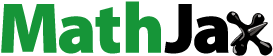
Abstract
Aerosol particles generated by dental procedures could facilitate the transmission of infectious diseases and contain carcinogen particles. Such particles can penetrate common surgical masks and reach the lungs, leading to increased risk for dental care professionals. However, the risk of inhaling contaminated aerosol and the effectiveness of aerosol reduction measures in dental offices remain unclear. The present study aimed to quantify aerosols produced by drilling and scaling procedures and to evaluate present recommendations for aerosol reduction. The concentration of aerosol particles released from the mock scaling and drilling procedures performed on a dental mannequin were measured using a TSI Optical Particle Sizer (OPS 3330) during 15-min sessions carried out in a single-patient examination room. Using the mock drilling procedure as the aerosol source, the aerosol reduction performance of two types of high-volume evacuators (HVEs) and a commercial off-the-shelf air purifier was evaluated in a simulated clinical setting. The use of either HVEs or the air purifier individually reduced the aerosol accumulated over the course of a 15-min drilling procedure at a reduction rate of 94.8 to 97.6%. Using both measures simultaneously raised the reduction rate to 99.6%. The results show that existing HVEs can effectively reduce aerosol concentration generated by a drilling procedure and can be further improved by using an air purifier. Following current regulatory guidelines can ensure a low risk of inhaling contaminated aerosol for dentists, assistants, and patients.
Copyright © 2022 American Association for Aerosol Research
EDITOR:
Introduction
Dental scaling and restorative procedures are common aerosol generating procedures (AGPs) in dental practices. The ultrasonic scalers and high-speed handpieces used in these procedures produce a visible mist composed of splatter and aerosol particles. The droplets that constitute splatter are mostly visible to the naked eye and typically settle on nearby surfaces after spending a few seconds airborne (Micik et al. Citation1969). In contrast, aerosol particles are too small to be seen unaided, can drift far from their source and can remain suspended in the air for an extended amount of time (Xie et al. Citation2007). Current literature suggests an upper size limit of 5–100 µm in diameter for aerosol particles (Bourouiba Citation2021; Innes et al. Citation2021; Lancet Respiratory Medicine Citation2020; Randall et al. Citation2021; Sills et al. Citation2020; Tang et al. Citation2021).
Both droplets and aerosol particles are known to carry bacteria (Innes et al. Citation2021; Meethil et al. Citation2021; Zemouri et al. Citation2017). Disease transmission via droplets can be managed by employing adequate personal protective equipment (PPE) and regular cleaning and disinfection. Unlike droplets, aerosol particles pose significant challenges for their control and removal due to their small size. A recent analysis of SARS-CoV-2 test data from asymptomatic individuals showed a mean of 2.1 × 107 virions per mL of saliva (Yang et al. Citation2021). Such a high concentration of viral particles, if aerosolised during dental AGPs, would pose a substantial risk for dentists and their personnel. In addition to being a disease vector, aerosol produced during AGPs may also contain heavy metals and other toxic particles (Ireland, Moreno, and Price Citation2003; Polednik Citation2014; Sotiriou et al. Citation2008). Their low mass allows them to remain airborne and drift with air currents unless removed through measures such as filtration. Moreover, their small size also enables them to penetrate surgical masks at a rate of up to 70% (Zangmeister et al. Citation2020). Other mask types and alternatives, such as cloth masks and gaiters, allows even more particles through (Lindsley et al. Citation2021; Morais et al. Citation2021). The larger particles that get through would deposit in the upper airways and pharynx, at a rate of up to 98% (Yeh and Schum Citation1980). Remaining smaller particles, which are not effectively filtered by the upper airways, would travel deeper into the respiratory system. For reference, over 90% of particles below 1 µm in diameter are expected to reach the lungs (Brown et al. Citation2013). Should any of these particles contain respiratory pathogens, they would pose a significant health hazard.
Surface contamination by droplets and bacteria from AGPs was the subject of numerous studies that used indirect measurement methods. Some counted bacteria colonies on exposed agar plates (Devker et al. Citation2012; Narayana et al. Citation2016), while others counted stains deposited on reactive or absorbent papers (Han et al. Citation2021; Veena et al. Citation2015). Although these methods are useful for assessing surface contamination from droplets and bacteria, they do not make direct measurements of aerosol particles in the respirable size range, which, as mentioned above, comprises of particles with diameters below 10 µm. Another method involves the use of air samplers, which traps airborne particles of a specific size range for bulk analysis (Allison et al. Citation2021b; Monarca et al. Citation2000). While this method can detect tracers or pathogens directly in particles with diameters well below 10 µm, it cannot provide a particle count, nor a size distribution of the particles. To that end, optical particle counting is a more suitable method since it can produce real-time data on the concentration and size distribution of aerosol particles over the size range of interest. This data would be complementary to current knowledge about surface contamination, as aerosol particles are not adequately addressed by typical surface cleaning procedures.
In response to the COVID-19 pandemic, dental regulators banned AGPs in the dental clinic until the introduction of new operating guidelines that reduced the risk of disease transmission. These new guidelines include mandatory use of aerosol reduction measures such as high-volume evacuators (HVEs) and air purifiers if an AGP is performed (CDA Citation2020; ODQ Citation2021; RCDSO Citation2021).
