Abstract
A new type of resin wool filter (RWF) that persists the load with oil droplets was developed by Kimura and colleagues. In the present work, the initial collection performances of RWF (A and C) are measured for various particle sizes (0.03, 0.05, 0.1, 0.15, 0.2, 0.25, and 0.3 μm) with different charging states at various filtration velocities (0.05, 0.1, 0.15, and 0.3 m/s). As a result, it is shown that the present RWF impregnated with PTBP resin can attain high collection efficiency (99.999% at filtration velocity of 0.05 m/s) with a pressure drop of less than 30 Pa. The charge density is estimated by applying prediction equations of single-fiber collection efficiencies of electret filters with a dipolar charge distribution because no other prediction equation for RWF are available at present. The experimental single-fiber efficiencies for uncharged particles are successfully predicted by assigning a single value of charge density in the prediction equations for dipolar fibers. The estimated charge density on RWF fibers is 2.1 × 10− 4 C/m2, which is much higher than those of conventional electret filter media. Therefore, RWF studied in the present work is suitable for the application to respirators as well as room air cleaners.
INTRODUCTION
Electrically charged filter media have been commonly used for dust respirators because they have high collection efficiency at a low pressure drop compared to mechanical filters. The oldest type of electrically active filter media is a resin wool filter (RWF), or Hansen filter. In 1930s, the RWF was invented by Hansen, and it was the first filter used for respirators of military use (CitationHinds 1999). Since the Hansen filter was invented, various types of electrically active filter media such as polypropylene electret filters, split electret filters, and melt-brown electret filters were developed. In order to clarify the collection mechanisms of charged filter media, much research has been conducted with theoretical approaches (CitationEmi and Kanaoka 1984; CitationBaumgartner and Loffler 1986; CitationPich et al. 1987; CitationBrown et al. 1988; Tanthapanichkoon et al. 2003) and experimental approaches (CitationWalsh and Stenhouse 1997a, Citationb; CitationJi et al. 2003). They showed that the electrical charge exponentially decays with particle load, leading to a decrease in the collection efficiency. Especially, electret filter media are apt to lose their charge readily when loaded with oil mist of a relatively large dielectric constant (CitationOtani et al. 1993). CitationRomay et al. (1998) compared the collection efficiencies of three types of electret filter media and estimated the effective surface electric field in these electret filters.
Much effort has gone into increasing the charge density while prolonging the filter life by minimizing the influence of captured particles. CitationLee et al. (2002) studied a melt-brown electret filter (high performance electret filter; HPEF) with a high charge density and estimated the charge density. CitationLeonard and Alan (1998) investigated the performance for liquid aerosols on various types of electret filter media and developed a new type of a melt-brown electret filter (BMF) with a high charge density. The new type of BMF had a high resistance to droplets by lowering degradation in overall collection efficiency due to droplet load because of its high charge density. Despite these efforts, the deficit of electret filters, i.e., the collection efficiency that decreases with particle load, has not yet been overcome.
RWFs, however, have an advantage over electret filters because the charge density and the surface property of the filter are easily adjusted by impregnating various reagents. Recently, CitationKimura et al. (2004) have developed a new type of RWF that retains a high charge density and persists organic droplet challenge. The RWF media have been successfully applied to dust respirators, but the detailed electrification mechanisms and collection performance are not yet well understood.
In the present work, we studied the filtration performance of the newly developed RWF impregnated with p-t-butyl-phenol-formaldehyde (PTBP) resin particles. The objectives of present work are as follows:
1. | evaluation of initial performances of RWFs for liquid organic particles with different sizes at various filtration velocities, | ||||
2. | comparison of collection performance of RWF and that of the conventional electret filters consisting of melt-brown polypropylene fibers, | ||||
3. | applicability of prediction equations of single-fiber collection efficiencies of electret filter to the single-fiber collection efficiencies of RWF, and | ||||
4. | estimation of charge density on RWF by applying the prediction equation of single-fiber efficiency of electret filters. |
MATERIAL AND METHOD
Filter Media
The RWF studied in the present work is shown in . The RWF consists of three layers, i.e., a wool layer without resin is sandwiched by the layers with resin particles. The raw wool filter is a mixture of merino wool and polyester fibers with the diameter of about 15 μ m. PTBP resin particles are impregnated on the fibers by soaking the filter with the resin solution followed by the breakage of cured resin. Since the fiber diameter of RWF is larger than that of the conventional electret filters (about 10 times the diameter of melt-brown electret fibers studied by CitationLee et al. (2002)), RWF has a lower pressure drop at the same packing density of the electret filter.
