, and P. R. Buseck. 2011. Shapes of internally mixed hygroscopic aerosol particles after deliquescence, and their effect on light scattering. Geophys. Res. Lett. 38 (13). doi:https://doi.org/10.1029/2011GL047540.
- Altaf, M. B., A. Zuend, and M. A. Freedman. 2016. Role of nucleation mechanism on the size dependent morphology of organic aerosol. Chem. Commun. (Camb.) 52 (59):9220–3. doi:https://doi.org/10.1039/c6cc03826c.
- Bai, Z., Y. Ji, Y. Pi, K. Yang, L. Wang, Y. Zhang, Y. Zhai, Z. Yan, and X. Han. 2018. Hygroscopic analysis of individual Beijing haze aerosol particles by environmental scanning electron microscopy. Atmos. Environ. 172:149–56. doi:https://doi.org/10.1016/j.atmosenv.2017.10.031.
- Bigg, E. K. 1980. Comparison of aerosol at four baseline atmospheric monitoring stations. J. Appl. Meteor. 19 (5):521–33. > 2.0.CO;2. doi:https://doi.org/10.1175/1520-0450(1980)019 < 0521:COAAFB.
- Cao, F., S.-C. Zhang, K. Kawamura, X. Liu, C. Yang, Z. Xu, M. Fan, W. Zhang, M. Bao, Y. Chang, et al. 2017. Chemical characteristics of dicarboxylic acids and related organic compounds in PM2.5 during biomass-burning and non-biomass-burning seasons at a rural site of Northeast China. Environ. Pollut. 231 (Pt 1):654–63. doi:https://doi.org/10.1016/j.envpol.2017.08.045.
- Coz, E., B. Artíñano, A. L. Robinson, G. S. Casuccio, T. L. Lersch, and S. N. Pandis. 2008. Individual particle morphology and acidity. Aerosol Sci. Technol. 42 (3):224–32. doi:https://doi.org/10.1080/02786820801958759.
- Ebert, M., M. Inerle-Hof, and S. Weinbruch. 2002. Environmental scanning electron microscopy as a new technique to determine the hygroscopic behaviour of individual aerosol particles. Atmos. Environ. 36 (39–40):5909–16. doi:https://doi.org/10.1016/S1352-2310(02)00774-4.
- Ferek, R. J., A. L. Lazrus, and J. W. Winchester. 1983. Electron microscopy of acidic aerosols collected over the northeastern United States. Atmos. Environ. 17 (8):1545–61. doi:https://doi.org/10.1016/0004-6981(83)90308-6.
- Frank, R., and J. P. Lodge. 1967. Morphological identification of airborne particles with the electron microscope. J. Microsc. 6:449–56.
- Freney, E. J., S. T. Martin, and P. R. Buseck. 2009. Deliquescence and efflorescence of potassium salts relevant to biomass-burning aerosol. Aerosol Sci. Technol. 43:799–807. doi: https://doi.org/10.1080/02786820902946620
- Fu, H., M. Zhang, W. Li, J. Chen, L. Wang, X. Quan, and W. Wang. 2012. Morphology, composition and mixing state of individual carbonaceous aerosol in urban Shanghai. Atmos. Chem. Phys. 12 (2):693–707. doi:https://doi.org/10.5194/acp-12-693-2012.
- Gras, J. L., and G. P. Avers. 1979. On sizing impacted sulfuric acid aerosol particles. J. Appl. Meteor. 18 (5):634–8. > 2.0.CO;2. doi:https://doi.org/10.1175/1520-0450(1979)018 < 0634:OSISAA.
- Hiranuma, N., S. D. Brooks, B. W. Auvermann, and R. Littleton. 2008. Using environmental scanning electron microscopy to determine the hygroscopic properties of agricultural aerosols. Atmos. Environ. 42 (9):1983–94. doi:https://doi.org/10.1016/j.atmosenv.2007.12.003.
- Kawamura, K., M. M. M. Hoque, T. S. Bates, and P. K. Quinn. 2017. Molecular distributions and isotopic compositions of organic aerosols over the western North Atlantic: Dicarboxylic acids, related compounds, sugars, and secondary organic aerosol tracers. Org. Geochem. 113:229–38. doi:https://doi.org/10.1016/j.orggeochem.2017.08.007.
- Kobayashi, H., M. Hayashi, K. Shiraishi, Y. Nakura, T. Enomoto, K. Miura, H. Takahashi, Y. Igarashi, H. Naoe, N. Kaneyasu, et al. 2014. Development of a polarization optical particle counter capable of aerosol type classification. Atmos. Environ. 97:486–92. doi:https://doi.org/10.1016/j.atmosenv.2014.05.006.
- Kokhanovsky, A. A. 2012. Light scattering reviews. Berlin, Heidelberg: Springer.
