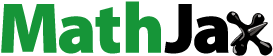
Abstract
Transparent barriers were installed as a response to the SARS-COV-2 pandemic in many customer-facing industries. Transparent barriers are an engineering control that intercept particles traveling between customers and workers. Information on the effectiveness of these barriers against aerosols is limited. In this study, a cough simulator was used to represent a cough from a customer. Two optical particle counters were used (one on each side of the barrier, labeled customer and worker) to determine the number of particles that migrated around a transparent barrier. Ten configurations were tested with six replicates for both sitting and standing scenarios, representing nail salons and grocery stores, respectively. Barrier efficiency was calculated using a ratio of the particle count results (customer/worker). Barriers had better efficiency (up to 93%) when its top was 9 to 39 cm above cough height and its width was at least 91 cm. Barriers that extended 91 cm above table height for both scenarios blocked 71% or more of the particles between 0.35–0.725 µm and 68% for particles between 1 to 3 µm. A barrier that blocked an initial cough was effective at reducing particle counts. While the width of the barriers was not as significant as the height in determining barrier efficiency it is important that a barrier be placed where interactions between customers and workers are most frequent. Bystander exposure was not taken into consideration along with other limitations.
EDITOR:
1. Introduction
SARS-CoV-2, a novel virus that causes COVID-19, is transmitted mostly through close contact. Close contact is defined by the CDC as: “someone within six feet of an infected individual for a cumulative total of 15 min or more within a 24 h period (CDC Citation2021a).” The cumulative time of exposure to infected individuals has been associated with COVID-19 risk (CDC Citation2021b; Pringle et al. Citation2020). Employees in service industries, such as nail technicians and cashiers, that spend extended (e.g., manicures take 30 to 60 min) time with multiple customers or spend a short time with a very large number of customers, are at greater risk than other workers (CDC Citation2020c; Faces Spa Citation2020). Despite the availability of safe and effective vaccines against COVID-19, employee exposure risk remains a concern because COVID-19 cases continue to occur (including the now predominant Delta variant), not everyone has been vaccinated, and mask mandates have been reduced (CDC Citation2021c; Markowitz Citation2021).
Transparent barriers, generally made of polycarbonate or polymethylmethacrylate (PMMA), are recommended by the Centers for Disease Control and Prevention (CDC) (CDC Citation2020a) and the Occupational Safety and Health Administration (OSHA) (OSHA Citation2021) as engineering controls to limit the transmission of SARS-CoV-2 to workers. Evidence-based recommendations are needed on the effectiveness of barriers and size requirements for employees in customer-facing industries such as the 155,300 nail salon technicians, 3.6 million grocery and convenience store cashiers, and other workers (Bureau of Labor Statistics Citation2021). One purpose for barriers is to reduce the number of inhalable (<100 µm aerodynamic equivalent diameter (AED)), thoracic (<10 to 15 µm) and respirable (<5 µm AED) particles that could migrate from an infected customer to a worker on the opposite side of a barrier, or from an infected worker to a customer (Eykelbosh Citation2020; Milton Citation2020). How barriers affect particle migration at the submicron fraction is vital as research indicates that infectious virions can be contained in particles smaller than 1 µm (Santarpia et al. Citation2021).
Transparent barriers provide desirable qualities as an intervention method. These barriers serve as a semi-permanent engineering control, enforce physical distancing, and are easily sanitized (Environmental Health and Safety University of Washington Citation2020). However, evidence is limited on the effectiveness of transparent barriers for preventing SARS-CoV-2 transmission and the appropriate size of barriers to provide the best efficiency for particle reduction (CDC Citation2020b). For instance, the University of Washington proposed barrier heights, from the floor to the top of the barrier, based on the tallest individuals (Environmental Health and Safety University of Washington Citation2020).
The objective of this laboratory study was to determine the efficiency of transparent barriers in blocking airborne particles and to evaluate efficiency against barrier size when two people are across from each other. Most particles that were produced by this study’s cough simulator were in the respirable range which travel into the lungs during breathing; some particles were in the inhalable and thoracic ranges which likely deposit into the nose, mouth, and throat. The size ranges of particles generated by the simulator in this study are similar to that of an adult human cough, with a count median diameter between 0.57 and 0.71 μm (average 0.63 μm, SD 0.05) (Lindsley et al. Citation2013). Size ranges for individual coughs vary greatly and have been measured from 0.01 to 500 µm (Gralton et al. Citation2011). Although larger particles (up to 100 µm) can be inhaled, most are not. Cloth masks, if worn properly, reduce the number of larger particles (>3 µm) both released and inhaled by the wearer, but for smaller particles additional protection is needed (Akhtar et al. Citation2020; Lindsley et al. Citation2021).
