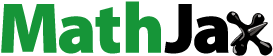
Abstract
The COVID-19 pandemic has resulted in a severe shortage of personal protective equipment. The World Health Organization (WHO) and the US Centers for Disease Control and Prevention (CDC) recommend the general public to use cloth masks as a protection for individuals and reduce the transmission of the disease. Here, we evaluated the size-dependent filtration efficiency of submicron particles and the overall filtration efficiency (total rejection of particles) of multiple commercially available materials in the size range of 17–700 nm. Single-layer samples of woven fabrics such as cotton and polyester have overall filtration efficiency of 12%−22%. Blended fabrics filter 11%−48% of particles. Products manufactured from cellulose have filtration efficiency of 29%−33%. Materials commonly found and used in hospitals remove 20%−63% of particles and filtration efficiency of filter materials ranges from 40% to 96%. Particle filtration of materials becomes more efficient when individual materials are stacked and used as multiple layers. Different combinations of woven, nonwoven, and blended fabrics were evaluated and have overall particle filtration efficiency of 38%−63%. Results in this study highlight the importance of using multiple layers in homemade masks to enhance filtration efficiency. Commercially accessible materials like Halyard sterilization wrap and blackout drapery lining show the best filtration performance among all tested single-layer materials and therefore are recommended to use as one of the layers in homemade masks. Universal mask wearing – regardless of whether an individual is infected – will help reduce transmission risk.
Copyright © 2021 American Association for Aerosol Research
EDITOR:
1. Introduction
Severe acute respiratory syndrome coronavirus 2 (SARS-CoV-2), which was first reported in 2019, has become a severe global health crisis (Lai et al. Citation2020; National Academies of Sciences, Engineering and Medicine Citation2020). The World Health Organization (WHO) noted that droplet and fomite transmissions are the primary modes of transmission of SARS-CoV-2 (WHO Citation2020b). Over the course of the pandemic, there has been increased recognition of the role of airborne transmission, where viruses in exhaled aerosols by infected individuals can stay suspended in the air for extended periods and be transported far from the sources (CDC Citation2020a; Lednicky et al. Citation2020; Morawska and Cao Citation2020; Morawska and Milton Citation2020; Prather, Wang, and Schooley Citation2020). Humans emit respiratory particles in the range of 0.1–1000 µm as one breathes, talks, sings, sneezes, coughs, etc. (Johnson et al. Citation2011). Due to its rapid transmission, the SARS-CoV-2 outbreak has increased the demand of high-filtration performance respirators (N95 respirators) for protection against the disease (Bałazy, Toivola, Adhikari et al. Citation2006; Bałazy, Toivola, Reponen et al. Citation2006). The skyrocketing demand of respirators caused a significant shortage of personal protective equipment (PPE) even in hospitals, which implies that the access of the general public to such high-performance respirators is minimal. In response to such a shortage of PPE, use of cloth masks by the public has been recommended to reduce the spread of the virus (CDC Citation2020b; WHO Citation2020a).
Efforts to evaluate the performance of homemade face masks are not only limited to the current pandemic but are also considerable in previous outbreaks such as SARS in Asia, Middle East respiratory syndrome (MERS), and avian influenza-A epidemics. A wide range in performance of homemade face masks has been reported. Bowen (Citation2010) tested bandana and reported overall filtration efficiency of only 11.3% for particles in the ∼0.1 − 20 µm size range. Rengasamy, Eimer, and Shaffer (Citation2010) tested 12 common fabric materials and 3 cloth masks against 0.02 − 1 µm particles and observed 10%−60% overall filtration efficiency for the tested materials. Shakya et al. (Citation2017) examined three types of cloth masks for particles in the 0.03 − 2.5 µm size range and observed 80%−90% overall filtration efficiency for one mask but 15%−57% for the others. Furthermore, Davies et al. (Citation2013) evaluated filtration of microorganisms by nine different materials and reported that homemade masks were three times less effective than the surgical mask. When taking mask fit (on the face) into consideration, it has been shown that homemade masks reduce exposure to respiratory infections among the general population, though they are less effective than surgical masks and respirators (Van Der Sande, Teunis, and Sabel Citation2008). Taken together, all these studies indicated that homemade masks provide a certain degree of filtration and protection, though their performance can vary and can be less efficient than that of surgical masks.
Although most of these studies conducted in the era of previous pandemics are limited to cloth fabrics as the materials for producing homemade face masks, a few recent studies evaluated materials in addition to cloth fabrics. Hao et al. (Citation2020) tested household air filters, vacuum bags, and coffee filters in addition to cloth materials. They observed that the performance of multiple layers of household air filter was comparable to N95 respirators or KN95 masks in terms of both filtration efficiency and flow resistance for particles in the 10 − 600 nm size range. Drewnick et al. (Citation2021) also reported that the performance of vacuum bag for particles in the 0.02 − 10 µm size range was comparable to N95 respirators among the 44 household materials tested in their study. It is important to note that borosilicate fiberglass-based materials pose certain safety risks when used as face masks due to the potential for inhalation of glass fiber shards. Furthermore, caution shall be exercised in using vacuum bags as materials for face masks due to potential impregnation of these materials with biocides.
In this study, we measured filtration efficiency of submicron particles for various materials that are commercially accessible and are not limited to cloth fabrics. Measurements were performed on both single-layer materials and multiple-layered samples. As filtration efficiency is highly dependent on particle size, we report both size-dependent and overall filtration efficiency (total rejection of particles). Based on these results, recommendations are provided to guide the use of different materials in homemade face masks for reducing the spread of COVID-19.
