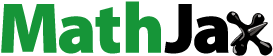
Abstract
The chemical components and mechanisms underlying the toxicity and adverse health effects of particulate matter (PM) in the atmosphere have not been fully elucidated. After designing a small, openable, stainless steel cyclone to collect PM samples effectively in powder form for use in toxicological experiments, we evaluated its performance. We compared it with a commercially available aluminum cyclone of similar dimensions, but which is unopenable. The aerodynamic cutoff diameter of the openable cyclone was found by experimentation to be approximately 0.2 µm at a flow rate of 90 L min−1, which is comparable to the unopenable commercial cyclone. The sampling yields, representing the fraction of obtained sample mass relative to the total mass of PM with aerodynamic diameter smaller than 2.5 μm (PM2.5) drawn into the sampler, were approximately 1.3 times higher, on average, for the openable cyclone than for the unopenable cyclone. The openable design of the cyclone might contribute to a marked increase in the finally obtained amounts of PM samples. Analyses of metal concentrations in the PM samples collected simultaneously using the stainless steel openable cyclone and aluminum unopenable cyclone suggest that the stainless steel cyclone is less likely than the aluminum cyclone to cause sample contamination from its material. The openable cyclone developed for this study facilitates the effective collection of powder-form PM samples suitable for use in toxicological experiments.
EDITOR:
1. Introduction
Particulate matter (PM) in the atmosphere is a complex mixture comprising various chemical components such as inorganic salts, metals, elemental carbon, organics, and microorganisms, originating from both anthropogenic and natural sources (Calvo et al. Citation2013). Numerous epidemiological studies have demonstrated that inhalation and exposure to PM with aerodynamic diameter smaller than 2.5 μm (PM2.5) adversely affect human health (e.g., Cohen et al. Citation2017; Lelieveld et al. Citation2015; Pope and Dockery Citation2006), leading particularly to cardiorespiratory diseases (e.g., Brook et al. Citation2010; Dockery and Pope Citation1994; Hoek et al. Citation2013). Nevertheless, the chemical components and mechanisms which trigger diseases associated with exposure to PM2.5 have not been fully elucidated to date.
Toxicological experiments investigating PM toxicity commonly use PM collected on filters, such as polytetrafluoroethylene (PTFE) membrane filters and quartz fiber filters. Extraction of the PM is a crucially important step in preparing PM samples collected on filters for exposure to cells or animals. For instance, organic and water-based solvents are used to extract PM samples from the filters, allowing for the study of the biological effects of the organic or aqueous fraction present in PM (e.g., Alessandria et al. Citation2014; Cavanagh et al. Citation2009; Heo et al. Citation2015). However, solid particles such as elemental carbon, metal oxides, and mineral dust particles are not recoverable using these methods. Complete transfer of the entire PM sample from the filter fibers into the solvents is quite difficult irrespective of the type of solvent or physical technique applied, such as vibration and sonication. As a result, the chemical compositions and bioactivities of the extracted PM fractions might differ considerably from those of the PM originally collected on the filters (Roper et al. Citation2019; Van Winkle et al. Citation2014). Additionally, to find the mass of the extracted PM and to exclude the effects of solvents from experiments, the extracted PM must be dried, recovered, and resuspended before exposure to cells or animals. Consequently, PM samples collected on filters might not be suitable for investigating the toxicity and health effects of actual atmospheric PM, including the synergetic effects of multiple components present in the PM.
To address the issues of the compositional artifacts resulting from extraction and the complex and laborious extraction procedures, an alternative method for PM sampling is to collect PM in powder form using a cyclone, rather than using filter-based sampling. Powder-form PM samples obviate the need for extraction. Okuda et al. (Citation2015, Citation2018) and Okuda and Isobe (Citation2017) developed a high-volume PM sampler equipped with a cyclone for toxicological experiments. Using the PM samples collected using this high-volume cyclone sampler, Honda et al. (Citation2021) compared the biological responses from exposure to crude cyclone-collected PM with those from exposure to the corresponding extracts. They found that exposure to crude cyclone-collected PM induces quite different biological responses compared to exposure to the corresponding extracts. Therefore, cyclone-collected powder-form PM samples might be suitable for experiments evaluating the toxicity and health effects of real atmospheric PM.
Cyclone samplers offer many benefits aside from the ability to collect powdery PM samples, such as simple structure, easy operation, and small pressure drop, thereby allowing for low-cost, long-term sampling. However, cyclones have been used only rarely for sampling PM2.5 because common cyclones have low collection efficiencies for PM2.5. The mass and size of PM collected using a cyclone depend on the cyclone’s design and its operating flow rate. Alimov et al. (Citation2022) conducted a comparison of chemical components and toxicity of PM2.5 collected using the high-volume cyclone sampler developed by Okuda et al. (Citation2015) (large cyclone with 68 mm inner diameter, HVS3; CS3 Inc., Sandpoint, ID) and a commercially available low-volume cyclone (small aluminum cyclone with 12 mm inner diameter; URG-2000-30EHB; URG Corp.). Their results suggest that PM samples collected using the commercially available small cyclone are more suitable for evaluating PM2.5 toxicity because the sizes of PM collected using the small cyclone were smaller than those collected using the high-volume cyclone sampler, which is equipped with the large cyclone. However, the small size of the cyclone makes it difficult to recover PM samples adhering to its interior. Although many studies have been conducted to improve the collection efficiency of cyclones for fine PM in the atmosphere (e.g., Azadi, Azadi, and Mohebbi Citation2010; Brar, Sharma, and Elsayed Citation2015; Fu et al. Citation2017; Nassaj, Toghraie, and Afrand Citation2019; Yoshida et al. Citation2012; Zhang et al. Citation2019; Zhang et al. Citation2021), few attempts have been made to improve the recovery efficiency for PM samples adhering to the inside of cyclone. Additionally, as reported by Alimov et al. (Citation2022), the PM samples collected using the metal cyclone might be contaminated by the cyclone’s material. To address these issues, we developed a small, openable stainless steel cyclone for this study and then evaluated its performance. Stainless steel is less likely than aluminum to be scraped. For that reason, sample contamination might be reduced by changing the cyclone material from aluminum to stainless steel.