2. Materials and methods
The purpose of this research was to assess the potential for a barrier to reduce respiratory aerosol particle exposure when two people are interacting directly across the barrier from each other. Transparent barriers of various sizes were the only interventions considered in this study.
2.1. Cough simulator
The cough simulator is made from a metal bellows and automated by a linear motor (Model STA2506; Copley Controls, Canton, MA). It has been described previously (Lindsley et al. Citation2021; Lindsley et al. Citation2019; Lindsley et al. Citation2014; Lindsley et al. Citation2013). Briefly, the cough aerosol expelled by the simulator is produced from a 28% Potassium Chloride (KCl) solution generated in a single-jet Collison nebulizer (ARGCNB1, CH Technologies (USA), Inc. Westwood, NJ) under 172 Kilopascal. The air brush previously employed to generate larger particles was not used in this study. The expelled aerosol for each “cough” had a peak flow rate of 11 L/s and a volume of 4.2 L. The flow rate and volume were similar to influenza patients as determined by Lindsley et al. (Lindsley et al. Citation2012). The cough simulator was chosen to avoid the use of any potential bioaerosol introduction that would be produced if using live human subjects.
2.2. Transparent barriers
The transparent barriers were clear acrylic sheets (OPTIX, Plaskolite, Columbus, OH). The acrylic sheets were 0.3 cm thick and cut into nine sizes. The heights were 61, 91 or 122 cm, widths were 61, 91 or 122 cm. A rectangular opening, 10 cm high by 31 cm wide, was cut into the bottom center of each test barrier to represent access for transactions. A control trial was conducted without the use of a barrier for use as a comparison for calculating efficiency. For data analysis, the vertical height between the top of the barrier and the mouth of the cough manikin was used.
2.3. Aerosol counts
The aerosols produced by the cough simulator were counted by factory calibrated GRIMM optical particle counters (OPC, Model 1.108; Grimm Technologies, Inc., Douglasville, GA). Two OPCs were used for each experimental run, denoted as customer OPC and worker OPC. Prior to sampling, each OPC was synced to the same computer to ensure matching times for each data point collected. Prior to initial data collection both OPCs displayed similar pre- and post- cough particle count measurements. The customer OPC was placed 30.5 cm from the front of the “mouth” of the cough simulator on the same side of the barrier with the inlet port directly in line at the same height. The worker OPC was placed on the far side of the barrier. The OPCs collected data every six seconds for particles in the size range from 0.35 to 22.0 µm in 15 size bins. For analysis, particle sizes were categorized into small (0.35–0.725 µm), moderate (0.9–2.5 µm), large (3.5–6.25 µm) and extra-large (8.75–22.5 µm).
2.4. Isolation room
The experiment was performed in an isolation room (). This isolation room was originally designed as a model hospital airborne infection isolation room (Thatiparti, Ghia, and Mead Citation2016). Physical measurements of the isolation room were 4.9 m by 4.3 m with a ceiling height of 2.4 m for a total of 58 m3. The isolation room ventilation rate was set to two air changes per hour (ACH) and confirmed by an initial sulfur hexafluoride (SF6) decay test, which indicated an ACH of 2.40, and multiple measurements throughout the study using a balometer (TSI/ALNOR EBT731, Shoreview, MN), which ranged from 1.81 to 2.11 ACH. Around 2 ACH is the calculated value for office spaces (ASHRAE Citation2004). Although the experimental room is not specific to our target workplaces, the air change rate used was thought to be conservative and represented a worst-case scenario. The supply air vents had measured readings, on average, of 17 L/s (36 cfm) for the vent behind the cough manikin and 16 L/s (34 cfm) for the vent opposite the manikin. The return air vents had measured exhaust readings, on average, of 9.9 L/s (21 cfm) for the vent opposite the manikin and 11.3 L/s (24 cfm) for the vent in the supply closet, which was open, as shown in . A smoke generator showed that the air generally flowed from behind the cough simulator past the barrier. Using the physical dimensions and airflow measurements, an air change rate (ACH) was calculated by dividing the greater of total exhaust or supply (m3/hr) by the area volume (m3).