Two RWF (A and C) were prepared to meet the requirements of NIOSH 42 CFR 84 P95 and P99 (CitationNIOSH 1995). The raw wool filters used were different in mass per unit filter area, filter thickness, and the packing density. Resins were impregnated in these filters at the same mass fraction. The properties of test filter media are shown in .
Table 1 Properties of raw wool filters and RWF (A and C)
Experimental Setup
The experimental setup for the measurement of initial collection efficiency is shown in . Test aerosol of Dioctyl phthalate (DOP) is generated with an evaporation–condensation-type aerosol generator, which are composed of four Laskin nozzles and condenser. The test aerosol particles are electrically neutralized by α -ray irradiation (241Am) and classified into monodisperse particles with a diameter from 0.03 to 0.3 μ m using a differential mobility analyzer (laboratory-made DMA) which was calibrated by TSI-made DMA (SMPS, TSI Model 3080L). The charging state of particles is regulated using a parallel-plate electrostatic precipitator and a 241Am neutralizer. The air flow rate through the filters is regulated by a mass flow controller (SEC-500-MK3 STEC Inc). The filtration velocities set by the mass flow controller are 0.05, 0.1, 0.15, and 0.3 m/s. The range of filtration velocity covers the filtration velocity at which air flows through a respirator (CitationNIOSH 1995). The filtration area of the filter is 40 mm in diameter, and the thickness of the filter is maintained with spacers by avoiding the filter compression. Aerosol particle concentrations at the inlet and outlet of test filter are simultaneously measured using two CNC (condensation nucleus counter, TSI Model-3022A) that are calibrated to give the same reading. The initial pressure drop is measured using a pressure transducer (PDR-C-2C MKS Inc).
Estimation of Charge Density on RWF
There are several ways to estimate the charge density of filters, e.g., the measurement of amount of ions necessary to neutralize electrical charge on a filter, and the fitting of experimental collection efficiency data with theoretical equations. CitationRomay et al. (1999) proposed a method for the measurement of charge density that employed the neutralization of electrical charge with bipolar ions generated by Alpha-ray irradiation. However, since the recombination of bipolar ions in a filter is inevitable, the charge density predicted by this method would be overestimated, as suggested by CitationLee et al. (2002). Further research is necessary for the determination of charge density with a neutralization technique.
Another method to predict the charge density on electret filter is to find the charge density that best fits the experimental collection efficiency with the theoretical equations. For prediction of single-fiber efficiency of electret filter with dipolar charge on fibers, CitationBrown (1981) derived the equations of single-fiber collection efficiencies due to induced and Coulombic forces without mechanical effects. Later, CitationOtani et al. (1993) modified the equations applicable to wide ranges of induced and Coulombic force parameters:
CitationOtani et al. (1993) assumed the additivity of single-fiber collection efficiencies, i.e., ηInM = ηM + ηIn and ηCInM = ηInM + ηC, and obtained ηM exp, ηIn exp, and ηC exp from the penetration of uncharged particles through uncharged filter, P M, the penetration of uncharged particles through charged filter, P InM, and the penetration of singly charged particles through charged filter, P CInM:
RESULTS AND DISCUSSION
Initial Pressure Drop of RWF
The initial pressure drops of both raw wool filter and RWF are plotted as a function of face velocity in . The initial pressure drops of both raw wool filters increase linearly with the filtration velocity and can be predicted with the drag force proposed by CitationDavies (1952) by letting α = 0.049 and 0.068, respectively, for RWFs (A and C):
As shown in , for both RWFs (A and C) the pressure drops of RWF are increased by the impregnation of resin, yet they hold the linear relationship between the pressure drop and filtration velocity. Based on Davis equation, the contribution of impregnated resin to the packing density of filter is about 10–20% for both RWFs.
We need the packing density of the filter to calculate the total fiber length in a unit filter area so that the filter collection efficiency is converted to the single-fiber efficiency via log penetration of Equations (9)–(11). Because the impregnation of resin particles does not affect the fiber length, we used the packing density of raw filter to convert the overall collection efficiency to the single-fiber efficiency.