2. Experiment
We tested 33 different samples in this study, summarized in . They include single-layer woven fabrics such as cotton and woven polyester, blended fabrics, nonwoven materials, cellulose-based materials, materials commonly found and used in hospitals, and various filter materials. Different combinations of woven, nonwoven, and blended fabrics were also evaluated. illustrates the schematic of the testing setup. We followed similar test procedures outlined in the NIOSH 42 CFR 84 test protocol for particulate respirator certification, in which the test sodium chloride (NaCl) aerosols have a particle size distribution with count median diameter of 75 nm and a geometric standard deviation not exceeding 1.9 (Rengasamy, Eimer, and Shaffer Citation2010). Experiments were performed at 1.9 LPM (equivalent face velocity of 8.6 cm sec−1). Polydisperse aerosols were first generated by atomization of 0.05 M NaCl solution with a constant output atomizer (TSI 3076) at 20 psi using UHP zero air. The generated aerosols were dried by a diffusion dryer and passed through a 210Po neutralizer (NRD Inc., Grand Island, NY, USA) where excess charges were eliminated to avoid overestimation in filtration efficiency due to electrostatic attraction. An aerosol dilution system was added in the setup to lower the NaCl aerosol number concentration to prevent clogs in the system that could cause an unstable flow. A circular sheet (25-mm diameter) was punched from each sample and placed into a stainless steel filter holder (Pall Corporation, Port Washington, NY, USA). The aerosols were either passed through the stainless steel filter holder (with the sample in place) or through a bypass line before their size-dependent number concentrations were measured by a Scanning Mobility Particle Sizer (SMPS) that consists of a Differential Mobility Analyzer (DMA, TSI 3080) and a Condensation Particle Counter (CPC, TSI 3775). SMPS was set to scan particle size ranging from 17 to 1000 nm (electrical mobility diameter). The pressure drop across each sample was measured using a Magnehelic Gage (Dwyer, Series 2000) (). The pressure difference due to the filter holder was corrected for at the same flow rate with no sample installed in the filter holder. To ensure the consistency of the experimental setup and measurements, the particle filtration efficiency of a N95 respirator (3M 8210) was validated before testing sample materials each day. We note that there are a few differences between our test procedures and the NIOSH protocols (42 CFR 84). Specifically, the tested samples were not preconditioned at 38 °C and 85% relative humidity, although we found that particle removal efficiency of conditioned and unconditioned N95 respirator samples (3M 8210) only showed a minor difference (Supplementary Figure S1). Also, the aerosol mass concentration was substantially lower in the setup here, and the NaCl particle size distribution was skewed toward a smaller size compared to NIOSH protocols (count median diameter in our study ranged from 55 to 65 nm with a geometric standard deviation of ∼1.9).
Table 1. Particle filtration efficiency and pressure drop of samples.
During testing, a total of nine SMPS scans were performed for each sample following this sequence: three bypass scans, three sample scans, and three bypass scans. Data from the first sample scan was discarded due to the interference of residual particles from the bypass scans. As the particle filtration efficiency is a strong function of particle diameter (Dp) (Hinds Citation1999), it was evaluated for each size bin. The size-dependent particle filtration efficiency (η (Dp)) was calculated as follows:
(1)
(1)
where nbypass(Dp) and nsample(Dp) represent the number concentration of particles with a diameter Dp when aerosols flow through bypass (average of six bypass scans) and sample lines (average of two sample scans), respectively. We also calculated the overall filtration efficiency (ηoverall), which is the total rejection of particles by the sample, using the equation below:
(2)
(2)
where nbypass and nsample are the total particle number concentrations in the bypass and sample lines, respectively. In addition to overall filtration efficiency, we report particle filtration efficiency at 300 nm in as it is a conventional indicator of filter performance (Hao et al. Citation2020).
3. Results and discussion
3.1. Single-layer samples
The size-dependent particle filtration efficiency of each single-layer material is shown in . Although the SMPS scans up to 1000 nm, we show data in the size range of 17–700 nm only, due to the low concentrations of particles and their large variability in larger size bins (>700 nm). Such variability was also observed during the tests of N95 respirator samples, which were used as the reference material to monitor the consistency of experiments each day (Supplementary Figure S1). Filtration is governed by five mechanisms: interception, inertial impaction, diffusion, gravitational settling, and electrostatic attraction (Hinds Citation1999). The filtration of particles in the size range in this study would mostly be affected by diffusion, interception, impaction, and electrostatic attraction. Specifically, governing mechanism shifts from diffusion to interception and impaction at around the particle diameter of minimum filtration efficiency. In addition to the N95 respirator, some samples tested (e.g., surgical mask, Minimum Efficiency Reporting Value (MERV) 13 filter, High Performance Particulate Air (HEPA) filter, and other synthetic materials) may also contain electret media, and for these samples, electrostatic attraction will be important in addition to the mechanisms of mechanical filtration. The results in show that all materials have different particle filtration efficiency as a function of particle diameter. Woven fabrics such as cotton and woven polyester () show particle filtration efficiency lower than 45% in the entire size range, with a minimum of 4% at Dp = 188 nm for cotton. The 100% cotton sample tested in this study is inferior to the sample of woven polyester fabric, as it only has overall filtration efficiency of 12% (). The overall filtration efficiency of cotton is similar to values reported in previous studies that investigated filtration of submicron particles. Rengasamy, Eimer, and Shaffer (Citation2010) evaluated five different categories of fabric (sweatshirts, t-shirts, towels, scarves, and cloth masks) at face velocities of 5.5 and 16.5 cm sec−1 and reported overall particle penetration to be higher than 80% for 100% cotton samples (i.e., filtration efficiency < 20%) except for the towels category. Zhao et al. (Citation2020) tested three different types of cotton; woven structure showed overall filtration efficiency of 5% while knit structure showed around 24%. Drewnick et al. (Citation2021) tested samples at different face velocities (high: 12.9 cm sec−1/low: 5.3 cm sec−1) and observed filtration efficiency of single-layer cotton to be <20% for the same particle size range of this study.