2. Methods
2.1. Design of the openable cyclone
presents an illustration of the openable cyclone, which comprises upper, lower, and middle parts. The middle part was designed to open vertically for the easy and effective collection of PM adhering to the inner wall. All the parts of the openable cyclone were manufactured using stainless steel (ISO: X5CrNi18-10). The leakage test experiment is presented along with online supplementary information (SI; Text S1 and Figure S1). Air leakage from the cyclone was found to be negligible. The openable cyclone dimensions are presented in . These dimensions closely resemble those of a commercially available small cyclone (URG-2000-30EHB; URG Corp.) based on a Stairmand-type cyclone described and evaluated by Moore and McFarland (Citation1993). Hereinafter, we refer to this commercially available cyclone as the “URG cyclone.” The URG cyclone is made of aluminum coated with PTFE. It has an aerodynamic cutoff diameter of 1 μm at a flow rate of 16.7 L min−1, according to data provided by the manufacturer. For this study, we evaluated the openable cyclone in comparison to the URG cyclone.
2.2. Particle penetration efficiencies of the openable cyclone
To evaluate the separation performance of the openable cyclone, we found the penetration efficiencies of the openable cyclone at a flow rate of 90 L min−1 under a standard condition (1 atm, 25 °C). The flow rate of 90 L min−1 was chosen based on the performance constraints of the pump (DA-241S; ULVAC). The aerodynamic cutoff diameter at flow rates higher than 17 L min−1 is not provided by the manufacturer for the URG cyclone. The penetration efficiency (P) is defined by the function of the aerodynamic diameter (Dae), as shown below:
(1)
(1)
In this equation, Nd, and Nu, respectively, represent the particle number concentrations measured downstream and upstream of the cyclone. To obtain P(Dae), we measured Nd and Nu for aerosols consisting of quasi-monodispersed ammonium sulfate (AS) particles or fluorescent polystyrene latex (PSL) sphere particles (Polysciences Inc., Warrington, PA, USA). For comparison, we also used the same method to find P(Dae) for the URG cyclone.
presents the experiment setup used to measure Nd using AS aerosol. A polydispersed AS aerosol was generated by atomizing a 4% AS solution using an atomizer (Model 3079; TSI Inc., St. Paul, MN, USA) at a flow rate of 3 L min−1. Subsequently, the aerosol was dried at a relative humidity (RH) of less than 35% using a diffusion dryer. To generate quasi-monodispersed AS aerosol, polydispersed AS aerosol was introduced into an Am-241 neutralizer and a differential mobility analyzer (DMA; model DMA-5180; Sibata Scientific Technology Ltd., Tokyo, Japan). The DMA-classified AS aerosol was diluted with particle-free air to 90 L min−1 before being introduced into the cyclone. Using a condensation particle counter (CPC, model 3750; TSI Inc.), the Nd values were measured downstream of the cyclone for the quasi-monodisperse AS aerosols with electrical mobility diameters ranging from approximately 0.033 µm to 0.30 µm. An isokinetic sampling probe, as shown in Figure S2, was used downstream of the cyclone to introduce the AS aerosol into the CPC. Immediately after measuring the size-resolved Nd, we performed the same experiments but without the cyclone to measure Nu. The Nd and Nu measurements were repeated five times. It is noteworthy that the DMA-classified aerosol is not perfectly monodispersed because of the presence of multiply charged particles, which are larger than the singly charged particles with the same electrical mobility. These multiply charged particles can engender considerable underestimation of the penetration efficiencies. Therefore, assuming the equilibrium charge distribution, we corrected for the effects of the multiply charged particles on Nd and Nu values based on the number concentrations measured for the larger particle size channels.
Figure 3. Experiment setups used to examine the particle penetration efficiencies of the openable and URG cyclones using particles of two types: (a) AS particles and (b) fluorescent PSL particles. These setups were intended specifically for measuring Nd. To measure Nu, the cyclone was excluded from the setups. Details of the isokinetic sampling probe are presented in online supplementary information (Figure S2).
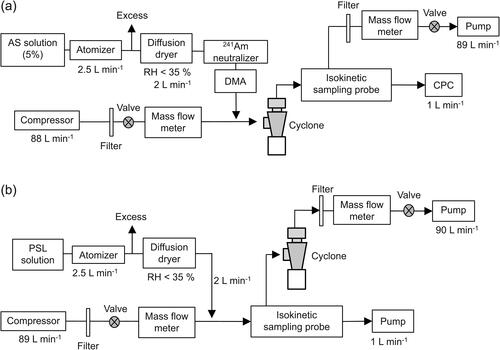
In accordance with the method reported by Alimov et al. (Citation2022), we measured Nd and Nu using monodispersed fluorescent PSL particles with geometric diameters (±1σ) of 0.210 (±0.008) µm, 0.47 (±0.01) µm, and 1.03 (±0.02) µm, according to the manufacturer’s instructions. presents an illustration of the experiment setup used to measure Nd using the fluorescent PSL particles. The PSL aerosol was generated using the same atomizer used for generating the AS aerosol and was subsequently dried to RH of less than 35%. The PSL aerosol was diluted with particle-free air to 91 L min−1. After passage through an isokinetic sampling probe (Figure S2), the PSL aerosol was introduced into the cyclone at a flow rate of 90 L min−1. To measure Nd, the PSL particles were collected downstream of the cyclone on a quartz fiber filter (Ф90 mm, QR-100; Advantec Co. Ltd.). They were then dissolved by shaking the filter in 10 mL of ethyl acetate for 30 min. Then, the ethyl acetate solution was filtered using a cellulose acetate membrane filter (0.20 µm pore size, 25CS020AN; Advantec Co. Ltd.) to remove quartz fibers. The fluorescence intensity of the ethyl acetate solution is proportional to the number of the fluorescent PSL particles collected on the filter. Therefore, Nd can be estimated by measuring the fluorescence intensity of this solution. The fluorescence intensity of the ethyl acetate solution was measured at a 490 nm wavelength using a spectrofluorometer (RF-6000; Shimadzu Corp., Kyoto, Japan). Immediately after measuring Nd, we conducted the same experiments without the cyclone to measure Nu.