2.5. Seated and standing workstation design
Two scenarios were analyzed to represent typical interactions: seated and standing (). A seated scenario represented an interaction between a worker and customer seated at a table, such as at a nail salon manicurist table. The standing scenario represented an interaction between a worker and customer standing at a counter, such as at a cashier counter or grocery checkout. The cough simulator was placed 76 cm from the barrier and 30.5 cm from the customer OPC. The worker OPC was placed 46 cm from the barrier on the opposite side.
Figure 2. (a) Seated and (b) Standing interaction shown with a cough simulator, worker, and customer optical particle counters and transparent barrier. h indicates the height of the barrier, which was 61, 91 or 122 cm from the table. The barrier widths, not shown, were 61, 91 or 122 cm. Table dimensions were 76 cm tall (for sitting) and 91 cm tall (for standing), 61 cm wide, and 102 cm long. A rectangular opening was cut into the bottom center of each barrier that was 10 cm tall and 31 cm wide.
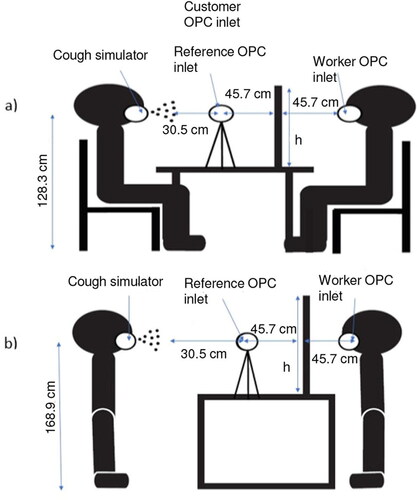
It is expected that customers and employees shift positions throughout an interaction. It was not feasible to represent this movement due to variation between individuals and situations. The OPCs were attached to tripods to elevate them to the correct height. The cough simulator was elevated by a constructed 80/20® (Columbia City, IN, USA) aluminum frame.
The sitting scenario represented an exposure at a salon while conducting a manicure. Since more women than men visit salons (72% compared to 52%), a woman’s average height was used for the sitting workstation scenario (Sharma et al. Citation2018). The cough simulator was positioned at a seated mouth height of a 95th percentile woman. This height represented an increased risk of exposure—a taller person would cough over a shorter barrier. The heights for the worker OPC and the cough simulator were determined from anthropometric data from the American Industrial Hygiene Association (AIHA) Engineering Reference Manual (Estill Citation1999). shows the heights at which each instrument was placed for both scenarios. To calculate cough height, seated eye height (79 cm) was added to the sitting knee height (55 cm) and 5 cm were subtracted to estimate the mouth position (128 cm). In the sitting scenario, the table was 76 cm high, 61 cm wide, and 102 cm long, similar to several commercial manicurist tables (Easy Nail Tech). Nail salon workstations are, however, diverse. The vertical distances from the top of the barrier to the mouth of the coughing manikin were 9, 39 and 70 cm for the three barrier heights.
For the standing workstations, the cough simulator was placed at a height chosen based on the 95th percentile height for men (Estill Citation1999). Male stature at the 95th percentile was considered the highest risk of exposure and was used as a worst-case scenario for men and women. To estimate mouth position, 15 cm was subtracted from male stature (184 cm) for a cough height of 169 cm. The counter was 91 cm high with a depth of 61 cm based on the American National Safety Institute (ANSI) and American with Disabilities Act (ADA) countertop requirements for sales counters (United States Access Board; US Department of Justice Citation1999). The vertical distance between the top of the barrier to the mouth of the coughing manikin was 16.5 cm below the mouth, 14 and 44.5 cm above the mouth.
2.6. Study trials
Each trial consisted of three 10-min phases. During the first phase background particle counts were measured. During the second phase particle counts were obtained while the cough simulator was activated to produce two coughs. The final phase was used to clean the air using a portable high-efficiency particulate air (HEPA) filtered unit (VACOMAGUS, Atrix, Burnsville, MN, USA) at 280 cubic feet per minute (cfm) to return aerosol levels in the room to almost initial background measures. For each scenario (sitting and standing) the order of barriers was randomized. Six replicates of each of the ten barrier conditions (nine barriers and no barrier) were performed resulting in 60 trials for each scenario.