Air purifiers present a particularly compelling solution to the aerosol problem. Off-the-shelf air purifiers are affordable compared to revising building ventilation systems and are often equipped with high-efficiency particulate air (HEPA) filters that can remove at least 99.7% of 0.3 µm particles at high flowrates (ASME Citation2019; ISO Citation2017). In theory, this promises rapid cleansing of the air in the operating area, which could reduce fallow time requirements and provide protection during the procedure itself (Raghava and Vidovic Citation2021). However, there is a paucity of quantitative data in for commercial air purifiers (Geisinger and Iaonnidou Citation2021). A similar gap exists for micrometric aerosol present during dental procedures.
This study aims to evaluate the effectiveness of air purifiers and HVEs for aerosol reduction and to characterize the aerosol generated by dental drilling and scaling procedures using optical particle counting. We hypothesize that current aerosol reduction measures can meaningfully remove aerosol produced by AGPs.
Materials and methods
Experimental setup
The experiments were carried out in a single-patient dental clinic room (3.5 × 3.0 × 2.85 m L × W × H, volume: 30 m3) equipped with a standard dental chair (A-dec 500 Dental Chair, A-dec 332 Delivery System, A-dec, Oregon, USA) and an air purifier equipped with a HEPA filter (LV-H113, Levoit, USA, ). The air purifier has a clean air delivery rate of 274 CFM, which translates to over 15 air changes per hour (ACH) for the room, beyond the 12 ACH required by local guidance.
Figure 1. (a) High-speed handpiece [HS]. (b) Piezoelectric ultrasonic scaler [PUS]. (c) High-speed handpiece with plastic high-volume evacuator [HS + HVE(P)]. (d) High-speed handpiece with metal high-volume evacuator [HS + HVE(M)]. (e) Four sampling positions: (1) Dentist, (2) Assistant, (3) 1 m, (4) 2 m. Notice air purifier placement to the right of patient’s feet. (f) Air purifier [AP]. (g) Particle counter.
![Figure 1. (a) High-speed handpiece [HS]. (b) Piezoelectric ultrasonic scaler [PUS]. (c) High-speed handpiece with plastic high-volume evacuator [HS + HVE(P)]. (d) High-speed handpiece with metal high-volume evacuator [HS + HVE(M)]. (e) Four sampling positions: (1) Dentist, (2) Assistant, (3) 1 m, (4) 2 m. Notice air purifier placement to the right of patient’s feet. (f) Air purifier [AP]. (g) Particle counter.](/cms/asset/1d3a4794-493b-4e16-a8ea-470bcc5f7168/uast_a_2040729_f0001_c.jpg)
The dental instruments used were a high-speed handpiece (MASTERtorque™ Mini M8700 L, KaVo Dental, Germany, ) and an ultrasonic scaler (Cleanse S+, Dmetec, South Korea, ). Both were operated at maximum intensity and water dispensing rate, which corresponds to 5.68 L/hr and 1.32 L/hr, respectively. A molded-plastic HVE nozzle (TRK-O-VAC™, Plasdent, USA, ) and a metal HVE nozzle (Tip-A-Dilly®, Pelton & Crane, USA, ), which represent the most common designs in dental practice, were tested. The plastic HVE features a built-in tongue depressor and a flared opening, while the metal HVE terminates with a straw. The minimum internal diameter is 8.5 mm for the former, measured at the connecting end, and 6.0 mm for the latter, measured at the opening. They were both connected to the same vacuum source, which removes air and aerosols at 12 CFM. A saliva suction unit, included with the dental chair, was used to continuously remove water in the oral cavity.
To control variables that might impact aerosol level during measurement, the central air circulation system was stopped, the doors were closed, and entry and exit were restricted during trials. All procedures were performed by the same licensed dentist and assistant on a dental mannequin, which negates risks of patient-to-dentist infection. The dentist and the assistant were present during the entire procedure for every trial, including the controls, and wore protective gowns, surgical masks, and hairnets to control the aerosol they released from their breathing and their clothing.
Study design
To assess the aerosol risk posed by drilling and scaling, aerosol concentrations were measured during 15-min mock drilling or scaling procedure. The mock drilling emulated a crown preparation procedure at the right lower first molar using a high-speed handpiece () and the mock scaling was performed around the cervical area of each teeth using a piezoelectric scaler (). A control with no running AGP, but with the dentist and assistant’s presence, was measured for reference.
To evaluate the effectiveness of aerosol reduction measures recommended by the Ordre des dentistes du Québec (ODQ Citation2021), four additional intervention groups were measured. The plastic and metal HVEs (), and the air purifier (), were individually tested with the aforementioned mock drilling procedure as the aerosol source. The combined use of the plastic HVE and the air purifier was also evaluated. Lastly, a control with the air purifier running was also measured.
Experimental procedures
A particle counter (Optical Particle Sizer 3330, TSI Incorporated, USA, ) was used to measure aerosol concentration. The particle counter continuously intakes air at 1.0 L/min and then counts particles in the air, according to their size, into channels that span from 0.3 to 10.0 µm at an adjustable sampling rate and duration (TSI Incorporated Citation2013). The particle counter is equipped with a 1.3-meter long 6.35 mm ID antistatic silicone rubber tubing that ensures minimal particle loss in the measured particle size range. Each measurement lasted 20 s, consisting of a 10-s sampling period preceded by a 10-s wash-out period. This corresponds to 3 measurements per minute. The wash-out period helped prevent contamination of each new sample by previously sampled air.