The electrical mobility diameters of the AS particles and the geometric diameters of the fluorescent PSL particles were converted to aerodynamic diameters (Dae) by solving the following equation:
(2)
(2)
The equation is derived from Stokes’ law applying slip correction (Hinds Citation1982). In this equation, Dg represents the geometric diameter, ρ denotes the density of AS or PSL particles (1.77 g cm−3 for AS, and 1.05 g cm−3 for PSL), Cg is the Cunningham correction factor for particles with diameter Dg, ρ0 stands for the unit density (1 g cm−3), and Cae is the Cunningham correction factor for particles with diameter Dae. The electrical mobility diameter of the spherical AS particles can be assumed to be equal to Dg. The Cunningham correction factor (C) for particles with diameter D is given as
(3)
(3)
here, λ stands for the mean free path of air molecules (67.3 nm). Also, α, β, and γ are the experimentally determined coefficients. For this study, the values of α, β, and γ were assigned, respectively, as 1.165, 0.483, and 0.997 according to values reported by Kim et al. (Citation2005).
To ascertain the cutoff diameter of the cyclones, the experimentally obtained P values were fitted using the logistic equation presented below (Iozia and Leith Citation1990).
(4)
(4)
In that equation, Dae50 represents the aerodynamic cutoff diameter; k denotes the slope of the curve. Fitting was performed using nonlinear least squares method with the Gauss–Newton algorithm (implemented using the nls function in R). The fitted curve was also used to calculate the geometric standard deviation (GSD) using the following equation:
(5)
(5)
In this equation, Dae16 and Dae84, respectively, represent the aerodynamic diameters corresponding to 16% and 84% penetration efficiency. The GSD value provides information about the sharpness of the particle separation.
2.3. Atmospheric PM sampling and chemical analysis
2.3.1. Atmospheric PM sampling
To evaluate the sampling performance of the openable cyclone and its ability to collect representative atmospheric PM samples, we conducted continuous atmospheric PM sampling at Yokohama, Japan (Keio University, Yagami Campus, 35.56°N, 139.66°E) during the summer (30 June–6 September 2021), autumn (8 September–25 November 2021), and winter (29 November 2021–8 February 2022) seasons. Simultaneously, we collected PM samples using the openable cyclone, URG cyclone, and filters. The sampling site was located in an urban area approximately 15 km southwest of the center of Tokyo, as described in reports of earlier studies (Honda et al. Citation2021; Kurihara et al. Citation2022). portrays a schematic diagram and an illustration of a PM sampling device called a Keio-Transportable Real impactor with Cyclone (K-TRiC), which incorporates the openable cyclone or URG cyclone. The flow rate of the K-TRiC was set as 90 L min−1. To collect particles smaller than 2.5 μm diameter using the cyclone, an impactor was used upstream of the cyclone to remove coarse particles with aerodynamic diameter larger than approximately 2.5 μm. The impactor design resembles that developed by Okuda et al. (Citation2015), but it differs in terms of the design of the inlet nozzle plate. The inlet nozzle plate design and the separation performance of the impactor are presented in the SI (Figures S3 and S4, respectively). In parallel, total PM2.5 was collected on a quartz fiber filter (8 × 10 inch, 2500QAT-UP; Pall Corp.) using a high-volume air sampler (HV-RW; Sibata Scientific Technology Ltd., Tokyo, Japan) operating at a flow rate of 740 L min−1. An impactor with a 2.5 mm cutoff diameter, similar to that used in the K-TRiC setup, was used upstream of the filter to remove coarse particles.
Figure 4. (a) Schematic diagram and (b) illustration of Keio-Transportable Real impactor with Cyclone (K-TRiC).
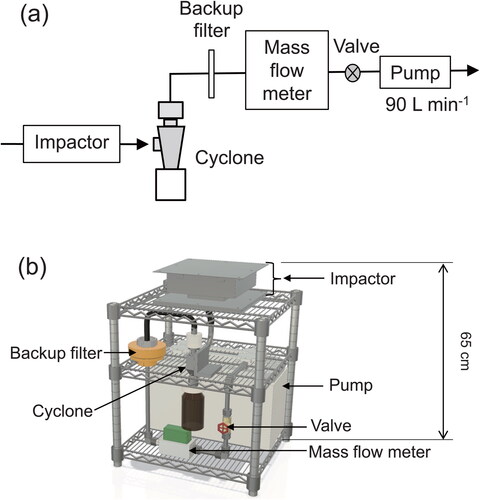
To collect PM samples in sufficient quantities to be used in toxicological experiments (at least tens of milligrams), the sampling duration was set as approximately two months per sample. After aerosol sampling, the PM samples adhering to the inside of the openable and URG cyclone were recovered by gently scraping them off on a piece of medicine paper using a stainless steel microspatula with a spoon width of 4 mm. For the openable cyclone, adhering PM samples were collected from the cyclone after opening its middle part (). For the URG cyclone, adhering PM samples were collected by scraping them out of the top and bottom openings of the cyclone. Although we did not have a strictly standardized procedure for sample PM recovery from the cyclones, our best efforts were made to recover as much PM as possible using these methods.