Figure 2. Filtration efficiency of single-layer samples. (a) Woven fabrics, (b) blended materials, (c) nonwoven materials, (d) cellulose processed samples, (e) materials used in hospitals, (f) filter samples, N95 respirator, and blue surgical mask. We condensed SMPS size distribution into seven size bins (23, 39, 64, 102, 166, 294, and 533 nm) for easier readability. Uncertainties correspond to the propagation of 1σ of particle number concentrations in EquationEquation (1)(1)
(1) .
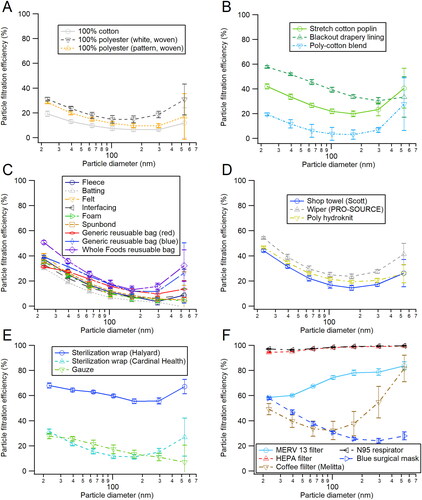
Konda et al. (Citation2020b) reported that the filtration efficiency of woven material is dependent on the thread counts of woven fabric. They observed around 65%−85% filtration efficiency for 600 threads per inch (TPI) cotton but only 7%−36% for 80 TPI cotton. However, other studies reported that particle filtration performance did not always increase with increasing thread counts. Hao et al. (Citation2020) did not observe thread count dependency for single-layer cotton at a face velocity of 9.2 cm sec−1. They instead observed a higher filtration efficiency for 600 TPI than 1000 TPI. Drewnick et al. (Citation2021) also found a lack of correlation between particle filtration efficiency and thread count. The authors suggested that the thinner threads in samples with a larger thread count might have a negative impact on filtration efficiency. Electric charging of filtering material can be another factor that can influence the particle filtration performance of materials. Zhao et al. (Citation2020) found that filtration efficiency of cotton decreases, but polyester increases when the filter medium is charged. Drewnick et al. (Citation2021) observed an increased electrostatic deposition for synthetic samples but negligible or small contribution of electrostatic deposition to filtration efficiency were found for natural fiber samples.
Poly cotton blend fabric (65% cotton, 35% polyester) shows comparable filtration efficiency to the 100% cotton sample for particles smaller than 200 nm but exhibits higher efficiency for particles larger than 200 nm (). The other two blended fabrics tested in this study, which are stretch cotton poplin (97% cotton, 3% spandex) and blackout drapery lining (30% cotton, 70% polyester), show 2.8 and 4.4 times higher overall filtration efficiency compared to the poly cotton blend, respectively (). For woven and blended materials, we observe that the higher filtration performance corresponds to higher material area density (Supplementary Figure S2). Blackout drapery lining is the densest material (233 g m−2), followed by stretch cotton poplin (175 g m−2), polyester (155 g m−2), and cotton (128 g m−2). The scanning electron microscope (SEM) images in Supplementary Figure S3 show that the blackout drapery lining has a more entangled structure and an apparently higher tortuosity, which likely contributes to enhanced filtration efficiency. Unlike the material area density, the thickness of these samples did not show a direct relationship with their filtration efficiency. Specifically, although the blackout drapery lining (0.36 mm) is the thickest material tested among these categories of samples, the thickness of 100% white polyester (0.23 mm) and stretch cotton poplin (0.20 mm) are comparable. We also tested the particle filtration efficiency of three reusable shopping bags (two generic bags, one Whole Foods bag). Among all the nonwoven fabrics tested, the Whole Foods reusable bag (100% polyester from 80% post-consumer recycled plastic bottles) has the best performance, with overall filtration efficiency of 33%. Compared to woven fabrics, nonwoven materials generally show comparable particle filtration for smaller particles (). However, filtration efficiency of larger particles varies depending on the material. Batting, felt, foam, and spunbond display weak U-shaped filtration curves within the particle size range tested in this study. Specifically, filtration ability of these materials is poor for the particles larger than 200 nm. On the other hand, the three reusable bags have comparable filtration efficiency to the woven polyester samples for these larger particles.
Figure 3. Particle filtration efficiency of multilayered samples. (a) two-layered samples (A: cotton + stretch cotton poplin, B: cotton + blackout drapery lining) and (b) three-layered samples (A: cotton + Whole Foods reusable bag + cotton, B: polyester + Whole Foods reusable bag + cotton, C: polyester + Whole Foods reusable bag + polyester, D: poly cotton blend + Whole Foods reusable bag + polyester, E: poly cotton blend + Whole Foods reusable bag + cotton). Uncertainties correspond to the propagation of 1σ of particle number concentrations in EquationEquation (1)(1)
(1) .