Four clinically relevant positions were selected for evaluation (): (1) the dentist’s nose level, at 11 o’clock; (2) the assistant’s nose level, at 2 o’clock; (3) one meter in front of the patient’s head; and (4) two meters in front of the patient’s head. For each measurement, the unconnected end of the antistatic tubing was moved to the measurement position while the particle counter stayed in a central position,
Each group consisted of measurements taken in 6 sessions. In each session, the baseline aerosol concentration was measured prior intervention start at positions 2, 3, 4. The particle counter was then kept at position 1 to record the aerosol concentration throughout the intervention for 15 min. Aerosol concentrations were subsequently collected again at positions 2, 3, 4 for a post-intervention reading. Between measurement sessions, the room air was filtered and recirculated by running the air purifier at maximum fan speed until the aerosol concentration, measured with the particle counter, had stabilized to a baseline level.
Data processing
Aerosol concentration data were aggregated and plotted using Excel (Microsoft Corporation, USA). Outliers due to transmission of ultrasonic vibrations to the device were excluded from analysis. Descriptive analysis was performed in Excel (Microsoft Corporation, USA) and GraphPad Prism 9 (GraphPad Software, USA). Inability to assume normal distribution for the data and the limited sample size precludes the use of inferential statistical analyses. Aerosol concentration and calculated rates were continuous variables and were presented as median and inter-quartile range (IQR).
Aerosol concentration values were the sum of the particle count from each of the instrument’s size bins. The aerosol concentration change was calculated by subtracting the aerosol concentration at 0 min from the aerosol concentration at 15 min. This value is the focus of cross-group comparisons because it is free of the influence that a variable baseline aerosol concentration could impose on the results.
The increase ratio ( was calculated by dividing the aerosol concentration at 15 min (
) and at 0 min (
).
The reduction rate () of aerosol reduction measures was calculated as the difference between the median aerosol concentration change after operating the high-speed handpiece for 15 min (
) and the aerosol concentration change after repeating the same procedure while employing an aerosol reduction measure (
) normalized by the first quantity. Values are reported in percentages.
Results
High-speed handpiece use causes a significant aerosol concentration increase
The aerosol concentration increase in the high-speed handpiece group [HS], 181.5 (161.6–229.2) particles/cm3, was much greater compared to that of the control group [CTL], 5.4 (4.0–7.7) particles/cm3 (, Table S1). The 40-fold increase in aerosol concentration makes the HS group stand out against all the other groups ( and , Table S1). By contrast, the piezoelectric ultrasonic scaler group [PUS] only showed an increase of 4.4 (3.2–5.3) particles/cm3, which was slightly less than the CTL group (, Table S1). Only two peaks of up to 36 particles/cm3 occurred in one session of the PUS group. Therefore, mock drilling using the high-speed handpiece was selected as the aerosol source for the aerosol reduction measure tests.
Figure 2. Median dynamic aerosol concentrations measured at the dentist’s position: (a) high-speed handpiece [HS], (b) piezoelectric ultrasonic scaler [PUS], (c) control [CTL], (d, e) high-speed handpiece with high-volume evacuator (plastic/metal) [HS + HVE(P/M)], (f) high-speed handpiece with air purifier [HS + AP], (g) high-speed handpiece with plastic high-volume evacuator and air purifier [HS + HVE(P)+AP], (h) air purifier only [AP]. Color band represents the interquartile range. Median (IQR) increase ratio () and reduction rate (
).
![Figure 2. Median dynamic aerosol concentrations measured at the dentist’s position: (a) high-speed handpiece [HS], (b) piezoelectric ultrasonic scaler [PUS], (c) control [CTL], (d, e) high-speed handpiece with high-volume evacuator (plastic/metal) [HS + HVE(P/M)], (f) high-speed handpiece with air purifier [HS + AP], (g) high-speed handpiece with plastic high-volume evacuator and air purifier [HS + HVE(P)+AP], (h) air purifier only [AP]. Color band represents the interquartile range. Median (IQR) increase ratio (RI˜) and reduction rate (RR˜).](/cms/asset/aa86dfd3-f141-41f8-8260-a696fdcf244e/uast_a_2040729_f0002_c.jpg)
Aerosol reduction measures effectively reduced aerosol accumulation from drilling procedures
When used during drilling procedures, the plastic and metal HVEs [HS + HVE(P/M)] yielded aerosol reduction rates of 94.8 (94.3–96.0)% and 97.6 (95.6–99.1)%, respectively. The aerosol concentration change observed in those groups, 9.8 (7.4–9.9) and 6.1 (2.0–7.7) particles/cm3, were slightly higher than the CTL group (, Table S1). Similarly, the air purifier group [HS + AP] yielded an aerosol reduction rate of 94.8 (93.8–95.1)%, with a median similar to the [HS + HVE(P)] group (). Momentary peaks in aerosol concentration of less than 30 particles/cm3, were occasionally observed in these three groups (Figure S1).
Simultaneous use of the plastic HVE and the air purifier during drilling [HS + HVE(P)+AP] removed aerosol at a rate of 99.6 (99.4–99.8)% (, Table S1). The evolution of aerosol concentration in the group was uniform with no excursions. Aerosol concentration increased only by 0.8 (0.4–1.3) particles/cm3, which was slightly above the −0.2 (−0.6–0.2) particles/cm3 change in the air purifier group [CTL + AP] () but much less than the 5.4 (4.0–7.7) particles/cm3 change of the CTL group (, Table S1).
Other observations
Aerosol concentration increase was similar across the 4 tested positions in all groups except for the HS group and the HS + AP group where a relatively slight decrease was observed for positions progressively further away from the patient (, Figures S2 and S3, Table S1). This decrease is however minor relative to the differences between groups. As such, unless specified, quoted values were given at the dentist’s position for simplicity; the observations can be generalized to all four measurement positions.