2.7. Statistical analysis
Summary statistics were provided as median and range for the variable of interest, efficiency, with right-skewed distribution. Efficiency was quantified using the ratios of the worker OPC count to the customer OPC count among ten barrier conditions to determine the percentage reduction. The background particle counts were adjusted and subtracted before calculating the ratios.
(1)
(1)
where particle counts for the worker OPC with and without the barrier (
and
) and for the customer OPC (
and
) were obtained after subtracting the background counts.
A median regression was carried out to estimate differences in the medians of the outcome or dependent variable, i.e., efficiency, and to determine how two independent variables, i.e., barrier width and height, in combination, influence the outcome in small, moderate, and large-sized particles and in each scenario. Corresponding 95% confidence intervals (CI) and p-values were also provided. Median regression is recommended when the outcome does not follow normal distribution or potential outliers are found to influence the mean relative to the median, both of which were existing for our data (Koenker and Hallock Citation2001). Additionally, multiple comparisons were performed to investigate significant differences of the outcome variable among all possible combinations of barrier settings. All statistical tests were two-sided at the 0.05 significance level and analyses were conducted in SAS version 9.4 (SAS Institute, Cary, NC, USA).
3. Results
Median and range for efficiency with right-skewed distributions are provided (). For the small sized particles (0.35–0.725 µm), barrier median efficiencies ranged from 71% to 86% for barriers where the top was at least 14 cm above cough height and all widths when compared to no barrier in the standing scenario, while the range was 83% to 93% for barriers at all heights and widths in the sitting scenario. For moderate sized particles (0.9–2.5 µm), barrier median efficiencies ranged from 68% to 87% for barriers with tops at least 14 cm above cough height for standing and from 71% to 94% for barriers with tops at least 9 cm above cough height for sitting. While standing, the 61 cm high barrier was 16.5 cm below cough height, had low efficiency, and was not significantly different from the no barrier configuration. With respect to the largest sized particles (3.5–6.25 µm) we analyzed, the median efficiencies for barriers that extended above cough height and at least 91 cm wide were 61% or greater for standing and 69% or greater for sitting (Table S1). The lower efficiency for larger particles is likely due to the lower amount produced in that size range by the cough simulator. presents the geometric means (GMs) of particle counts by category size and OPC location for one randomly selected scenario. The GMs of customer OPC were greater than the values of worker OPC in each scenario. The smallest particles were the most abundant, and differences between customer and worker OPC were greatest for the small and moderate sized particles.
Figure 3. Geometric means of particle counts by particle size and OPC location for the configuration of 14 cm above cough height and 91 cm width in standing, and 39 cm above cough height and 91 cm width in sitting.
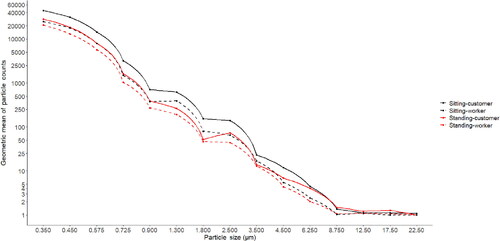
Table 1. Collection efficiency by particle size in standing and sitting scenarios.
For the smallest particles (Table S2), the barrier that extended above cough height blocked aerosols by 76 to 90% compared with no barrier (all p-values < 0.001). For the standing scenario, the efficiencies of all barriers that extended at least 14 cm above cough height did not significantly differ from each other. As expected, the barrier that was 16.5 cm below cough height in the standing scenario was not significantly different from no barrier. The results of efficiencies for moderate sized particles are provided in Table S3. Most barriers that extended at least 14 cm above cough height in the standing scenario significantly blocked the aerosols relative to no barrier. In the sitting scenario, all barrier settings with moderate size outperformed no barrier by 86 to 93% (p-values < 0.001).
4. Discussion
Physical transparent barriers provide benefits for workers by reducing the number of particles from a customer’s cough that make it to the worker. This laboratory simulation showed that barriers reduced particle count by at least 71% for the smallest particles when the top was located at least 9 cm above cough height.
To be effective a barrier top needs to be above cough height so that it can block the exhaled air from a cough. Our analysis showed that the best performing barriers had: (1) tops at least 9 cm above cough height, (2) preferably tops 15 cm above cough height while standing and (3) tops 39 cm above cough height while sitting. Barriers that were shorter than cough height did not protect and were not different than not using a barrier. The tallest barriers with tops 122 cm above table height that were used in both scenarios did not provide improved efficiency compared to those whose tops were 91 cm tall; there was no added benefit from increasing the barrier height beyond 91 cm.