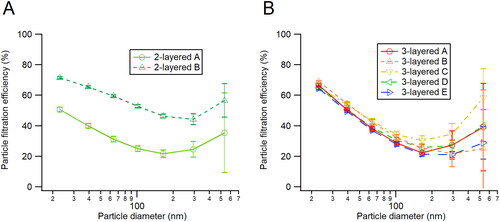
Cellulose-processed materials are commonly used in wiper/towel products. The filtration efficiency curves for shop towel, wiper, and poly hydroknit samples are shown in . All materials in this category have better particle filtration efficiency than woven and nonwoven materials tested in this study except for the Whole Foods reusable bag. The filtration performance of wiper/towel products can differ depending on the brand, manufacturing process, and the type of materials used. Among the three tested samples, shop towel from Scotts, which is mainly composed of cellulose, shows the least efficiency, filtering 29% of particles between 17 and 700 nm. General purpose wiper from PRO-SOURCE is made of bonded cellulose using DRC (double-reinforced crepe) technology. It has the highest filtration efficiency in this category and filters 37% of particles overall. Poly hydroknit is a blend of polyester fiber for softness and oil absorbency, wood fiber for water absorbency, and spunbond material for durability. The filtration performance of this material generally falls between Scotts shop towel and PRO-SOURCE general purpose wiper and removes 32% of particles overall. Unlike the towel and wiper samples whose filtration efficiency shows a continuous increase for particles >200 nm, the poly hydroknit sample shows a weak enhancement in filtration performance for particles larger than ∼400 nm. Nemer et al. (Citation2020) reported aerosol filtration efficiency of 19 different wiper/towel products including both single- and multiple-layer samples, which ranged from 11% to 44%. It was found that single-layer Microfiber Magic Streak Free Cloth, Kimtech Wiper, and Contec Clean Room Wipe showed filtration efficiency of 36%, 44%, and 23%, respectively, for particles ranging from ∼15 nm to 450 nm. Although these wiper/towel products were manufactured with different materials such as polypropylene, their filtration efficiency was similar to our findings.
exhibits the filtration performance of materials that are commonly found and used in hospitals. Sterilization wraps are commonly used in the packaging of surgical instruments and are also commercially available. The particle filtration efficiency of sterilization wraps depends on the brand. Halyard sterilization wrap (weight model: H500; made from spunbond-meltblown-spunbond polypropylene) shows the best performance among all the tested materials in this study other than the filter materials typically used in air circulation systems (see next section). The multilayered structure of Halyard sterilization wrap is shown in the SEM image in Supplementary Figure S4. Not only does it have a good overall filtration of 63%, it also consistently has a good filtration efficiency for particles across all sizes. The filtration efficiency of the Halyard sample we tested is in between the values reported previously for lighter weight (H300; 49%) and heavier weight (H600; 65%) models for 300 nm particles (Lammers et al. Citation2021; Oude Vrielink and Meijer Citation2020; Pei et al. Citation2020). Cardinal Health (CH4045) paper sterilization wrap (made from wetlaid cellulose) and gauze perform relatively poorly compared to Halyard sterilization wrap, having overall filtration efficiency of 20% and 23%, respectively. The filtration efficiency of Cardinal Health sterilization wrap is lower than what was observed in Pei et al. (Citation2020), which tested Cardinal Health CH600 sterilization wrap (made from spunbond-meltblown-spunbond polypropylene) at the face velocity of 10.5 cm sec−1 and observed particle filtration efficiency of 34% at 300 nm. Taken together, these results suggest that multilayered structures and heavier weight models of sterilization wraps have better filtration efficiency. Nevertheless, the possible effects of differing face velocity on filtration efficiency should be considered when comparing results across studies in the literature. Similar to batting, felt, foam, and spunbond, the filtration curve of gauze does not exhibit a strong U-shape and the filtration efficiency is especially poor for particles between 300 and 700 nm compared to other samples.
Figure 4. Size distributions and total number concentrations of human exhaled particles from speaking (a and c) and coughing (b and d) for three scenarios: no mask (data from Johnson et al. [Citation2011]), and after filtration by one and two cloth masks.
![Figure 4. Size distributions and total number concentrations of human exhaled particles from speaking (a and c) and coughing (b and d) for three scenarios: no mask (data from Johnson et al. [Citation2011]), and after filtration by one and two cloth masks.](/cms/asset/2f580a16-c69c-494e-89f5-17c7d4b551a1/uast_a_1905149_f0004_c.jpg)
Particle filtration efficiency of different types of filters is shown in . Among the tested filters, HEPA filters report the highest particle filtration efficiency followed by MERV 13 filter and coffee filter. Typically, HEPA and MERV 13 filters are made of fibrous materials. These filters are commonly used in heating, ventilating, and air-conditioning (HVAC) systems. MERV 13 filter is designed to filter 90% of particles in the 3 − 10 µm range, 85% of particles in the 1 − 3 µm range, and 50% of particles in the 0.3 − 1 µm range (ASHRAE Citation2017). Although the shape of filtration efficiency curve of MERV 13 filter in is different from some previous studies (Azimi, Zhao, and Stephens Citation2014; Stephens and Siegel Citation2013), the shape of the curve is dependent on the manufacturers of the filters (Fazli, Zeng, and Stephens Citation2019). The filtration efficiency of 0.3 − 1 µm particles for the MERV 13 filter tested in our study is 81%, in accordance with the ASHARE rating. HEPA filter, which can be classified as MERV 17 to 20 rating (Hecker and Hofacre Citation2008), shows overall filtration efficiency of 96%, comparable to N95 respirators ( and Supplementary Figure S1). A recent study that tested fibrous materials also reported that the particle filtration efficiency of vacuum bag is comparable to KN95 or N95 respirators (Hao et al. Citation2020). The coffee filter (Melitta) tested in this study shows decent particle filtration efficiency of 40%, for overall 58% at 300 nm, and a minimum of 31% at 88 nm. Such filtration efficiency is comparable to blue surgical mask, but the size-dependent filtration shows that the coffee filter shows better filtration performance for particles larger than 100 nm but the opposite for the smaller particles (). Coffee filters has other brands (e.g., Natural Brew, Brew Rite, etc.) were tested by Hao et al. (Citation2020). Although filtration efficiency of multiple layers (three layers) of coffee filters was evaluated by Hao et al. (Citation2020), they reported a lower filtration performance, smaller than 30% at 300 nm at a face velocity similar to this study. Therefore, it appears that the filtration performance of coffee filters can be highly dependent on the brand/manufacturer.