2.3.2. Metal analysis
The metal concentrations in the PM samples collected using the openable and URG cyclones were analyzed using the following procedure. First, 1 mg of the PM sample was decomposed in a mixture consisting of 5 mL of HNO3 (68%), 1 mL of H2O2 solution (35%), and 2 mL of HF (38%) using a microwave sample preparation system (Titan MPS; PerkinElmer Inc., Waltham, USA) at 200 °C for 30 min. After acid digestion, the resulting mixture was diluted using ultrapure water to a fixed volume of 50 ml. Subsequently, it was diluted 10-, 100-, and 1000-fold further using a water solution consisting 5% HNO3 and 1% HF. The concentrations of Na, Mg, Al, Si, P, K, Ca, Ti, V, Cr, Mn, Fe, Ni, Cu, Zn, and Pb in the diluted sample solutions were subsequently measured using an inductively coupled plasma mass spectrometer (iCAP-TQ; Thermo Fisher Scientific Inc., Waltham, USA).
2.3.3. Water-Soluble ions analysis
The concentrations of water-soluble ions in the PM samples collected using the openable and URG cyclones, as well as the total PM2.5 samples collected on the quartz fiber filter, were analyzed using the following procedure. For the PM samples collected by the cyclones, 2 mg of the PM sample was extracted by shaking for 15 min in 20 mL of ultrapure water (>18.2 MΩ cm). For the total PM2.5 samples collected on the quartz fiber filter, a filter punch (1 cm × 1 cm square) was extracted by shaking for 15 min in 10 mL of ultrapure water. The concentrations of Cl–, NO3–, SO42–, Na+, NH4+, K+, Mg2+, and Ca2+ in the water extracts were ascertained using ion chromatographs: an anion chromatograph (ICS-2100; Thermo Fisher Scientific Inc., Waltham, USA) equipped with an analytical column (AS18; Thermo Fisher Scientific Inc., Waltham, USA) and a guard column (AG18; Thermo Fisher Scientific Inc., Waltham, USA), and a cation chromatograph (ICS-1100; Thermo Fisher Scientific Inc., Waltham, USA) equipped with an analytical column (CS12A; Thermo Fisher Scientific Inc., Waltham, USA) and a guard column (CG12A; Thermo Fisher Scientific Inc., Waltham, USA). The eluents, 3.75 mM Na2CO3/0.1 mM NaHCO3 and 20 mM CH3SO3H, were used for anion and cation separation.
3. Results and discussion
3.1. Separation performance of the openable cyclone
presents the penetration efficiencies (P) of the openable and URG cyclones measured using AS and fluorescent PSL aerosols at a flow rate of 90 L min−1. Based on the regression curves fitted on the experimentally obtained P values using the AS aerosol, Dae50 for the openable and URG cyclones were determined as approximately 0.200 and 0.144 µm. The values of k in the logistic function (EquationEquation (4)(4)
(4) ) were approximately 2.58 and 2.56, respectively, for the openable and URG cyclones. The values of GSD were approximately 1.88 and 1.91, respectively, for the openable and URG cyclones. These results indicate that the openable cyclone exhibits separation performance that is similar to that of the URG cyclone. Furthermore, the P values of the openable cyclone measured using fluorescent PSL particles agree well with those measured for the AS aerosol, suggesting that the penetration curves obtained using AS particles have no significant error. The reason for the slight difference between the penetration curves of the openable and URG cyclones, even though their dimensions are almost identical, is unclear. Air leakage from the openable cyclone, metalworking precision, different surface finish, and experimental errors might be causes of the slight difference in the penetration curves. In an urban atmosphere, the mass–size distributions of the fine particles typically exhibit a mode at approximately 0.3 µm in diameter (Seinfeld and Pandis Citation2016). Therefore, using either the openable or URG cyclone at a flow rate of 90 L min−1, approximately half of PM2.5 mass can be collected in an urban atmosphere.
Figure 5. Penetration efficiencies (P) of the openable and URG cyclones measured using AS and fluorescent PSL aerosols. The points and error bars, respectively, represent the average values and standard deviations of five measurements. The lines (black and magenta) represent results of fitting using the logistic function.
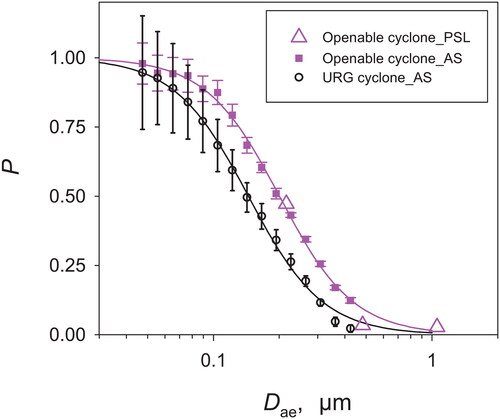
3.2. Sampling yields of the atmospheric PM sampling using the openable and URG cyclones
This section presents comparison of the sampling yields of PM sampling using the openable and URG cyclones. The sampling yield refers to the mass fraction of the obtained PM sample relative to the total PM2.5 mass in the total sample air volume drawn into the sampler. presents the dataset used to calculate the sampling yield, including the average atmospheric PM2.5 concentration during the sampling period, total sample air volume, and the collected PM mass. The average atmospheric PM2.5 concentrations were calculated from the PM2.5 concentrations measured at a monitoring station (Kohoku-ku sougou-chousha station) managed by Yokohama city, located 4.5 km distant from the sampling site, because we did not have a device to measure PM2.5 mass concentrations at the Keio University site. Atmospheric observations conducted during a different period from this study have shown that PM2.5 mass concentrations at the Keio University site and the monitoring site of Yokohama city are almost identical (Okuda et al. Citation2019).