When considering barrier width, we excluded barriers that were below cough height for the standing scenario. The 91 cm wide barriers performed better than the 61 cm wide barriers for small and moderate sized particles in both the sitting and standing scenarios. The 91 cm wide barrier performed statistically better than the 122 cm wide barriers for moderate sized particles. There was not a statistically significant difference between barrier widths of 91 cm and 122 cm for the small sized particles. Although all the barrier widths were more efficient than no barrier, 91 cm wide barriers were preferred because they were 3 to 13% more efficient than the 61 cm and 122 cm.
It has been shown that airflow is impacted by vent and barrier placement (Lee and Awbi Citation2004). There is some evidence that desk shields, a type of barrier, may increase transmission among school students and their families (Lessler et al. Citation2021). Ventilation configurations will vary greatly at workplaces. Larger barriers were not shown to be statistically better than smaller barrier sizes in our study. For both height and width, the middle-sized barriers had greater efficiencies and sometimes efficiencies that were statistically greater than the largest heights and widths. The largest barriers may have interfered with the room air movement. In this study, air was moving in the room at approximately two ACH, typical for a store or office (ASHRAE Citation2004). The largest barrier may have reduced mixing and may have entrained the exhaled cough to move in the direction that was the least blocked. For instance, a wider barrier may have forced the exhaled cough to go over a short height. Proper ventilation will aid in preventing smaller particles, which remain in the air longer, from remaining in one location.
To provide worker protection, it is preferred that barriers encompass the location where a customer primarily interacts with a worker. For example, if a customer at a grocery store checkout needs to load groceries and then make a payment, it is preferred that barriers are erected at each interaction location. A good measure to ensure the greatest range of protection for height is to use the 95th percentile of height for males for both sitting and standing interactions. The 95th percentile cough height for U.S. males is 168.9 cm. Therefore, the top of the transparent barriers for standing interactions shown to be the most effective in this study was 183 cm from the floor. For sitting interactions at nail salons, the barrier height shown to be the most effective in this study was 140 cm from the floor based on the 95th percentile for sitting height for females with a chair height of 56 cm (Estill Citation1999). Female height was used because of the greater percentage of female workers and customers in the nail salon industry (Sharma et al. Citation2018). However, to offer the optimal community protection, the sitting barrier heights of 150 cm from the floor are preferred as they account for the 95th percentile sitting height for males using a 58 cm chair height.
There are some major limitations in this experiment. First, all experiments were performed in an isolation room that provided consistent ventilation rates for comparison which may not be representative of occupational settings. Further studies with other general air movement patterns are needed. A diversity of particle production and dispersion occurs when talking or breathing compared to coughing. However, only coughing was considered for this study. There is a wide variation in workstation configurations. The scenarios used here do not accurately reflect all real-world situations and a larger access opening is likely necessary at salons for performing manicures. When manicures are being performed through these access windows air flow may be altered. The experiments did not consider worker or customer movement and only measured a potential exposure from two direct linear coughs. The OPCs collected data at 6-sec intervals, so a travel time associated with the aerosolized particles could not be calculated. The cough simulator did not produce many particles in the larger size ranges. We collected data for 10 min after each cough. Since smaller particles remain in the air for longer, the two ACH we used aided in removing the small particles and prevented them from migrating and settling. This study did not evaluate the potential increase risk of exposure to others in the same area when the particles were forced to circumvent the barriers by the top and sides. Finally, masks or other transmission control methods were not considered in this work. Barriers were solely considered to assess this engineering control. Other control methods used in combination with barriers would likely provide additional reductions in exposure.
In summary, laboratory simulations demonstrate that barriers can reduce cough particle exposures in locations where workers interact with others and especially for situations where there is extended interaction time (e.g., nail salons). Barriers can be used in conjunction with other prevention recommendations, e.g., strategies to improve ventilation, vaccination, and masks. While barriers designed with tops to be at least 9 cm above the height of the expected customer’s mouth were shown to be effective, barriers tops that were 39 cm above the customer’s mouth were more effective. Efficiency above 71% for the smallest particles can be achieved for most workers by using barriers that extend 183 cm from the floor for standing interactions and 150 cm from the floor for sitting interactions. The most effective width was 91 cm for the scenarios studied; wider barriers were not shown to improve barrier efficiency Barriers with tops taller than 39 cm above the customer’s mouth height were not shown to improve barrier efficiency.