Initial Penetrations of RWF
The penetrations of uncharged and singly charged particles through RWF A and C as well as uncharged filters (raw wool filters) are compared in at the filtration velocity of 0.05 m/s. There is no difference in the penetration of charged and uncharged particles through uncharged filter (RWF A), indicating that the raw filter does not retain any electrical charge.
Figure 4 Penetrations of uncharged and singly charged particles through raw wool filters and RWF (A and C).
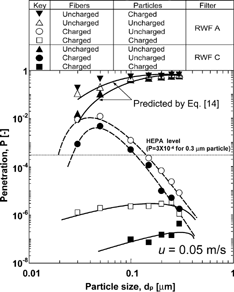
The particle penetrations due to Brownian diffusion predicted by Equation (Equation14) are also shown in . Because the test particle sizes are small and the fiber diameter is large, the interception effect is small. The single-fiber collection efficiency due to Brownian diffusion is given by CitationKirsch and Fuchs (1968) as
The particle penetrations, P InM, through RWFs (A and C) for uncharged particles have the maximum at the particle size of about 40 nm, indicating that induced force is the dominant collection mechanism for particles larger than 40 nm, while Brownian diffusion dominates the capture of particles smaller than 40 nm. The penetrations, P CInM, through RWFs (A and C) for singly charged particles are smaller than P = 1 × 10−6, which is close to the detection limit of the present experimental setup, having very weak dependence on the particles size. This is because Coulombic force increases with decreasing particle size, while the induced force increases with particle size so that both forces would compensate each other, eliminating the dependence of particle penetration on the particle size. Incidentally, the penetrations through both RWFs for particles larger than 0.3 μ m in diameter are smaller than P = 3 × 10− 4, which satisfies the requirement of HEPA filters, although RWF is composed of coarse wool fibers.
Comparison of RWF and Conventional Electret Filter
compares the experimental penetrations of RWF A and the electret polypropylene filter measured by CitationRomay et al. (1998) at u = 0.1 m/s. CitationRomay et al. (1998) measured the initial performances of electret polypropylene filters by using NaCl particles. Since the properties of electret filter used by CitationRomay et al. (1998) are close to those of RWF A as shown in , enables us to compare the electrostatic effects on particle collection by RWF A and the electret filter. The dependence of penetrations of uncharged particles through RWF A on particle size is more pronounced compared to the electret filter, indicating that RWF retains more charge than the electret filter. Because the induced force exerts on particles with smaller sizes due to the high charge density of RWF, the most penetrating particle size (MPPS) of RWF A is about 40 nm. Furthermore, the penetration of singly charged particles, P CInM, through RWF A is considerably low compared to the electret filter because the induced force is effective for the collection of charged particles in RWF.
Table 2 Properties of RWF A and conventional electret filters
Figure 5 Comparison of initial penetrations through RWF A and that through electret polypropylene filter measured by CitationRomay et al. (1998).
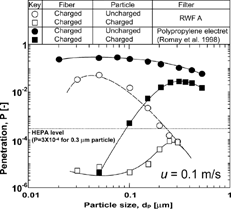
In , the penetrations of RWF A for uncharged and singly charged particles are compared with those of electret filter at various filtration velocities measured by CitationLee et al. (2002). CitationLee et al. (2002)measured the initial performance of melt-brown electret filter with a high charge density using ZnCl2 particles. The penetrations curves of RWF A are similar to those of the electret filter, although the properties of the filter studied by CitationLee et al. (2002) were made of fine fibers with a higher packing density compared to RWF A, as shown in . It is concluded from these comparisons that the RWF studied in this work retains a higher charge density than that of the conventional electret filters, having higher collection performance for both uncharged and charged particles.
Figure 6 Comparison of initial penetrations through RWF A and through melt-brown electret filter measured by CitationLee et al. (2002).
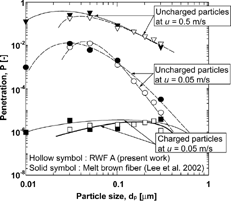
Charge Density on RWF
As shown in , the RWF studied in the present work consists of the fibers and the resin particles with a volume comparable to the volume of fibers. The resin particles without electrical charge would contribute to an increase in the mechanical collection efficiency, especially raising the interception efficiency, but the RWF with resin particles without electrical charge is not available. Consequently, we neglected the mechanical collection by the resin particles, assuming that the electrostatic efficiency is sufficiently large compared to the mechanical one, i.e., P M = 1 in Equation (Equation9). Furthermore, Equations (1)–(5) were derived for the electret filters with dipolar charges on fibers, which is different from the charging state of RWF fibers. Since we do not have any other model that describes the particle collection by RWF where the charged resin particles adhered on fibers capture particles, Equations (1)–(5) are applied to estimate the charge density of RWF.