- Li, J., M. Pósfai, P. V. Hobbs, and P. R. Buseck. 2003. Individual aerosol particles from biomass burning in southern Africa: 2, Compositions and aging of inorganic particles. J. Geophys. Res. 108 (D13):8484. doi:https://doi.org/10.1029/2002.JD002310.
- Li, W., L. Shao, D. Zhang, C.-U. Ro, M. Hu, X. Bi, H. Geng, A. Matsuki, H. Niu, and J. Chen. 2016a. Review a review of single aerosol particle studies in the atmosphere of East Asia: Morphology, mixing state, source, and heterogeneous reactions. J. Clean. Prod. 112:1330–49. 0959-6526. doi:https://doi.org/10.1016/j.jclepro.2015.04.050.
- Li, W., J. Sun, L. Xu, Z. Shi, N. Riemer, Y. Sun, P. Fu, J. Zhang, Y. Lin, X. Wang, et al. 2016b. A conceptual framework for mixing structures in individual aerosol particles. J. Geophys. Res. Atmos. 121 (22):13784–98. doi:https://doi.org/10.1002/2016JD025252.
- Li, W. J., L. Y. Shao, and P. R. Buseck. 2010. Haze types in Beijing and the influence of agricultural biomass burning. Atmos. Chem. Phys. 10 (17):8119–30. doi:https://doi.org/10.5194/acp-10-8119-2010.
- Liu, I., and M. I. Mishchenko. 2020. Spectrally dependent linear depolarization and lidar ratios for nonspherical smoke aerosols. J. Quant. Spectrosc. Radiat. Transfer 248:106953. doi:https://doi.org/10.1016/j.jqsrt.2020.106953.
- Ma, X., F. Yu, and G. Luo. 2012. Aerosol direct radiative forcing based on GEOS-Chem-APM and uncertainties. Atmos. Chem. Phys. 12 (12):5563–81. doi:https://doi.org/10.5194/acp-12-5563-2012.
- Matsui, H. 2016a. Black carbon simulations using a size- and mixing-state-resolved three-dimensional model: 1. Radiative effects and their uncertainties. J. Geophys. Res. Atmos. 121 (4):1793–807. doi:https://doi.org/10.1002/2015/JD023998.
- Matsui, H. 2016b. Black carbon simulations using a size- and mixing-state-resolved three-dimensional model: 2. Aging timescale and its impact over East Asia. J. Geophys. Res. Atmos. 121 (4):1808–21. doi:https://doi.org/10.1002/2015/JD023999.
- Matsumura, T., and M. Hayashi. 2007. Hygroscopic growth of an (NH4)2SO4 aqueous solution droplet measured using an environmental scanning electron microscope (ESEM). Aerosol Sci. Technol. 41 (8):770–4. doi:https://doi.org/10.1080/02786820701436831.
- Mayama, N., Y. Miura, K. Misawa, A. Takami, T. Sakamoto, and M. Fuji. 2013. Characterization of black carbon in fine aerosol particles using high lateral resolution TOF-SIMS. Anal. Sci. 29 (4):479–81. doi:https://doi.org/10.2116/analsci.29.479.
- Moosmüller, H., R. K. Chakrabarty, and W. P. Arnott. 2009. Aerosol light absorption and its measurement: A review. J. Quant. Spectrosc. Radiat. Transfer 110 (11):844–78. doi:https://doi.org/10.1016/j.jqsrt.2009.02.035.
- Nakagawa, M., T. Nakayama, H. Sasago, S. Ueda, D. S. Venables, and Y. Matsumi. 2016. Design and characterization of a novel single-particle polar nephelometer. Aerosol Sci. Technol. 50 (4):392–04. doi:https://doi.org/10.1080/02786826.2016.1155105.
- Pósfai, M., J. R. Anderson, P. R. Buseck, and H. Sievering. 1995. Compositional variations of sea-salt-mode aerosol particles from the North Atlantic. J. Geophys. Res. 100 (D11):23063–74. doi:https://doi.org/10.1029/95JD01636.
- Prenni, A., P. J. DeMott, and S. M. Kreidenweis. 2003. Water uptake of internally mixed particles containing ammonium sulfate and dicarboxylic acids. Atmos. Environ. 37 (30):4243–51. doi:https://doi.org/10.1016/S1352-2310(03)00559-4.
- Riemer, N., A. P. Ault, M. West, R. L. Craig, and J. H. Curtis. 2019. Aerosol mixing state: Measurements, modelling, and Impact. Rev. Geophys. 57 (2):187–249. doi:https://doi.org/10.1029/2018RG000615.
- Riemer, N., and M. West. 2013. Quantifying aerosol mixing state with entropy and diversity measures. Atmos. Chem. Phys. 13 (22):11423–39. doi:https://doi.org/10.5194/acp-13-11423-2013.
- Scarnato, B. V., S. D. T. Vahidinia, T. W. Richard, and T. W. Kirchstetter. 2013. Effects of internal mixing and aggregate morphology on optical properties of black carbon using a discrete dipole approximation model. Atmos. Chem. Phys. 13 (10):5089–101. doi:https://doi.org/10.5194/acp-13-5089-2013.