Table 1. Average PM2.5 concentrations, total sample air volumes, collected PM masses, and sampling yieldsTable Footnotea of the PM sampling using the openable and URG cyclones.
The sampling yields for the openable cyclone sampling were 29%–38% (35% on average), whereas the yields for the URG cyclone sampling were 22%–33% (27% on average). A statistical test (t test) demonstrated that the sampling yield of the openable cyclone was significantly higher than that of the URG cyclone at a significance level of p < 0.05, which indicates that the openable cyclone allows for more effective collection of the PM sample than that provided by the URG cyclone. presents photographs of the inner wall of the middle part of the openable cyclone (a) before and (b) after sampling. After sampling, substantial amounts of sample PM were observed to have been deposited, particularly on the inner walls upstream of the cylinder (indicated by a gray arrow in ) and downstream of the cone (indicated by a white arrow in ). In the case of unopenable small cyclones such as the URG cyclone, collecting the PM adhering to the cone is difficult. However, the openable cyclone allows for easy collection of almost all PM adhering to its inner wall because of its openable design. This unique feature of the openable cyclone, enabling effective sampling of the PM adhering to the cyclone, is expected to contribute considerably to the increased yield of the PM sample.
3.3. Concentrations of metals and water-soluble ions in the PM samples collected using the openable and URG cyclones and filters
3.3.1. Metals
To compare the concentrations of metals in the PM samples collected using the openable and URG cyclones, the ratios of the metal concentration in the openable-cyclone-collected PM sample were calculated with respect to the concentration of the same metal in the URG-cyclone-collected PM sample ([X]opn/[X]URG for element X). presents the elemental concentrations of Na, Fe, Si, K, Ca, Al, Mg, Zn, P, Mn, Cr, Cu, Ti, Ni, Pb, and V in the openable-cyclone-collected and URG-cyclone-collected PM samples. It also presents the [X]opn/[X]URG ratios during (a) summer, (b) autumn, and (c) winter. It is noteworthy that the elemental concentrations presented in are the concentrations in the PM samples collected inside the cyclones, but not the concentrations in the atmosphere. The [Al]opn/[Al]URG ratios were invariably less than unity in all samples (0.20–0.32), indicating a significant excess of Al in the URG-cyclone-collected samples, which might mostly originate from the aluminum material of the cyclone itself. Assuming that the excess Al in the URG-cyclone-collected samples was derived entirely from the URG-cyclone-material, the estimated mass concentrations of cyclone-origin Al2O3 in the URG-cyclone-collected PM samples were approximately 3.0% to 4.2% (4% on average).
Figure 7. Concentrations of metals in PM samples collected using the openable and the URG cyclones, and their ratios ([X]opn/[X]URG ratios) during three seasons: (a) summer, (b) autumn, (c) winter, and (d) average [X]opn/[X]URG ratio. For panels (a), (b), and (c), the right y-axis shows the [X]opn/[X]URG ratio. For panel (d), the thin and thick (black and magenta) lines inside the box, respectively, represent median and mean values. Lower and upper ends of boxes, respectively, denote lower and upper quartiles.
![Figure 7. Concentrations of metals in PM samples collected using the openable and the URG cyclones, and their ratios ([X]opn/[X]URG ratios) during three seasons: (a) summer, (b) autumn, (c) winter, and (d) average [X]opn/[X]URG ratio. For panels (a), (b), and (c), the right y-axis shows the [X]opn/[X]URG ratio. For panel (d), the thin and thick (black and magenta) lines inside the box, respectively, represent median and mean values. Lower and upper ends of boxes, respectively, denote lower and upper quartiles.](/cms/asset/ac772722-ee48-4b34-a443-67fbc90deaff/uast_a_2322680_f0007_c.jpg)
In contrast, the [Cr]opn/[Cr]URG and [Ni]opn/[Ni]URG ratios were consistently greater than unity in all samples (3.4–45 for Cr and 2.2–12 for Ni). The openable cyclone material (stainless steel, X5CrNi18-10) contains substantial amounts of Cr and Ni (18% and 8% by weight in the stainless steel, respectively). Therefore, the excess Cr and Ni in the openable-cyclone-collected samples might have derived mostly from the openable-cyclone material itself. Assuming that the entire excess Cr and Ni are from stainless steel, the estimated mass concentrations of stainless steel in the openable-cyclone-collected PM samples were approximately 0.4%–1.5% (1% on average) and 0.3%–1.5% (0.8% on average), respectively, which are significantly lower than the estimated cyclone-origin Al2O3 concentration in the URG-cyclone-collected samples (4% on average). This result suggests that the risk of sample contamination from the cyclone material can be reduced by changing the cyclone material from aluminum to stainless steel. No report of the relevant literature has presented clear evidence indicating that the toxicity of stainless steel particles is greater than that of Al2O3 particles (Lanone et al. Citation2009). Therefore, the influence of cyclone material contamination to the toxicological experiments might also be reduced when the cyclone material is changed from aluminum to stainless steel.