The penetrations of uncharged particles through RWF A are converted to the single-fiber collection efficiencies using Equation (Equation10), and they are plotted against the induced force parameter, K In. In calculating K In, the charge density is assumed at Q f = 2. 1 × 10− 4 C/m2. As shown in , the experimental efficiencies, ηIn exp, for RWF A at various filtration velocities and particle sizes fall on a single line with the slope in agreement with the power of K In in Equation (Equation7).
Figure 7 Experimental single-fiber collection efficiency due to induced force as a function of induced force parameter for RWF A.
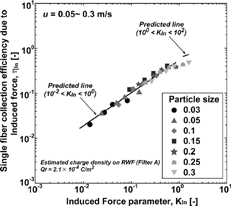
In , the single-fiber collection efficiency due to induced force for RWF C is plotted against the induced force parameter calculated with the charge density obtained for RWF A. We can see reasonably good agreement in , suggesting that RWFs with the same mass fraction of resin might retain the same charge density. Using the same approach, CitationLee et al. (2002) estimated the charge density of melt-brown electret filter to be Q f = 5.2 × 10− 5 C/m2, which is about one-forth of the charge density of RWFs studied in the present work.
Figure 8 Experimental single-fiber collection efficiency due to induced force as a function of induced force parameter for RWF C.
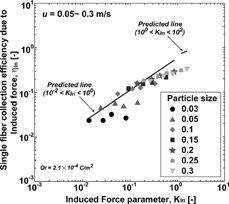
The charge density of RWF, which is estimated from the penetrations of uncharged particles through RWF, is used to calculate the Coulombic force parameter, K C, and the single-fiber efficiencies due to Coulombic force obtained by Equation (Equation11) are plotted against K C in Figures and , respectively, for RWFs (A and C). In these figures, the collection efficiencies due to Coulombic force are somewhat low compared to the predicted efficiency. There are several possible reasons for the discrepancy between the predicted lines and the experimental data. The first reason is that the penetrations, P CInM, through RWFs (A and C) for singly charged particles are smaller than P = 1 × 10− 6, which is close to the detection limit of the present experimental setup. Accordingly, the penetration data for singly charged particles have a larger error compared to those of uncharged particles. As a second reason, CitationLee et al. (2002) suggested that the assumption in additivity between ηC and ηInM might not hold in the region of K In > 1 because the induced force is so strong that it dominates the collection even for singly charged particles. Furthermore, Equations (1)–(5) were tested against the experimental data with only relatively small K In and K C because electret filters with such a high charge density as the present RWF were not available at that time. Therefore, future work is necessary on the interaction between induced force and Coulombic force at a high charge density of fibers. Another reason would be that the manner in which the induced and Coulombic forces exert on particles in RWF is different from that in electret filters. Detail trajectory calculation is necessary to clarify the collection mechanisms in RWF, where particles are captured by the charged particles adhered on fibers.
CONCLUSIONS
Through the measurements of initial collection performance of a newly developed RWF and the estimation of the charge density, the following conclusions are obtained:
1. | The initial penetrations through RWFs studied in the present work are smaller than P = 1 × 10− 5 for particles with diameter larger than 0.3 μ m at filtration velocity of 0.05 m/s with the pressure drop of less than 30 Pa. Therefore, the RWF studied in the present work can achieve high collection efficiency at a low pressure drop, satisfying the requirement of filter media for respirators. | ||||
2. | RWFs containing the same fraction of resin may retain the same charge density on fibers. | ||||
3. | The estimated charge density is Q f = 2.1 × 10− 4 C/m2, which is much higher than those of previously studied electret filter media. |
Future work is necessary to elucidate the electrification mechanisms of RWF and to construct a model for the capture of particles in RWF.
Notes
a Packing densities of filters were obtained from the pressure drops using Davies' equation for drag force.
REFERENCES
- Baumgartner , H. and Loffler , F. 1986 . Particle Collection Performance of Electet Filters in the Particle Size Range 10 nm–10 μ m . J. Aerosol Sci. , 17 : 438 – 445 .