Supplemental Material
Download MS Word (33.2 KB)Acknowledgments
The authors would like to thank William Lindsley, Duane Hammond, Kevin Menchaca, Ken Mead, Brian Curwin and Matt Dahm with their assistance and feedback throughout the experimental process.
Additional information
Funding
References
- Akhtar, J., A. L. Garcia, L. Saenz, S. S. Kuravi, and K. Fangjun; Kota. 2020. Can face masks offer protection from airborne sneeze and cough droplets in close-up, face-to-face human interactions? – A quantitative study. Phys. Fluids 32:127112. doi:10.1063/5.0035072.
- ASHRAE. 2004. Ventilation for acceptable indoor air quality. In Minimum ventilation rates in breathing zone, 13. Atlanta, GA: American Society of Heating Refrigerating and Air-conditioning Engineers, Inc. https://www.ashrae.org/File%20Library/Technical%20Resources/Standards%20and%20Guidelines/Standards%20Addenda/62-2001/62-2001_Addendum-n.pdf.
- Bureau of Labor Statistics, United States Department of Labor. 2021. Occupational outlook handbook. Accessed June 4, 2021. https://www.bls.gov/ooh/.
- CDC. 2020a. Covid-19 employer information for office buildings. In Coronavirus disease 2019 (COVID-19). Accessed April 22, 2021. https://www.cdc.gov/coronavirus/2019-ncov/community/office-buildings.html.
- CDC. 2020b. Standard operating procedure (sop) for triage of suspected covid-19 patients in non-us healthcare settings: Early identification and prevention of transmission during triage. Accessed May 12, 2021. https://www.cdc.gov/coronavirus/2019-ncov/hcp/non-us-settings/sop-triage-prevent-transmission.html.
- CDC. 2020c. What grocery and food retail workers need to know about covid-19. Accessed July 10, 2020. https://www.cdc.gov/coronavirus/2019-ncov/community/organizations/grocery-food-retail-workers.html
- CDC. 2021a. Appendices, in contact tracing. Accessed July 18, 2021. https://www.cdc.gov/coronavirus/2019-ncov/php/contact-tracing/contact-tracing-plan/appendix.html.
- CDC. 2021b. How covid-19 spreads. In COVID-19 Your Health. Accessed May 12, 2021. https://www.cdc.gov/coronavirus/2019-ncov/prevent-getting-sick/how-covid-spreads.html.
- CDC. 2021c. Variant proportions. In COVID Data Tracker. Accessed July 26, 2021. https://covid.cdc.gov/covid-data-tracker/#variant-proportions..
- Easy Nail Tech. How wide & tall should a manicure table be correct dimensions. Accessed July 27, 2020. https://easynailtech.com/manicure-table-dimensions/.
- Environmental Health and Safety University of Washington. 2020. Uw guidance for plexiglass barriers in the workplace. https://ehs.washington.edu/system/files/resources/COVID-19-plexiglass-barriers-workplace.pdf.
- Estill, C. 1999. Ergonomics. In Aiha Engineering Reference Manual, 137–57.
- Eykelbosh, A. 2020. Physical barriers for covid-19 infection prevention and control in commercial settings [blog]. Vancouver, BC: National Collaborating Center for Environmental Health. https://ncceh.ca/content/blog/physical-barriers-covid-19-infection-prevention-and-control-commercial-settings.
- Gralton, J., E. Tovey, M.-L. McLaws, and W. D. Rawlinson. 2011. The role of particle size in aerosolised pathogen transmission: A review. J. Infect. 62 (1):1–13. doi:10.1016/j.jinf.2010.11.010.
- Koenker, R., and K. F. Hallock. 2001. Quantile regression. J. Econ. Perspect. 15 (4):143–6. doi:10.1257/jep.15.4.143.
- Lee, H., and H. B. Awbi. 2004. Effect of internal partitioning on indoor air quality of rooms with mixing ventilation – basic study. Build. Environ. 39 (2):127–41. doi:10.1016/j.buildenv.2003.08.007.
- Lessler, J., M. K. Grabowski, K. H. Grantz, E. Badillo-Goicoechea, C. J. E. Metcalf, C. Lupton-Smith, A. S. Azman, and E. A. Stuart. 2021. Household covid-19 risk and in-person schooling. Science 372 (6546):1092–7. doi:10.1126/science.abh2939.