Overall, while the samples tested in this category show better filtration performance than other materials, we do not recommend using them as face mask materials in general. MERV 13 and HEPA filters are typically made of fiberglass, which is hazardous to breathe through. For this reason, unless they are certified to be free of fiberglass, these materials should not be used as inner layers for homemade face masks even though they show outstanding performance. In the case of coffee filter, we observe the highest pressure drop across this sample compared to all other single-layer samples tested in this study. This pressure drop (220 Pa) is twice higher than that of N95 respirators (110 Pa) in our study () but is lower than the three-layered coffee filter samples in the study by Hao et al. (Citation2020) (∼450 Pa). This indicates that there could potentially be breathability issues if the material is used as one of the layers in homemade face masks, and caution shall be exercised.
3.2. Multilayered samples
We observed an improvement in particle filtration efficiency with multiple layers. shows the filtration efficiency of two-layered samples, which consist of a 100% cotton layer and either stretch cotton poplin or blackout drapery lining as a second layer. Excluding the filter materials shown in , the two-layered cotton/drapery lining sample shows the most efficient particle filtration in this study, removing 63% of particles overall (this filtration efficiency is the same as the Halyard sterilization wrap). Compared to the single-layer 100% cotton sample, particle removal efficiency improves by 26% and 51% with the addition of the stretch cotton poplin or blackout drapery lining, respectively.
The three-layered samples in are combinations of 100% cotton, poly cotton blend, and polyesters (100% polyester – white or pattern sample; recycled polyester – Whole Foods reusable bag). It is noted that recycled polyester is used as the middle layer for all three-layered samples tested. Filtration efficiency of all samples reports a subtle difference, from 46% to 51%. The combination C, which consists of polyester fabrics for all three layers, shows higher filtration efficiency for particles larger than 100 nm but comparable to the other samples for particles below 100 nm. Among all the individual materials used in the three-layered samples, the filtration performance of recycled polyester is the most efficient as discussed earlier. This indicates that this layer could play a key role in dampening particle penetration in the three-layered samples. The overall filtration efficiency of each three-layered sample improves by 13%−39% compared to the individual layers. The improvement in overall filtration efficiency (ηtotal) of multilayered samples can be approximated from the overall filtration efficiency of individual layers as in previous studies (Supplementary Figure S8a) (Drewnick et al. Citation2021; Shou et al. Citation2015):
(3)
(3)
The pressure drop of two- and three-layered samples ranged from 260 to 400 Pa (). Among these samples, the two-layered A (cotton + stretch cotton poplin) shows the largest pressure drop, likely due to the large pressure drop across the stretch cotton poplin itself (200 Pa). Considering both filtration efficiency and pressure drop, we recommend two-layered B (cotton + blackout drapery lining) as it has the highest filtration efficiency among these multilayered samples and a relatively low pressure drop (270 Pa). Although this pressure drop is larger than that measured for N95 respirator and surgical mask in this study, it is comparable to commercial respirators reported in a previous study (Hao et al. Citation2020). The pressure drop of multilayered samples in this study shows a linearly additive relationship with the pressure drop of individual layers (Supplementary Figure S8b). It is noted that multilayered samples that include a cotton layer (two-layered A, two-layered B, three-layered A, three-layered B, and three-layered E) exhibited an intercept (115 Pa), which could be due to interference between the layers, i.e., layers having poor alignments (Drewnick et al. Citation2021).
Recent studies have reported improvement in particle filtration efficiency when two or more layers of the same material are combined (Drewnick et al. Citation2021; Hao et al. Citation2020; Konda et al. Citation2020b). Hao et al. (Citation2020) tested multiple layers of household air filter, coffee filter, bandana, scarf, and pillowcases. When pillowcases, bandana, and scarf were folded into four layers, particle filtration efficiency improved by ∼10%−40% compared to a single layer for experiments conducted at a face velocity of 9.2 cm sec−1, which was similar to the face velocity of this study. Although multiple layers of fibrous materials from household air filters can achieve comparable filtration performance to N95 respirators, they suggested using a combination of fibrous and fabric materials to prevent inhalation of fiber fragments, as we discussed earlier. Konda et al. (Citation2020b) tested multiple layers of chiffon and silk and observed improvement in filtration efficiency when more layers were stacked. They also tested various types of hybrid samples and found that filtration efficiency improved slightly when cotton was used in combination with chiffon or silk, while the benefits from using polypropylene or cotton quilt were more significant. In general, the filtration efficiency of three-layered samples in this study is comparable to that of the materials tested by Gobi et al. (Citation2019). They tested filtration efficiency of particles in the 300 nm to 3 µm size range for three-layered samples made with different proportions of nonwoven polyester + polypropylene top layer, cotton + polyethylene middle layer, and viscose + polypropylene bottom layer. The three-layered samples tested in that study removed 13% to 64% of particles between 300 nm to 1 µm.