Except for Al, Cr, and Ni, the [X]opn/[X]URG ratios of the metals were approximately unity, but slightly higher than unity for most metals. In the summer and autumn samples, the Fe concentrations in the openable-cyclone-collected samples were approximately 0.8% higher than those in the URG-cyclone-collected samples. This excess Fe concentration, 0.8%, is consistent with the stainless steel concentration (approximately 1%) estimated from the excess Cr or Ni concentration because Fe concentration in the stainless steel is 74%. Therefore, for the summer and autumn samples, the excess Fe in the openable-cyclone-collected sample is mostly attributable to the contamination from the openable cyclone material. However, in the winter sample, the excess Fe concentration was found to be 2.4%, which is significantly greater than in the summer and autumn samples. This excess Fe concentration cannot be explained fully by stainless steel contamination alone. Moreover, the higher [X]opn/[X]URG ratios of the metals not included in stainless steel are not explainable by the stainless steel contamination. Except for Al, the concentrations of the metals in the URG-cyclone-collected samples are expected to be more diluted by the cyclone material than those in the openable-cyclone-collected samples. Based on the estimated concentrations of cyclone-origin Al2O3 and stainless steel (1% and 4%, respectively), the [X]opn/[X]URG ratios are estimated as increasing by a factor of approximately 1.03 (104/101) as a result of the dilution, except for Al, Cr, Ni, and Fe, which only slightly explain the observed [X]opn/[X]URG ratios (e.g., 1.25–1.69 for [Na]opn/[Na]URG, 1.11–1.67 for [Si]opn/[Si]URG, and 1.12–1.35 for [K]opn/[K]URG). The particle sizes of the PM samples collected from the inner wall of the openable and URG cyclones are likely to differ because the particles adhering to the cone, where lightweight (smaller) particles might tend to adhere, were collected only slightly from the URG cyclone, as described in Section 3.2. Therefore, in addition to the cyclone material contamination, particle size differences might also contribute to the differences in the metal concentrations between the openable-cyclone-collected and URG-cyclone-collected samples.
3.3.2. Water-soluble ions
presents the concentrations of SO42–, NO3–, NH4+, Ca2+, Na+, K+, and Mg2+ in the PM samples collected using the openable and URG cyclones, as along with their ratios ([X]opn/[X]URG for ion X), during (a) summer, (b) autumn, and (c) winter. In summer and autumn, SO42– was the dominant ion by mass among the measured water-soluble ions in both the openable and URG cyclone-collected samples. However, in the winter sample, both SO42– and NO3– concentrations were dominant. The higher concentration of nitrate in the winter sample can be attributed to the low air temperature, which favors nitrate formation in the atmosphere (Seinfeld and Pandis Citation2016). The compositions of water-soluble ions in the PM samples collected using the openable and URG cyclones were comparable, with SO42– being the dominant ion in the summer and autumn samples, and with both SO42– and NO3– showing significant contributions in the winter sample. Although the [X]opn/[X]URG ratios were consistently close to unity for all ions measured for this study, the concentrations of some ions were slightly higher in the openable-cyclone-collected samples compared to the URG-cyclone-collected samples. For example, the ratios of [SO42–]opn/[SO42–]URG were approximately 1.21–1.35. The ratios of [NH4+]opn/[NH4+]URG were approximately 1.30–1.74. Similarly to the concentrations of the metals, this difference of the ion concentrations might be attributable to differences of the particle sizes between the openable-cyclone-collected and URG-cyclone-collected samples.
Figure 8. Concentrations of water-soluble ions in PM samples collected using the openable and the URG cyclones and in PM2.5 samples collected using filters, and their ratios ([X]opn/[X]URG and [X]opn/[X]PM2.5) during three seasons: (a) summer, (b) autumn, and (c) winter, and average (d) [X]opn/[X]URG and (e) [X]opn/[X]PM2.5. For panels (a), (b), and (c), the right y-axis shows the [X]opn/[X]URG (shown by circles) and [X]opn/[X]PM2.5 ratios (shown by triangles). For panels (d) and (e), The thin (black) lines inside the box represent median values; the thick (magenta or blue) lines represent mean values. Lower and upper ends of boxes, respectively, denote lower and upper quartiles.
![Figure 8. Concentrations of water-soluble ions in PM samples collected using the openable and the URG cyclones and in PM2.5 samples collected using filters, and their ratios ([X]opn/[X]URG and [X]opn/[X]PM2.5) during three seasons: (a) summer, (b) autumn, and (c) winter, and average (d) [X]opn/[X]URG and (e) [X]opn/[X]PM2.5. For panels (a), (b), and (c), the right y-axis shows the [X]opn/[X]URG (shown by circles) and [X]opn/[X]PM2.5 ratios (shown by triangles). For panels (d) and (e), The thin (black) lines inside the box represent median values; the thick (magenta or blue) lines represent mean values. Lower and upper ends of boxes, respectively, denote lower and upper quartiles.](/cms/asset/5a4ea743-688a-45a1-b58c-aaf43be8cb2e/uast_a_2322680_f0008_c.jpg)
Additionally, we compared the concentrations of water-soluble ions in the openable-cyclone-collected samples with those in the total PM2.5 collected on the filters. The [X]opn/[X]PM2.5 ratios, shown as blue squares in , represent the ratios of the concentration of ion X in the openable-cyclone-collected PM samples to that in the total PM2.5 collected on filters. The compositions of water-soluble ions in the openable-cyclone-collected samples were comparable also to the total PM2.5, with SO42– being the dominant ion in the summer and autumn samples, with both SO42– and NO3– showing significant contributions in the winter sample. However, the [Ca2+]opn/[Ca2+]PM2.5 ratios were much lower than unity for all seasons (0.37–0.45). Actually, Ca2+ in the atmosphere, present mostly in the coarse particles, originates mainly from mineral dusts. Therefore, the low [Ca2+]opn/[Ca2+]PM2.5 ratios might result from the performance of the impactor upstream of the cyclone or filters. Except for Ca2+, the [X]opn/[X]PM2.5 ratios were close to unity for all ions. Despite the limitation of not collecting particles smaller than 0.2 μm, as shown in , the concentrations of water-soluble ions in the PM samples collected using the openable cyclone were similar to those in the total PM2.5. This result suggests that the chemical composition of the PM samples collected using the openable cyclone is representative of the bulk chemical composition of PM2.5, although the samples themselves might not be completely identical to one another.