- Lindsley, W. G., F. M. Blachere, B. F. Law, D. H. Beezhold, and J. D. Noti. 2021. Efficacy of face masks, neck gaiters and face shields for reducing the expulsion of simulated cough-generated aerosols. Aerosol. Sci. Technol. 55 (4):449–57. doi:10.1080/02786826.2020.1862409.
- Lindsley, W. G., F. M. M. Blachere, L. Tia, D. T. Neu, A. Mnatsakanova, S. B. Martin, Jr., K. R. Mead, and J. D. Noti. 2019. Efficacy of an ambulance ventilation system in reducing ems worker exposure to airborne particles from a patient cough aerosol simulator. J. Occup. Environ. Hyg. 16 (12):804–16. doi:10.1080/15459624.2019.1674858.
- Lindsley, W. G., J. D. Noti, F. M. Blachere, J. V. Szalajda, and D. H. Beezhold. 2014. Efficacy of face shields against cough aerosol droplets from a cough simulator. J. Occup. Environ. Hyg. 11 (8):509–18. doi:10.1080/15459624.2013.877591.
- Lindsley, W. G., T. A. Pearce, J. B. Hudnall, K. A. Davis, S. M. Davis, M. A. Fisher, R. Khakoo, J. E. Palmer, K. E. Clark, I. Celik, et al. 2012. Quantity and size distribution of cough-generated aerosol particles produced by influenza patients during and after illness. J. Occup. Environ. Hyg. 9 (7):443–9. doi:10.1080/15459624.2012.684582.
- Lindsley, W. G., J. S. Reynolds, J. V. Szalajda, J. D. Noti, and D. H. Beezhold. 2013. A cough aerosol simulator for the study of disease transmission by human cough-generated aerosols. Aerosol. Sci. Technol. 47 (8):937–44. doi:10.1080/02786826.2013.803019.
- Markowitz, A. 2021. State-by-state guide to face mask requirements. In Healthy Living. Accessed July 15, 2021. https://www.aarp.org/health/healthy-living/info-2020/states-mask-mandates-coronavirus.html.
- Milton, D. K. 2020. A rosetta stone for understanding infectious drops and aerosols. J. Pediatric Infect. Dis. Soc. 9 (4):413–5. doi:10.1093/jpids/piaa079.
- OSHA. 2021. Protecting workers: Guidance on mitigating and preventing the spread of covid-19 in the workplace. Accessed June 2, 2021. https://www.osha.gov/coronavirus/safework
- Pringle, J. C., J. Leikauskas, S. Ransom-Kelley, B. Webster, S. Santos, H. Fox, S. Marcoux, P. Kelso, and N. Kwit. 2020. Covid-19 in a correctional facility employee following multiple brief exposures to persons with covid-19—vermont, July–August 2020. Morb. Mortal Wkly Rep. 69 (43):1569–70. doi:10.15585/mmwr.mm6943e1.
- Santarpia, J. L., V. L. Herrera, D. N. Rivera, S. Ratnesar-Shumate, S. P. Reid, D. N. Ackerman, P. W. Denton, J. W. S. Martens, Y. Fang, N. Conoan, et al. 2021. The size and culturability of patient-generated sars-cov-2 aerosol. J. Expo. Sci. Environ. Epidemiol. . doi:10.1038/s41370-021-00376-8.
- Sharma, P., S. Waheed, V. Nguyen, L. Stepick, R. Orellana, L. Katz, S. Kim, and K. Lapira. 2018. Nail file: A study of nail salon workers and industry in the united states. Ucla labor center and california healthy nail salon https://www.labor.ucla.edu/wp-content/uploads/2018/11/NAILFILES_FINAL.pdf.
- Spa, F. 2020. How long does a manicure take? In Faces. NE. https://facesspa.com/blog/how-long-does-a-manicure-take/.
- Thatiparti, D. S., U. Ghia, and K. R. Mead. 2016. Computational fluid dynamics study on the influence of an alternate ventilation configuration on the possible flow path of infectious cough aerosols in a mock airborne infection isolation room. Sci. Technol. Built Environ. 23 (2):355–66. doi:10.1080/23744731.2016.1222212.
- United States Access Board. Chapter 9: Built in elements. https://www.access-board.gov/guidelines-and-standards/buildings-and-sites/about-the-ada-standards/background/ibc-comparison/chapter-9.
- US Department of Justice. 1999. Ada guide for small business. Accessed July 29, 2020. https://www.ada.gov/smbusgd.pdf.