Using the two-layered cotton/drapery lining sample as a test mask (overall filtration efficiency of 63%), we evaluated its filtration performance for human exhaled particles (). The size distributions of human exhaled particles from speaking and coughing are adopted from Johnson et al. (Citation2011). We combined our measured size-dependent filtration efficiency for the two-layered cotton/drapery lining mask for submicron particles with two layers of cotton jersey from Drewnick et al. (Citation2021) for particles >1 µm to cover the full size range of respiratory particles, as the experimental setup and filtration curves in our study and in the study by Drewnick et al. (Citation2021) are similar. In a system of two people where one of them is actively speaking or coughing, the total particle number from speaking and coughing in the system will decrease by 84% and 77% when the active person is wearing a mask (). If the other person is also wearing a mask, the number of speech and coughing particles that they are exposed to will reduce by 96% and 92%, respectively, compared to the scenario when both people are not wearing a mask. These are rough estimates and do not consider the fit of the mask, possible shrinkage in particle size by evaporation upon emission, and other factors such as potential emission of micron-scale particulates from friable cellulosic fibers of the cloth mask. Specifically, fitness of the mask was emphasized in previous studies as even 1% of area leak would lead to 50% decrease in filtration efficiency (Drewnick et al. Citation2021; Konda et al. Citation2020a). The drying of particles after exhalation can affect the particle size distribution and corresponding filtration efficiency (Atkinson et al. Citation2009; Krarnes, Büttner, and Ebert Citation1991). Furthermore, paper or cotton masks can generate significant number of particles if rubbed, around 7 − 8 particles/sec (Asadi et al. Citation2020). Nevertheless, our results highlight that homemade masks can provide an acceptable level of protection for the general public and the protection amplifies when every individual wears a mask in an appropriate manner (e.g., good fit and not touching the exterior surface of the mask).
We note several potential limitations of our study. We only measured filtration efficiency under dry conditions (UHP zero air, dry particles, and dry samples). In practice, higher humidity, wet respiratory particles, and wetted materials (e.g., masks become wet from breathing) could impact filtration efficiency of the materials. Also, the tested materials could have differing interactions with particles owing to their varying affinity for water (hydrophilic/hydrophobic) or when they carry electrostatic charges.
4. Conclusions
This work reports size-dependent particle filtration efficiency and overall filtration efficiency of multiple commercial materials, including samples that have not been studied to date. Among the 24 single-layer samples, 4 materials (HEPA filter, MERV 13 filter, Halyard sterilization wrap, and blackout drapery lining) present better filtration compared to the blue surgical mask, which is relatively accessible currently ( and ). HEPA and MERV 13 filters show better filtration efficiency than the other single-layer samples, removing more than 65% of particles overall. Other than filter samples, materials that report an efficient filtration are sterilization wrap manufactured by Halyard followed by blackout drapery lining. These materials show a decent overall filtration efficiency of 63% and 48%, respectively. These materials also exhibit a relatively small pressure drop (similar to surgical mask), making them good candidates for homemade masks.
Most of the materials tested here exhibit insufficient particle filtration efficiency by themselves. However, multiple layers improve the performance and can provide some level of protection. Overall, layered samples tested in this study offer around 50% particle filtration efficiency. Using the two-layered cotton/drapery lining sample as a test mask, we estimate that it can filter out around 80% of human exhaled particles when one person is wearing a mask, and further reduce to 96% when two people are wearing a mask, highlighting the amplified protection of the general public from universal mask wearing.
Following the results here, we recommend using multiple layers when making a homemade mask. With their relatively higher filtration efficiency and comparable pressure drop to commercial surgical masks, Halyard sterilization wrap and blackout drapery lining are recommended to be used as one of the layers in making face masks (). Measurements of material area density, thickness of the sample, and the SEM images () indicate that their better performance could arise from the dense construction of the blackout drapery lining and the three-layered (dense fine fibers in the middle layer, which would play an important role in particle filtration, sandwiched by rough fibers in upper and lower layers that improve material strength) structure of Halyard sterilization wrap. Furthermore, we note that it is important to check if a homemade mask is breathable without any suffocation issue and to avoid fiberglass materials since they can damage the respiratory system if inhaled. Lastly, the fit of the mask should be checked as particles can easily pass through gaps between the face and the mask, resulting in little to no protection.
Figure 6. Pressure drop and overall filtration of each sample. Each sample is labeled by the sample number listed in and colored by the categories shown in .
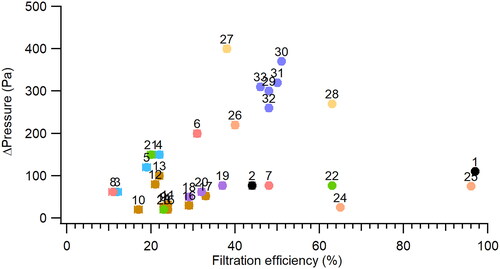
The application of results from this study is not only limited to the current COVID-19 outbreak, but they can be used to guide the choice of materials in making face masks to protect against airborne transmission of viruses in general. For instance, it has been reported that 35% and 64% of influenza A viruses was associated with submicron particles and particles smaller than 2.5 µm, respectively (Yang, Elankumaran, and Marr Citation2011). Furthermore, another study reported a substantial enhancement in survivability of animal viruses (transmissible gastroenteritis virus, swine influenza virus, and avian influenza virus) in 300 − 450 nm particles (Zuo et al. Citation2013). Using face masks in public areas will reduce and slow the spread of diseases by decreasing both the inhalation of infectious particles and the release of viral particles from asymptomatic individuals into the surrounding.
Supplemental Material
Download PDF (1,000.1 KB)Acknowledgments
We thank Christine McCool for helpful discussions on filtration setup and protocols. We also thank Julie Linsey and Katie Copenhaver for providing some of the samples.
Additional information
Funding
References
- Asadi, S., C. D. Cappa, S. Barreda, A. S. Wexler, N. M. Bouvier, and W. D. Ristenpart. 2020. Efficacy of masks and face coverings in controlling outward aerosol particle emission from expiratory activities. Sci. Rep. 10 (1):15665. doi:https://doi.org/10.1038/s41598-020-72798-7.