4. Conclusions
After developing a small openable cyclone for the effective sampling of atmospheric PM, we compared its performance with that of a commercially available cyclone of similar dimensions but without an openable design. The penetration efficiency curves of both cyclones were ascertained by experimentation using aerosols containing ammonium sulfate particles or fluorescent polystyrene latex particles. They were found to be very similar. The openable cyclone had an aerodynamic cutoff diameter of approximately 0.2 µm at a flow rate of 90 L min−1. To evaluate the sampling performance of the openable cyclone and its ability to collect representative atmospheric PM samples, we applied the cyclones to collect atmospheric PM. Sampling yields were 35%, on average, for the openable cyclone when operated at a flow rate of 90 L min−1. This yield was approximately 1.3 times higher than that of the unopenable commercial cyclone. The openable design of the newly developed cyclone increased the obtained sample mass considerably. Metal analysis of the cyclone-collected PM samples revealed contamination from the cyclone materials. Comparison of the metal concentrations in the PM samples collected using the openable and commercial cyclones, which are made, respectively, of stainless steel and aluminum, demonstrated that the aluminum cyclone was more likely to cause sample contamination than the stainless steel cyclone. The concentrations of water-soluble ions in the openable-cyclone-collected samples were similar to those in the total PM2.5 collected on the filters, suggesting that the chemical composition of the openable-cyclone-collected sample is similar to that of the total PM2.5. These results demonstrate that PM samples collected using the openable cyclone are promising for use in toxicological experiments to evaluate the health effects of PM2.5 and to elucidate its toxicity mechanisms.
Supplemental Material
Download MS Word (109.7 KB)Acknowledgments
The authors are grateful to K. Watanabe for metal machining, to A. Suizu for IC analysis, and to the Seikan Kensa Center Inc. for ICP-MS analysis.
Disclosure statement
No potential conflict of interest was reported by the author(s).
Additional information
Funding
References
- Alessandria, L., T. Schilirò, R. Degan, D. Traversi, and G. Gilli. 2014. Cytotoxic response in human lung epithelial cells and ion characteristics of urban-air particles from Torino, a northern Italian city. Environ. Sci. Pollut. Res. Int. 21 (8):5554–64. doi: 10.1007/s11356-013-2468-1.
- Alimov, Z. B., H. Youn, A. Iwata, K. Nakano, T. Okamoto, A. Sasaki, T. Katori, and T. Okuda. 2022. Comparison of the chemical characteristics and toxicity of PM2.5 collected using different sizes of cyclones. Asian J. Atmos. Environ. 16 (3):103–21. doi: 10.5572/ajae.2022.062.
- Azadi, M., M. Azadi, and A. Mohebbi. 2010. A CFD study of the effect of cyclone size on its performance parameters. J. Hazard. Mater. 182 (1–3):835–41. doi: 10.1016/j.jhazmat.2010.06.115.
- Brar, L. S., R. P. Sharma, and K. Elsayed. 2015. The effect of the cyclone length on the performance of Stairmand high-efficiency cyclone. Powder Technol. 286:668–77. doi: 10.1016/j.powtec.2015.09.003.
- Brook, R. D., S. Rajagopalan, C. A. Pope, J. R. Brook, A. Bhatnagar, A. V. Diez-Roux, F. Holguin, Y. Hong, R. V. Luepker, M. A. Mittleman, et al. 2010. Particulate matter air pollution and cardiovascular disease. Circulation 121 (21):2331–78. doi: 10.1161/CIR.0b013e3181dBece1.
- Cavanagh, J.-A E., K. Trought, L. Brown, and S. Duggan. 2009. Exploratory investigation of the chemical characteristics and relative toxicity of ambient air particulates from two New Zealand cities. Sci. Total Environ. 407 (18):5007–18. doi: 10.1016/j.scitotenv.2009.05.020.
- Calvo, A. I., C. Alves, A. Castro, V. Pont, A. M. Vicente, and R. Fraile. 2013. Research on aerosol sources and chemical composition: Past current, and emerging issues. Atmos. Res. 120–121:1–28. doi: 10.1016/j.atmosres.2012.09.021.
- Cohen, A. J., M. Brauer, R. Burnett, H. R. Anderson, J. Frostad, K. Estep, K. Balakrishnan, B. Brunekreef, L. Dandona, R. Dandona, et al. 2017. Estimates and 25-year trends of the global burden of disease attributable to ambient air pollution: An analysis of data from the global burden of diseases study 2015. Lancet 389 (10082):1907–18. doi: 10.1016/S0140-6736(17)30505-6.
- Dockery, D. W., and C. A. Pope. 1994. Acute respiratory effects of particulate air pollution. Annu. Rev. Public Health. 15 (1):107–32. doi: 10.1146/annurev.pu.15.050194.000543.
- Fu, P.-B., F. Wang, X.-J. Yang, L. Ma, X. Cui, and H.-L. Wang. 2017. Inlet particle-sorting cyclone for the enhancement of PM2.5 separation. Environ. Sci. Technol. 51 (3):1587–94. doi: 10.1021/acs.est.6b04418.
- Heo, J., D. S. Antkiewicz, M. M. Shafer, D. A. Perkins, C. Sioutas, and J. J. Schauer. 2015. Assessing the role of chemical components in cellular responses to atmospheric particle matter (PM) through chemical fractionation of PM extracts. Anal. Bioanal. Chem. 407 (20):5953–63. doi: 10.1007/s00216-015-8749-4.
- Hinds, W. C. 1982. Aerosol technology. New York: John Wiley & Sons, Inc.
- Hoek, G., R. M. Krishnan, R. Beelen, A. Peters, B. Ostro, B. Brunekreef, and J. D. Kaufman. 2013. Long-term air pollution exposure and cardio-respiratory mortality: A review. Environ. Health 12 (1):43. doi: 10.1186/1476-069X-12-43.