- Seinfeld, J. H. and S. N. Pandis, eds. 2006. Atmospheric chemistry and physics: From air pollution to climate change. New York, NY: Wiley.
- Shi, Z., D. Zhang, H. Ji, S. Hasegawa, and M. Hayashi. 2008. Modification of soot by volatile species in an urban atmosphere. Sci Total Environ. 389 (1):195–291. doi:https://doi.org/10.1016/j.scitotenv.2007.08.016.
- Song, M., C. Marcolli, U. K. Krieger, A. Zuend, and T. Peter. 2012. Liquid–liquid phase separation and morphology of internally mixed dicarboxylic acids/ammonium sulfate/water particles. Atmos. Chem. Phys. 12 (5):2691–712. doi:https://doi.org/10.5194/acp-12-2691-2012.
- Tang, I. N., and H. R. Munkelwitz. 1993. Composition and temperature dependence of the deliquescence properties of hygroscopic aerosols. Atmos. Environ. 27 (4):467–73. doi:https://doi.org/10.1016/0960-1686(93)90204-C.
- Tang, I. N., and H. R. Munkelwitz. 1994. Water activities, densities, and refractive indices of aqueous sulfates and sodium nitrate droplets of atmospheric importance. J. Geophys. Res. 99 (D9):18801–8. doi:https://doi.org/10.1029/94JD01345.
- Treuel, L., S. Pederzani, and R. Zellner. 2009. Deliquescence behaviour and crystallisation of ternary ammonium sulfate/dicarboxylic acid/water aerosols. Phys. Chem. Chem. Phys. 11 (36):7976–84. doi:https://doi.org/10.1039/b905007h.
- Ueda, S., T. Nakayama, F. Taketani, K. Adachi, A. Matsuki, Y. Iwamoto, Y. Sadanaga, and Y. Matsumi. 2016. Light absorption and morphological properties of soot-containing aerosols observed at an East Asian outflow site, Noto Peninsula, Japan. Atmos. Chem. Phys. 16 (4):2525–41. doi:https://doi.org/10.5194/acp-16-2525-2016.
- Ueda, S., K. Osada, K. Hara, M. Yabuki, F. Hashihama, and J. Kanda. 2018. Morphological features and mixing states of soot-containing particles in the marine boundary layer over the Indian and Southern Oceans. Atmos. Chem. Phys. 18 (13):9207–24. doi:https://doi.org/10.5194/acp-18-9207-2018.
- Ueda, S., K. Osada, and A. Takami. 2011. Morphological features of soot-containing particles internally mixed with water-soluble materials in continental outflow observed at Cape Hedo, Okinawa, Japan. J. Geophys. Res. 116 (D17):D17207. doi:https://doi.org/10.1029/2010JD015565.
- Veghte, D. P., D. R. Bittner, and M. R. Freedman. 2014. Cryo-transmission electron microscopy imaging of the morphology of submicrometer aerosol containing organic acids and ammonium sulfate. Anal. Chem. 86 (5):2436–42. doi:https://doi.org/10.1021/ac403279f.
- Waller, R. E., A. G. F. Brooks, and J. Cartwright. 1963. An electron microscope study of particles in town air. J. Air Wet. Pollut. 7:779–85.
- Wang, W., L. Shao, J. Xing, J. Li, L. Chang, and W. Li. 2018. Physicochemical characteristics of individual aerosol particles during the 2015 China victory day parade in Beijing. Atmosphere 9 (2):40. doi:https://doi.org/10.3390/atmos90200402017.
- Xu, J., D. Imre, R. McGraw, and I. Tang. 1998. Ammonium sulfate: Equilibrium and metastability phase diagrams from 40 to -50 °C. J. Phys. Chem. B 102 (38):7462–9. doi:https://doi.org/10.1021/jp981929x.
- Xu, L., L. Liu, J. Zhang, Y. Zhang, Y. Ren, X. Wang, and W. Li. 2017. Morphology, composition, and mixing state of individual aerosol particles in northeast China during wintertime. Atmosphere 8 (12):47–9. doi:https://doi.org/10.3390/atmos8030047.
- Yu, J. Z., X.-F. Huang, J. Xu, and M. Hu. 2005. When aerosol sulfate goes up, so does oxalate: Implication for the formation mechanisms of oxalate. Environ. Sci. Technol. 39 (1):128–33. doi:https://doi.org/10.1021/es049559f.
- Zelenay, V., M. Ammann, A. Křepelová, M. Birrer, G. Tzvetkov, M. G. C. Vernooij, J. Raabe, and T. Huthwelker. 2011. Direct observation of water uptake and release in individual submicrometer sized ammonium sulfate and ammonium sulfate/adipic acid particles using X-ray microspectroscopy. J. Aerosol Sci. 42 (1):38–51. doi:https://doi.org/10.1016/j.jaerosci.2010.11.001.