- ASHRAE. 2017. Method of testing general ventilation air-cleaning devices for removal efficiency by particle size: Standard 52.2-2017. Atlanta, Georgia: ASHRAE.
- Atkinson, J., Y. Chartier, C. L. Pessoa-Silva, P. Jensen, Y. Li, and W.-H. Seto. 2009. Natural ventilation for infection control in health-care settings. Geneva, Swiss: World Health Organization.
- Azimi, P., D. Zhao, and B. Stephens. 2014. Estimates of hvac filtration efficiency for fine and ultrafine particles of outdoor origin. Atmos. Environ. 98:337–46. doi:https://doi.org/10.1016/j.atmosenv.2014.09.007.
- Bałazy, A., M. Toivola, A. Adhikari, S. K. Sivasubramani, T. Reponen, and S. A. Grinshpun. 2006. Do n95 respirators provide 95% protection level against airborne viruses, and how adequate are surgical masks? Am. J. Infect. Control. 34 (2):51–7. doi:https://doi.org/10.1016/j.ajic.2005.08.018.
- Bałazy, A., M. Toivola, T. Reponen, A. Podgórski, A. Zimmer, and S. A. Grinshpun. 2006. Manikin-based performance evaluation of n95 filtering-facepiece respirators challenged with nanoparticles. Ann. Occup. Hyg. 50 (3):259–69. doi:https://doi.org/10.1093/annhyg/mei058.]
- Bowen, L. E. 2010. Does that face mask really protect you? Appl. Biosaf. 15 (2):67–71. doi:https://doi.org/10.1177/153567601001500204.
- CDC. 2020a. Scientific brief: Sars-CoV-2 and potential airborne transmission. Accessed October 5, 2020. https://www.cdc.gov/coronavirus/2019-ncov/more/scientific-brief-sars-cov-2.html.
- CDC. 2020b. Use of masks to help slow the spread of covid-19. Accessed June 28, 2020. https://www.cdc.gov/coronavirus/2019-ncov/prevent-getting-sick/diy-cloth-face-coverings.html.
- Davies, A., K.-A. Thompson, K. Giri, G. Kafatos, J. Walker, and A. Bennett. 2013. Testing the efficacy of homemade masks: Would they protect in an influenza pandemic? Disaster Med Public Health Prep 7 (4):413–8. doi:https://doi.org/10.1017/dmp.2013.43.
- Drewnick, F., J. Pikmann, F. Fachinger, L. Moormann, F. Sprang, and S. Borrmann. 2021. Aerosol filtration efficiency of household materials for homemade face masks: Influence of material properties, particle size, particle electrical charge, face velocity, and leaks. Aerosol Sci. Technol. 55 (1):63–67. doi:https://doi.org/10.1080/02786826.2020.1817846.
- Fazli, T., Y. Zeng, and B. Stephens. 2019. Fine and ultrafine particle removal efficiency of new residential hvac filters. Indoor Air 29 (4):656–69. doi:https://doi.org/10.1111/ina.12566.
- Gobi, N., S. Evangelin, R. Kasthuri, and D. Nivetha. 2019. Multilayer nonwoven fabrics for filtration of micron and submicron particles. J. Text. Eng. Fashion Technol. 5:81–4. doi:https://doi.org/10.15406/jteft.2019.05.00185.
- Hao, W., A. Parasch, S. Williams, J. Li, H. Ma, J. Burken, and Y. Wang. 2020. Filtration performances of non-medical materials as candidates for manufacturing facemasks and respirators. Int. J. Hyg. Environ. Health 229:113582. doi:https://doi.org/10.1016/j.ijheh.2020.113582.
- Hecker, R., and K. Hofacre. 2008. Development of performance data for common building air cleaning devices (final report no. Epa/600/r-08/013). NC: US Environmental Protection Agency, Office of Research and Development/National Homeland Security Research Center Research Triangle Park,.
- Hinds, W. C. 1999. Aerosol technology: Properties, behavior, and measurement of airborne particles. New York: Wiley.
- Johnson, G. R., L. Morawska, Z. D. Ristovski, M. Hargreaves, K. Mengersen, C. Y. H. Chao, M. P. Wan, Y. Li, X. Xie, D. Katoshevski, et al. 2011. Modality of human expired aerosol size distributions. J. Aerosol Sci. 42 (12):839–51. doi:https://doi.org/10.1016/j.jaerosci.2011.07.009.
- Konda, A., A. Prakash, G. A. Moss, M. Schmoldt, G. D. Grant, and S. Guha. 2020a. Aerosol filtration efficiency of common fabrics used in respiratory cloth masks. ACS Nano 14 (5):6339–47. doi:https://doi.org/10.1021/acsnano.0c03252.
- Konda, A., A. Prakash, G. A. Moss, M. Schmoldt, G. D. Grant, and S. Guha. 2020b. Response to letters to the editor on aerosol filtration efficiency of common fabrics used in respiratory cloth masks: Revised and expanded results. ACS Nano. 14 (9):10764–70. doi:https://doi.org/10.1021/acsnano.0c04897.
- Krarnes, J., H. Büttner, and F. Ebert. 1991. Submicron particle generation by evaporation of water droplets. J. Aerosol Sci. 22:S15–S18. doi:https://doi.org/10.1016/S0021-8502(05)80023-0.