- Honda, A., T. Okuda, M. Nagao, N. Miyasaka, M. Tanaka, and H. Takano. 2021. PM2. 5 collected using cyclonic separation causes stronger biological responses than that collected using a conventional filtration method. Environ. Res. 198:110490. doi: 10.1016/j.envres.2020.110490.
- Iozia, D. L., and D. Leith. 1990. The logistic function and cyclone fractional efficiency. Aerosol. Sci. Technol. 12 (3):598–606. doi: 10.1080/02786829008959373.
- Kim, J. H., G. W. Mulholland, S. R. Kukuck, and D. Y. Pui. 2005. Slip correction measurements of certified PSL nanoparticles using a nanometer differential mobility analyzer (nano-DMA) for Knudsen number from 0.5 to 83. J. Res. Natl. Inst. Stand. Technol. 110 (1):31–54. doi: 10.6028/jres.110.005.
- Kurihara, K., A. Iwata, S. G. Murray Horwitz, K. Ogane, T. Sugioka, A. Matsuki, and T. Okuda. 2022. Contribution of physical and chemical properties to dithiothreitol-measured oxidative potentials of atmospheric aerosol particles at urban and rural sites in Japan. Atmosphere. 13 (2):319. doi: 10.3390/atmos13020319.
- Lanone, S., F. Rogerieux, J. Geys, A. Dupont, E. Maillot-Marechal, J. Boczkowski, G. Lacroix, and P. Hoet. 2009. Comparative toxicity of 24 manufactured nanoparticles in human alveolar epithelial and macrophage cell lines. Part. Fibre Toxicol. 6 (1):14. doi: 10.1186/1743-8977-6-14.
- Lelieveld, J., J. S. Evans, M. Fnais, D. Giannadaki, and A. Pozzer. 2015. The contribution of outdoor air pollution sources to premature mortality on a global scale. Nature 525 (7569):367–71. doi: 10.1038/nature15371.
- Moore, M. E., and A. R. McFarland. 1993. Performance modeling of single-inlet aerosol sampling cyclones. Environ. Sci. Technol. 27 (9):1842–8. doi: 10.1021/es00046a012.
- Nassaj, O. R., D. Toghraie and, and M. Afrand. 2019. Effects of multi inlet guide channels on the performance of a cyclone separator. Powder Technol. 356:353–72. doi: 10.1016/j.powtec.2019.08.038.
- Okuda, T., R. Isobe, Y. Nagai, S. Okahisa, K. Funato, and K. Inoue. 2015. Development of a high-volume pm2.5 particle sampler using impactor and cyclone techniques. Aerosol Air Qual. Res. 15 (3):759–67. doi: 10.4209/aaqr.2014.09.0194.
- Okuda, T., and R. Isobe. 2017. Improvement of a high-volume aerosol particle sampler for collecting submicron particles through the combined use of a cyclone with a smoothened inner wall and a circular cone attachment. Asian J. Atmos. Environ. 11 (2):131–7. doi: 10.5572/ajae.2017.11.2.131.
- Okuda, T., D. Shishido, Y. Terui, K. Fujioka, R. Isobe, Y. Iwaki, K. Funato, and K. Inoue. 2018. Development of a high-volume simultaneous sampler for fine and coarse particles using virtual impactor and cyclone techniques. Asian J. Atmos. Environ. 12 (1):78–86. doi: 10.5572/ajae.2018.12.1.078.
- Okuda, T., M. Sakaide, K. Fujioka, R. Tabata, K. Kurosawa, Y. Nomura, A. Iwata, and M. Fujiwara. 2019. Investigation of the characteristics of particulate matter suspended in a subway station platform. J Japan Soc Atmosph Environ 54:28–33. doi: 10.11298/taiki.54.28.
- Pope, C. A., III,., and D. W. Dockery. 2006. Health effects of fine particulate air pollution: Lines that connect. J. Air Waste Manag. Assoc. 56 (6):709–42. doi: 10.1080/10473289.2006.10464485.
- Roper, C., L. S. Delgado, D. Barrett, S. L. Massey Simonich, and R. L. Tanguay. 2019. Pm2.5 filter extraction methods: Implications for chemical and toxicological analyses. Environ. Sci. Technol. 53 (1):434–42. doi: 10.1021/acs.est.8b04308.
- Seinfeld, J. H., and S. N. Pandis. 2016. Atmospheric chemistry and physics: From air pollution to climate change. Hoboken: Wiley.
- Van Winkle, L. S., K. Bein, D. Anderson, K. E. Pinkerton, F. Tablin, D. Wilson, and A. S. Wexler. 2014. Biological dose response to pm2.5: Effect of particle extraction method on platelet and lung responses. Toxicol. Sci. 143 (2):349–59. doi: 10.1093/toxsci/kfu230.
- Yoshida, H., Y. Hayase, K. Fukui, and T. Yamamoto. 2012. Effect of conical length on separation performance of sub-micron particles by electrical hydro-cyclone. Powder Technol. 219:29–36. doi: 10.1016/j.powtec.2011.12.002.
- Zhang, P., G. Chen, J. Duan, and W. Wang. 2019. Experimental evaluation of separation performance of fine particles of circulatory circumfluent cyclone separator system. Sep. Purif. Technol. 210:231–5. doi: 10.1016/j.seppur.2018.08.008.
- Zhang, Y., R. Jin, S. Dong, Y. Wang, K. Dong, Y. Wei, and B. Wang. 2021. Heterogeneous condensation combined with inner vortex broken cyclone to achieve high collection efficiency of fine particles and low energy consumption. Powder Technol. 382:420–30. doi: 10.1016/j.powtec.2021.01.003.