- Brown , R. C. 1981 . Capture of Dust Particles in Filters by Line-Dipole Charged Fibers . J. Aerosol Sci. , 12 : 349 – 356 . [CROSSREF]
- Brown , R. C. , Gray , W. R. , Blackford , D. B. and Bostock , G. J. 1988 . Effect of Industrial Aerosols on the Performance of Electrically Charged Filter Material . Ann. Occupat. Hygiene , 32 : 271 – 294 .
- Davies , C. N. 1952 . The Separation of Airborne Dust and Particles . Proc. Inst. Mech. Eng. Lond. , 131 : 185
- Emi , H. and Kanaoka , C. 1984 . “ Most Penetrating Particle Size in Electret Fiber Filtration ” . In Aerosols , Edited by: Lui , B. Y. H. , Pui , D. Y. H. and Fissan , H. pp. 567 – 572 . Amsterdam : Elsevier .
- Hinds , W. C. 1999 . Aerosol Science , pp. 182 – 205 . New York : John Wiley & Sons .
- Ji , J. H. , Bae , G. N. , Kang , S. H. and Hwang , J. 2003 . Effect of Particle Loading on the Collection Performance of an Electret Cabin Air Filter for Submicron Aerosols . J. Aerosol Sci. , 34 : 1493 – 1503 . [CROSSREF]
- Kimura , K. , Kimura , M. and Shirai , H. 2004 . The Application of the Electrostatic Filter to the Dust Respirator Using the Wool . Sen'i Gakkaishi , 60 : 158 – 161 .
- Kirsch , A. A. and Fuchs , N. A. 1968 . Studies of Fibrous Aerosol Filters-III Diffusional Deposition of Aerosols in Fibrous Filters . Ann. Occup. Hyg. , 11 : 299 – 304 . [PUBMED] [INFOTRIEVE]
- Lee , M. H. , Otani , Y. , Namiki , N. and Emi , H. 2002 . Prediction of Collection Efficiency of High-Performance Electret Filters . J. Chem. Eng. Japan , 35 : 57 – 62 . [CROSSREF]
- Leonard , W. B. and Alan , D. R. 1998 . Aerosol Loading Performance of Electret Filter Media . Am. Ind. Hyg. Assoc. J. , 59 : 532 – 539 .
- National Institute for Occupational Safety and Health (NIOSH) . 1995 . Particulate Filter Penetraion Procedure to Test Negative Pressure Respirators against Liquid Particulates Procedure APRS-STP-0051-00
- Otani , Y. , Emi , H. and Mori , J. 1993 . Initial Collection Efficiency of Electret Filter and Its Durability for Solid and Liquid Particles . J. Chem. Eng. Japan , 11 : 207 – 214 .
- Pich , J. , Emi , H. and Kanaoka , C. 1987 . Coulombic Deposition Mechanism in Electret Filters . J. Aerosol Sci. , 18 : 29 – 35 . [CSA] [CROSSREF]
- Romay , F. J. , Liu , B. Y. H. and Chae , S. J. 1998 . Experimental Study of Electrostatic Capture Mechanisms in Commercial Electret Filters . Aerosol Sci. Technol. , 28 : 224 – 234 .
- Romay , F. J. , Liu , B. Y. H. and Chae , S. J. 1999 . Charge Density Measurement of Electret Filters Using Alpha-Ray Ionizing Radiation . Filtration & Separation , 36 : 51 – 56 . [CSA] [CROSSREF]
- Tanthapanichakoon , W. , Maneeintr , K. , Charinpanitkul , T. and Kanaoka , C. 2003 . Estimation of Collection Efficiency Enhancement Factor for an Electret Fiber with Dust Load . J. Aerosol Sci. , 34 : 1505 – 1522 . [CROSSREF]
- Walsh , D. C. and Stenhouse , J. I. T. 1997a . Clogging of a Electrically Active Fibrous Filter Material: Experimental Results and Two-Dimensional Simulations . Powder Technol. , 93 : 63 – 75 . [CROSSREF]
- Walsh , D. C. and Stenhouse , J. I. T. 1997b . The Effect of Particle Size, Charge, and Composition on the Loading Characteristics of an Electrically Active Fibrous Filter Materal . J. Aerosol Sci. , 28 : 307 – 321 . [CSA] [CROSSREF]