Across all groups and positions, over 94.9% of sampled particles were smaller than 1.0 µm. Especially for the HS and PUS groups, more than 98.0% of sampled particles are smaller than 1.0 µm (, Table S2). Aerosol removal efficacy appears to decrease for smaller particles ().
Discussion
This study found that aerosol concentrations during drilling procedures could be reduced by 99.6% with the combination of an air purifier and an HVE. These findings, obtained through direct aerosol concentration measurements using optical particle counting, support the aerosol reduction measures advocated by dental regulatory bodies (CDA Citation2020; ODQ Citation2021; RCDSO Citation2021). The results demonstrated that correctly used aerosol reduction measures can be greatly reduce the aerosol exposure of dentists, assistants, and patients.
Effectiveness of the air purifier
The air purifier provided a reduction rate of 94.8 (93.8–95.1)% for aerosol produced during the drilling procedure. The combination of a HVE and an air purifier reached an aerosol reduction rate of 99.6 (99.4–99.8)%. A few studies have concluded that air purifiers are effective as a tool for localized infection control in residential and hospital settings (Boswell and Fox Citation2006; Mousavi et al. Citation2020; Qian et al. Citation2010; Zuraimi, Nilsson, and Magee Citation2011). Recent studies also reports their effectiveness in a school setting (Curtius, Granzin, and Schrod Citation2021) and a dental setting (Ren et al. Citation2021).The present results support a similar conclusion.
Current dental regulatory guidelines prescribe 6 air changes per hour (ACH) for most clinics, with one third from outside air, and 12 ACH if gas anesthesia is involved (ODQ Citation2021). Air purifiers should be selected according to room dimensions to meet or exceed ACH requirements. It should be noted that HEPA filtering is not a substitute for ventilation with outside air and does not remove volatile compounds and gaseous contaminants. Care must also be taken to orient the flow so that aerosol is not kicked up and allowed to remain in the room. In the present case, the air purifier was placed away from the patient and the airflow was unimpeded upwards. The ceiling deflected this airflow down along the walls toward the floor, producing a slight downdraft where the dentist and assistant were positioned.
Effectiveness of high-volume evacuators
The use of plastic or metal HVEs can reduce the aerosol generated by high-speed handpieces with a median reduction rate of 94.8 (94.3–96.0)% and 97.6 (95.6–99.1)%, respectively. These results show that the two tested HVE designs, which are very common in dental practices, perform similarly. This was expected since both were connected to the same vacuum source, which provides a flowrate of 12 CFM. These results are also similar to that of the air purifier used alone, which cleans air at 274 CFM. The similar aerosol removal performance despite the large difference in flowrate highlights the importance of capturing aerosols at the source. This proximity effect is also shown in the evaluation of other HVE designs that are placed outside of the mouth. Comparable aerosol removal performance was achieved with flow rates of 131–182 CFM using extraoral extraction devices placed from 15 to 40 cm away from the patient’s mouth (Allison et al. Citation2021a; Ehtezazi et al. Citation2021; Shahdad et al. Citation2020).
The measured reduction mirrors previous studies that demonstrated, using particle deposition methods, a reduction in surface contamination of the surroundings using HVEs (Devker et al. Citation2012; Meethil et al. Citation2021; Nagraj et al. Citation2020; Narayana et al. Citation2016). Studies employing particle counters also found similar results, noting that HVEs reduced aerosol concentration by up to 89.4% (Matys and Grzech-Leśniak Citation2020). However, the particle source for most studies about aerosols was the ultrasonic scaler, which produces mostly droplets and low amounts of aerosol. The present study improves upon previous findings by using the high-speed handpiece, a significantly greater source of aerosol. The study also finds HVEs an effective aerosol reduction measure.
Dental aerosol generating procedures
Only the high-speed handpiece greatly increased aerosol concentration compared to the control group. By contrast, the piezoelectric ultrasonic scaler did not cause an aerosol concentration increase that is outside of the range observed in the control group. These results are consistent with previous publications that used a particle counting device measuring aerosol generated from ultrasonic scalers and/or high-speed handpieces in a standard dental clinic room (Allison et al. Citation2021b; Matys and Grzech-Leśniak Citation2020; Polednik Citation2014, Citation2021; Sotiriou et al. Citation2008). The high aerosol production by the high-speed handpiece is also corroborated by air sampling of aerosolized tracer (Allison et al. Citation2021b).
The current consensus is that scaling procedures pose a more significant threat of contamination than drilling procedures (Harrel and Molinari Citation2004). Our findings, that the aerosol accumulation from scalers is not greater than the control group and less than the drilling procedure, seem to be contradictory. This is likely due to differences in the experimental method. Frequently cited studies mostly used particle deposition methods based on exposed agar plates or absorbent papers (Al-Amad et al. Citation2017; Devker et al. Citation2012; Innes et al. Citation2021; Narayana et al. Citation2016; Timmerman et al. Citation2004; Veena et al. Citation2015; Zemouri et al. Citation2017). These measurement methods cannot differentiate whether the contamination is from droplets or aerosol particles. The reported visible surface contamination could mostly be attributed to droplets, against which PPE offers adequate protection. In contrast, measurements in this study target specifically particles in the range of 0.3 to 10 µm, which are invisible and can float in the air for hours before depositing on surfaces.