- Lai, C.-C., T.-P. Shih, W.-C. Ko, H.-J. Tang, and P.-R. Hsueh. 2020. Severe acute respiratory syndrome coronavirus 2 (sars-cov-2) and coronavirus disease-2019 (covid-19): The epidemic and the challenges. Int. J. Antimicrob. Agents 55 (3):105924. doi:https://doi.org/10.1016/j.ijantimicag.2020.105924.
- Lammers, D. T., I. F. Jones, C. W. Marenco, K. R. Morte, J. M. Mcclellan, M. J. Eckert, and J. R. Bingham. 2021. Safety code blue! Assessing the use of blue surgical sterilization wrap for homemade respirator masks during the covid-19 crisis. Am. J. Infect. Control. 49 (2):274–5. doi:https://doi.org/10.1016/j.ajic.2020.07.021.
- Lednicky, J. A., M. Lauzardo, Z. Hugh Fan, A. Jutla, T. B. Tilly, M. Gangwar, M. Usmani, S. N. Shankar, K. Mohamed, A. Eiguren-Fernandez, et al. 2020. Viable SARS-CoV-2 in the air of a hospital room with COVID-19 patients. Int. J. Infect. Dis. 100:476–82. doi:https://doi.org/10.1016/j.ijid.2020.09.025.
- Morawska, L., and J. Cao. 2020. Airborne transmission of SARS-CoV-2: The world should face the reality. Environ. Int. 139:105730. doi:https://doi.org/10.1016/j.envint.2020.105730.
- Morawska, L., and D. K. Milton. 2020. It is time to address airborne transmission of coronavirus disease 2019 (Covid-19). Clin. Infect. Dis. 71 (9):2311–3. doi:https://doi.org/10.1093/cid/ciaa939.
- National Academies of Sciences, Engineering and Medicine. 2020. Rapid expert consultations on the covid-19 pandemic: March 14, 2020–April 8, 2020. Washington (DC): National Academies Press (US). http://www.ncbi.nlm.nih.gov/books/NBK556962/.
- Nemer, M., A. Grillet, A. L. Sanchez, and K. M. Emmer. 2020. Alternative materials for mask construction by the public. Albuquerque, NM (United States): Sandia National Lab.(SNL-NM).
- Oude Vrielink, T. J. C., and J. H. Meijer. 2020. Using surgical wrapping material for the fabrication of respirator masks. PLOS One. 15 (7):e0236239. doi:https://doi.org/10.1371/journal.pone.0236239.
- Pei, C., Q. Ou, S. C. Kim, S.-C. Chen, and D. Y. H. Pui. 2020. Alternative face masks made of common materials for general public: Fractional filtration efficiency and breathability perspective. Aerosol Air Qual. Res. 20 (12):2581–2591. doi:https://doi.org/10.4209/aaqr.2020.07.0423.
- Prather, K. A., C. C. Wang, and R. T. Schooley. 2020. Reducing transmission of SARS-CoV-2. Science 368 (6498):eabc6197. doi:https://doi.org/10.1126/science.abc6197.
- Rengasamy, S., B. Eimer, and R. E. Shaffer. 2010. Simple respiratory protection-evaluation of the filtration performance of cloth masks and common fabric materials against 20-1000 nm size particles. Ann. Occup. Hyg. 54 (7):789–98. doi:https://doi.org/10.1093/annhyg/meq044.
- Shakya, K. M., A. Noyes, R. Kallin, and R. E. Peltier. 2017. Evaluating the efficacy of cloth facemasks in reducing particulate matter exposure. J. Expo. Sci. Environ. Epidemiol. 27 (3):352–57. doi:https://doi.org/10.1038/jes.2016.42.
- Shou, D., J. Fan, L. Ye, H. Zhang, X. Qian, and Z. Zhang. 2015. Inverse problem of air filtration of nanoparticles: Optimal quality factors of fibrous filters. J. Nanomater. 2015:168392. https://doi.org/10.1155/2015/168392 doi:https://doi.org/10.1155/2015/168392.
- Stephens, B., and J. A. Siegel. 2013. Ultrafine particle removal by residential heating, ventilating, and air-conditioning filters. Indoor Air 23 (6):488–97. doi:https://doi.org/10.1111/ina.12045.
- Van Der Sande, M., P. Teunis, and R. Sabel. 2008. Professional and home-made face masks reduce exposure to respiratory infections among the general population. PLoS One 3 (7):e2618. doi:https://doi.org/10.1371/journal.pone.0002618.
- WHO. 2020a. Advice on the use of masks in the context of COVID-19: Interim guidance. Geneva, Swiss: World Health Organization.
- WHO. 2020b. Country & technical guidance – Coronavirus disease (COVID-19). Geneva, Swiss: World Health Organization.
- Yang, W., S. Elankumaran, and L. C. Marr. 2011. Concentrations and size distributions of airborne influenza a viruses measured indoors at a health centre, a day-care centre and on aeroplanes. J. R. Soc. Interface 8 (61):1176–84. doi:https://doi.org/10.1098/rsif.2010.0686.
- Zhao, M., L. Liao, W. Xiao, X. Yu, H. Wang, Q. Wang, Y. L. Lin, F. S. Kilinc-Balci, A. Price, L. Chu, et al. 2020. Household materials selection for homemade cloth face coverings and their filtration efficiency enhancement with triboelectric charging. Nano Lett. 20 (7):5544–5552. doi:https://doi.org/10.1021/acs.nanolett.0c02211.
- Zuo, Z., T. H. Kuehn, H. Verma, S. Kumar, S. M. Goyal, J. Appert, P. C. Raynor, S. Ge, and D. Y. H. Pui. 2013. Association of airborne virus infectivity and survivability with its carrier particle size. Aerosol Sci. Technol. 47 (4):373–82. doi:https://doi.org/10.1080/02786826.2012.754841.