Our results showed that over 94.9% of measured aerosol particles were less than 1 µm and could thus penetrate surgical masks at a rate above 50% (Brown et al. Citation2013; Zangmeister et al. Citation2020). Given that ultrasonic scalers produce measurably less aerosol particles, it could be argued that hygienists, in a typical clinical setup and wearing adequate PPE, would experience relative safety compared to dentists using high-speed handpieces since they would be exposed to less aerosols. However, in terms of exposure to contaminated droplets and aerosols, the ultrasonic scaler may pose a greater risk given previous studies that showed the latter produces more contaminated splatter (Innes et al. Citation2021). Furthermore, evidence using air sampling with tracers suggests that most of the aerosol produced by the high-speed handpiece is from the coolant fluid and would have very little saliva content. Estimates based on the salivary viral load for SARS-CoV-2 gives a viral content of roughly 4.0 copies/cm2 (Holliday et al. Citation2021). Considering N95 mask use and a threshold for an infective dose that is likely in the low hundreds of viral copies (Karimzadeh, Bhopal, and Nguyen Tien Citation2021), the risk from contaminated aerosol would appear to be low. Additionally, rubber dams can further reduce aerosol contamination for high-speed handpieces (Vernon et al. Citation2021). As such, it is important to consider evidence regarding both droplets and aerosol to assess contamination risks faced by the medical personnel in a dental practice.
Instrument limitations
While an optical particle counter is highly sensitive to the presence of particles within its range of measurement, it can only measure the particle size and cannot distinguish between different sources of aerosol. The particle counter also cannot assess the particle mass nor surface area, which are characteristics that greatly impact the particle’s aerodynamic properties and its ability to carry pathogens. There are a number of contributing factors to an increase in aerosol concentration, such as the operators, the air pollution, dust, etc. Since the particle counter cannot discriminate particles by source, it becomes imperative to eliminate and isolate aerosol sources external to the experiment. Hence, strategies such as the closing of doors, the stoppage of the ventilation system, the usage of PPE by operators, and the air renewal between each measurement, were used in the present study.
Biological tracers could have been used to simulate contaminants and track aerosol spread. However, the measured reduction in aerosol concentration is likely to correlate proportionally to a reduction in contaminated aerosol floating in the air. A recent study that assessed biological content in dental AGPs aerosols suggests that the presence of aerosolized microorganisms can be effectively reduced by HVE, and that of salivary microbial loads, by mouth rinses (Meethil et al. Citation2021). The present study did not assess any droplet deposition as it was extensively studied in other publications (Innes et al. Citation2021).
Contaminants in aerosol could affect their geometry and drying characteristics, and thus their aerodynamic behavior over time. The instruments used in this study can only detect particles with a diameter as small as 0.3 µm. The virus motivating this study, SARS-CoV-2, ranges from 60 to 140 nm in diameter (Zhu et al. Citation2020). While its presence was detected in particles as only small as 250 nm (Liu et al. Citation2020), it remains possible that particles with diameters as small as 60 nm could potentially transmit the disease. Additionally, environmental factors affect the infectivity of viral particles in the aerosol (Dabisch et al. Citation2021), which should be taken into consideration when assessing infection risks.
Methodological limitations
Many factors may affect the aerosol concentration, such as the room size, the instrument settings for the AGPs, the atmospheric conditions inside and outside of the clinic (humidity, temperature), the presence of healthcare workers and the patient, and the room furnishing. No patients were involved in this study due to regulatory constraints from COVID-19. However, a patient’s breathing could also add aerosol to the room. The interaction between central ventilation systems and air purifiers may also impact aerosol removal. These factors should be considered when making comparisons or attempting to replicate the present study.
The present study simulated worst-case conditions, with no air circulation in the room and maximum waterflow in the instruments. This measure of shutting off the central ventilation system for the entire clinic was taken to account for the variations in mechanical ventilation performance that exist between different clinics and sometimes between different rooms in the same clinic. The reasoning is that any kind of mechanical ventilation should have a better aerosol removal performance than a closed room with no ventilation. Hence, by measuring the worst case performance, our results would establish an upper limit for the aerosol concentration expected from the tested interventions in a room of similar dimensions. This similar reasoning prompted the use of maximum intensity and waterflow in the dental instruments.
In the control group, the aerosol concentration increased after 15 min. There are two possible sources contributing to this increase, one is the presence of the dentist and assistant, which is necessary to the experiment, and the other is the ventilation system, which could have allowed the gradual diffusion of the aerosols from the outside of the room despite being stopped. The latter could be mitigated in future studies by temporarily sealing any vents the lead into to test location.
Conclusion
Aerosol reduction measures can effectively remove the aerosol generated by drilling procedures. Air purifiers and high-volume extractors used individually reduced aerosol concentration at a rate of 94.8% to 97.6%. Using both measures simultaneously brought the reduction rate to 99.6%. Following current regulatory guidelines can ensure a low risk of inhaling contaminated aerosol for dentists, assistants, and patients.
Authors’ contributions
Z. He and Q. Gao contributed to the conception, study design, data acquisition and interpretation, drafted and critically revised the manuscript. A. Henley contributed to the study design and data acquisition. Z. D. Der Khatchadourian, W. Somerville, F. Tamimi and L. Mongeau contributed to conception and study design, and critically revised the manuscript. Z. D. Der Khatchadourian and M. Wiseman provided test facilities and guidance in the experimental procedure. All authors gave their final approval and agree to be accountable for all aspects of the work.
Supplemental Material
Download MS Word (808.8 KB)Acknowledgments
Open Access funding provided by the Qatar National Library. We sincerely thank Loïc Wingert from the Institut de recherche Robert-Sauvé en santé et en sécurité du travail (IRSST) for their generous loan of the OPS 3330 and technical guidance. The suggestions, comments, and encouragements of Dr. Caroline Duchaine are gratefully acknowledged. The authors thank Dr. Caroline Duchaine for sharing the results with the Quebec dentist community and regulatory agencies. We sincerely thank Brendan Leslie Kelly, Dr. Didem Dagdeviren and Dr. Natalie Morin from the McGill University Faculty of Dentistry for their loan of the experimental equipment. We sincerely thank Katherine Hales and the McGill Seeds of Change team, Niousha Noushi, Andrée Lessard, Julie Brown, and David Leblanc for their help in raising funds for the project.
Disclosure statement
The authors declare no conflict of interest.
Additional information
Funding
References
- Al-Amad, S. H., M. A. Awad, F. M. Edher, K. Shahramian, and T. A. Omran. 2017. The effect of rubber dam on atmospheric bacterial aerosols during restorative dentistry. J. Infect. Public Health. 10 (2):195–200. doi:10.1016/j.jiph.2016.04.014.
- Allison, J. R., C. Dowson, K. Pickering, G. Červinskytė, J. Durham, N. S. Jakubovics, and R. Holliday. 2021a. Local exhaust ventilation to control dental aerosols and droplets. J. Dent. Res. doi:10.1177/00220345211056287.
- Allison, J. R., D. C. Edwards, C. Bowes, K. Pickering, C. Dowson, S. J. Stone, J. Lumb, J. Durham, N. Jakubovics, and R. Holliday. 2021b. The effect of high-speed dental handpiece coolant delivery and design on aerosol and droplet production. J. Dent. 112. doi:10.1016/j.jdent.2021.103746.
- ASME. 2019. AG-1: Code on nuclear air and gas treatment. In FC: HEPA filters. New York, NY: The American Society of Mechanical Engineers.
- Boswell, T. C., and P. C. Fox. 2006. Reduction in MRSA environmental contamination with a portable HEPA-filtration unit. J. Hosp. Infect. 63 (1):47–54. doi:10.1016/j.jhin.2005.11.011.
- Bourouiba, L. 2021. The fluid dynamics of disease transmission. Annu. Rev. Fluid Mech. 53 (1):473–508. doi:10.1146/annurev-fluid-060220-113712.
- Brown, J. S., T. Gordon, O. Price, and B. Asgharian. 2013. Thoracic and respirable particle definitions for human health risk assessment. Part Fibre Toxicol. 10:12. doi:10.1186/1743-8977-10-12.
- CDA. 2020. Return-to-practice office manual. Ottawa: Canadian Dental Association.
- Curtius, J., M. Granzin, and J. Schrod. 2021. Testing mobile air purifiers in a school classroom: Reducing the airborne transmission risk for sars-cov-2. Aerosol Sci. Technol. 55 (5):586–99. doi:10.1080/02786826.2021.1877257.
- Dabisch, P., M. Schuit, A. Herzog, K. Beck, S. Wood, M. Krause, D. Miller, W. Weaver, D. Freeburger, I. Hooper, et al. 2021. The influence of temperature, humidity, and simulated sunlight on the infectivity of sars-cov-2 in aerosols. Aerosol Sci. Technol. 55 (2):142–53. doi:10.1080/02786826.2020.1829536.
- Devker, N. R., J. Mohitey, A. Vibhute, V. S. Chouhan, P. Chavan, S. Malagi, and R. Joseph. 2012. A study to evaluate and compare the efficacy of preprocedural mouthrinsing and high volume evacuator attachment alone and in combination in reducing the amount of viable aerosols produced during ultrasonic scaling procedure. J. Contemp. Dent. Pract. 13 (5):681–9. doi:10.5005/jp-journals-10024-1209.
- Ehtezazi, T., D. G. Evans, I. D. Jenkinson, P. A. Evans, V. J. Vadgama, J. Vadgama, F. Jarad, N. Grey, and R. P. Chilcott. 2021. Sars-cov-2: Characterisation and mitigation of risks associated with aerosol generating procedures in dental practices. Br. Dent. J. doi:10.1038/s41415-020-2504-8.
- Geisinger, M. L., and E. Iaonnidou. 2021. Up in the air? Future research strategies to assess aerosols in dentistry. JDR Clin. Trans. Res. 6 (2):128–31. doi:10.1177/2380084420982506.
- Han, P., H. Li, L. J. Walsh, and S. Ivanovski. 2021. Splatters and aerosols contamination in dental aerosol generating procedures. Appl. Sci. 11 (4):1914. doi:10.3390/app1104.
- Harrel, S. K., and J. Molinari. 2004. Aerosols and splatter in dentistry: A brief review of the literature and infection control implications. J. Am. Dent. Assoc. 135 (4):429–37. doi:10.14219/jada.archive.2004.0207.
- Holliday, R., J. R. Allison, C. C. Currie, D. C. Edwards, C. Bowes, K. Pickering, S. Reay, J. Durham, J. Lumb, N. Rostami, et al. 2021. Evaluating contaminated dental aerosol and splatter in an open plan clinic environment: Implications for the covid-19 pandemic. J. Dent. 105:103565. doi:10.1016/j.jdent.2020.103565.
- Innes, N., I. G. Johnson, W. Al-Yaseen, R. Harris, R. Jones, S. Kc, S. McGregor, M. Robertson, W. G. Wade, and J. E. Gallagher. 2021. A systematic review of droplet and aerosol generation in dentistry. J. Dent. 105:103556. doi:10.1016/j.jdent.2020.103556.
- Ireland, A. J., T. Moreno, and R. Price. 2003. Airborne particles produced during enamel cleanup after removal of orthodontic appliances. Am. J. Orthod. Dentofacial Orthop. 124 (6):683–6. doi:10.1016/S0889-5406(03)00623-1.
- ISO. 2017. ISO 29463: High efficiency filters and filter media for removing particles from air. In Part 1: Classification, performance, testing and marking. Switzerland: International Standards Organization.
- Karimzadeh, S., R. Bhopal, and H. Nguyen Tien. 2021. Review of infective dose, routes of transmission and outcome of covid-19 caused by the sars-cov-2: Comparison with other respiratory viruses. Epidemiol. Infect. 149:e96. doi:10.1017/S0950268821000790.
- Lancet Respiratory Medicine. 2020. Covid-19 transmission—up in the air. Lancet Respir. Med. 8 (12):1159. doi:10.1016/S2213-2600(20)30514-2.
- Lindsley, W. G., F. M. Blachere, B. F. Law, D. H. Beezhold, and J. D. Noti. 2021. Efficacy of face masks, neck gaiters and face shields for reducing the expulsion of simulated cough-generated aerosols. Aerosol Sci. Technol. 55 (4):449–57. doi:10.1080/02786826.2020.1862409.
- Liu, Y., Z. Ning, Y. Chen, M. Guo, Y. Liu, N. K. Gali, L. Sun, Y. Duan, J. Cai, D. Westerdahl, et al. 2020. Aerodynamic analysis of sars-cov-2 in two Wuhan hospitals. Nature 582 (7813):557–60. doi:10.1038/s41586-020-2271-3.
- Matys, J., and K. Grzech-Leśniak. 2020. Dental aerosol as a hazard risk for dental workers. Materials 13 (22):5109. doi:10.3390/ma13225109.
- Meethil, A. P., S. Saraswat, P. P. Chaudhary, S. M. Dabdoub, and P. S. Kumar. 2021. Sources of sars-cov-2 and other microorganisms in dental aerosols. J. Dent. Res. 100 (8):817–23. doi:10.1177/00220345211015948.
- Micik, R. E., R. L. Miller, M. A. Mazzarella, and G. Ryge. 1969. Studies on dental aerobiology. I. Bacterial aerosols generated during dental procedures. J. Dent. Res. 48 (1):49–56. doi:10.1177/00220345690480012401.
- Monarca, S., M. Grottolo, D. Renzi, C. Paganelli, P. Sapelli, I. Zerbini, and G. Nardi. 2000. Evaluation of environmental bacterial contamination and procedures to control cross infection in a sample of Italian dental surgeries. Occup. Environ. Med. 57 (11):721–6. doi:10.1136/Foem.57.11.721.
- Morais, F. G., V. K. Sakano, L. N. d. Lima, M. A. Franco, D. C. Reis, L. M. Zanchetta, F. Jorge, E. Landulfo, L. H. Catalani, H. M. J. Barbosa, et al. 2021. Filtration efficiency of a large set of covid-19 face masks commonly used in brazil. Aerosol Sci. Technol. 55 (9):1028–41. doi:10.1080/02786826.2021.1915466.
- Mousavi, E. S., K. J. Godri Pollitt, J. Sherman, and R. A. Martinello. 2020. Performance analysis of portable HEPA filters and temporary plastic anterooms on the spread of surrogate coronavirus. Build. Environ. 183:107186. doi:10.1016/j.buildenv.2020.107186.
- Nagraj, S. K., P. Eachempati, M. Paisi, M. Nasser, G. Sivaramakrishnan, and J. H. Verbeek. 2020. Interventions to reduce contaminated aerosols produced during dental procedures for preventing infectious diseases. Cochrane Database Syst Rev 10:CD013686. doi:10.1002/14651858.CD013686.pub2.
- Narayana, T., L. Mohanty, G. Sreenath, and P. Vidhyadhari. 2016. Role of preprocedural rinse and high volume evacuator in reducing bacterial contamination in bioaerosols. J. Oral Maxillofac. Pathol. 20 (1):59. doi:10.4103/0973-029X.180931.
- ODQ. 2021. Phase 4: Prestation des services buccodentatires en contexte de pandémie. Directives intermédiaires: Ministère de la Santé et des Services Sociaux (MSSS).
- Polednik, B. 2014. Aerosol and bioaerosol particles in a dental office. Environ. Res. 134:405–9. doi:10.1016/j.envres.2014.06.027.
- Polednik, B. 2021. Exposure of staff to aerosols and bioaerosols in a dental office. Build. Environ. 187:107388. doi:10.1016/j.buildenv.2020.107388.
- Qian, H., Y. Li, H. Sun, P. V. Nielsen, X. Huang, and X. Zheng. 2010. Particle removal efficiency of the portable HEPA air cleaner in a simulated hospital ward. Build. Simul. 3 (3):215–24. doi:10.1007/s12273-010-0005-4.
- Raghava, N., and B. Vidovic. 2021. Using computational fluid dynamics to evaluate the role of air purification in reducing fallow time in dentistry. Int. J. Dent. Oral Health 7 (3):1–7. doi:10.16966/2378-7090.362.
- Randall, K., E. T. Ewing, L. Marr, J. Jimenez, and L. Bourouiba. 2021. How did we get here: What are droplets and aerosols and how far do they go? A historical perspective on the transmission of respiratory infectious diseases. Interface Focus. 11 (6). doi:10.1098/rsfs.2021.0049.
- RCDSO. 2021. Covid-19: Managing infection risks during in-person dental care. Toronto, Ontario, Canada: Royal College of Dental Surgeons of Ontario.
- Ren, Y.-F., Q. Huang, T. Marzouk, R. Richard, K. Pembroke, P. Martone, T. Venner, H. Malmstrom, and E. Eliav. 2021. Effects of mechanical ventilation and portable air cleaner on aerosol removal from dental treatment rooms. J. Dent. 105:103576. doi:10.1016/j.jdent.2020.103576.
- Shahdad, S., T. Patel, A. Hindocha, N. Cagney, J.-D. Mueller, N. Seoudi, C. Morgan, and A. Din. 2020. The efficacy of an extraoral scavenging device on reduction of splatter contamination during dental aerosol generating procedures: An exploratory study. Br. Dent. J. doi:10.1038/s41415-020-2112-7.
- Sills, J., K. A. Prather, L. C. Marr, R. T. Schooley, M. A. McDiarmid, M. E. Wilson, and D. K. Milton. 2020. Airborne transmission of sars-cov-2. Science 370 (6514):303–4. doi:10.1126/science.abf0521.
- Sotiriou, M., S. F. Ferguson, M. Davey, J. M. Wolfson, P. Demokritou, J. Lawrence, S. N. Sax, and P. Koutrakis. 2008. Measurement of particle concentrations in a dental office. Environ. Monit. Assess. 137 (1–3):351–61. doi:10.1007/s10661-007-9770-7.
- Tang, J. W., W. P. Bahnfleth, P. M. Bluyssen, G. Buonanno, J. L. Jimenez, J. Kurnitski, Y. Li, S. Miller, C. Sekhar, L. Morawska, et al. 2021. Dismantling myths on the airborne transmission of severe acute respiratory syndrome coronavirus-2 (sars-cov-2). J. Hosp. Infect. 110:89–96. doi:10.1016/j.jhin.2020.12.022.
- Timmerman, M. F., L. Menso, J. Steinfort, A. J. Van Winkelhoff, and G. A. Van Der Weijden. 2004. Atmospheric contamination during ultrasonic scaling. J. Clin. Periodontol. 31 (6):458–62. doi:10.1111/j.1600-051X.2004.00511.x.
- TSI Incorporated. 2013. Model 3330 optical particle sizer spectrometer operation and service manual. Shoreview, MN: TSI Incorporated.
- Veena, H. R., S. Mahantesha, P. A. Joseph, S. R. Patil, and S. H. Patil. 2015. Dissemination of aerosol and splatter during ultrasonic scaling: A pilot study. J. Infect. Public Health 8 (3):260–5. doi:10.1016/j.jiph.2014.11.004.
- Vernon, J. J., E. V. I. Black, T. Dennis, D. A. Devine, L. Fletcher, D. J. Wood, and B. R. Nattress. 2021. Dental mitigation strategies to reduce aerosolization of sars-cov-2. J. Dent. Res. 100 (13):1461–7. doi:10.1177/00220345211032885.
- Xie, X., Y. Li, A. T. Chwang, P. L. Ho, and W. H. Seto. 2007. How far droplets can move in indoor environments-revisiting the Wells evaporation-falling curve. Indoor Air 17 (3):211–25. doi:10.1111/j.1600-0668.2007.00469.x.
- Yang, Q., T. K. Saldi, P. K. Gonzales, E. Lasda, C. J. Decker, K. L. Tat, M. R. Fink, C. R. Hager, J. C. Davis, C. D. Ozeroff, et al. 2021. Just 2% of sars-cov-2-positive individuals carry 90% of the virus circulating in communities. Proc. Natl. Acad. Sci. USA 118 (21):e2104547118. doi:10.1073/pnas.2104547118.
- Yeh, H.-C., and G. M. Schum. 1980. Models of human lung airways and their application to inhaled particle deposition. Bull. Math. Biol. 42 (3):461–80. doi:10.1007/BF02460796.
- Zangmeister, C. D., J. G. Radney, E. P. Vicenzi, and J. L. Weaver. 2020. Filtration efficiencies of nanoscale aerosol by cloth mask materials used to slow the spread of sars-cov-2. ACS Nano 14 (7):9188–200. doi:10.1021/acsnano.0c05025.
- Zemouri, C., H. de Soet, W. Crielaard, and A. Laheij. 2017. A scoping review on bio-aerosols in healthcare and the dental environment. PLOS One 12 (5):e0178007. doi:10.1371/journal.pone.0178007.
- Zhu, N., D. Zhang, W. Wang, X. Li, B. Yang, J. Song, X. Zhao, B. Huang, W. Shi, R. Lu, China Novel Coronavirus Investigating and Research Team, et al. 2020. A novel coronavirus from patients with pneumonia in china, 2019. N. Engl. J. Med. 382 (8):727–33. doi:10.1056/NEJMoa2001017.
- Zuraimi, M. S., G. J. Nilsson, and R. J. Magee. 2011. Removing indoor particles using portable air cleaners: Implications for residential infection transmission. Build. Environ. 46 (12):2512–9. doi:10.1016/j.buildenv.2011.06.008.