ABSTRACT
Climate change provides new challenges to Nordic forestry. Use of non-native species, such as Douglas fir, can be a means of mitigating the effects of climate change and meeting the growing demand for biomass. When Douglas fir is grown in Sweden, it is several degrees of latitude north of its normal range, which can affect timing of growth cessation, hardening of seedlings, and seedling storability. The objective of this study was to investigate tolerance to freezing and sub-zero storability of seven Douglas fir provenances and make comparisons with the native Norway spruce. Freezing tolerance and storability were evaluated by measuring the electrolytic leakage of shoots and roots after freezing, complemented with a three-week cultivation test after storage. All provenances of Douglas fir used in this study were able to tolerate frozen storage at −4°C for about four months. Norway spruce and the interior Douglas fir provenance, Three Valley, could be stored earlier in the autumn than the southern coastal Douglas fir provenances. Consequently, it should be possible to store Douglas fir seedlings with the same routines as for Norway spruce.
Introduction
The impact of climate change is substantial (IPCC Citation2014). In boreal countries, like Sweden, forestry and the use of harvested wood can mitigate climate change as forest and wood products are a considerable carbon sink (Werner et al. Citation2010; Lundmark et al. Citation2014). Norway spruce (Picea abies L. Karst) is the most common tree species in Sweden, comprising as much as 42% of the total standing volume (Christensen Citation2014). However, Norway spruce will have difficulties in coping with higher temperatures due to climate change (Spiecker et al. Citation1996). An alternative to Norway spruce on some locations may be the non-native Douglas fir (Pseudotsuga menziesii (Mirb.) Franco). Douglas fir is of interest because of its potentially fast growth (Karlberg Citation1961; Nord-Larsen et al. Citation2009), valuable and usable wood and its specific capacity to adapt to changing environmental conditions (Hermann & Lavender Citation1999; Hansson Citation2014; Isaac-Renton et al. Citation2014). The required quantity of fossil energy for forest management activities (Gonzáles-García et al. Citation2013b) and environmental impact over the life cycle of Douglas fir compared to Norway spruce (Gonzáles-García et al. Citation2013a) are also factors to take into consideration. Douglas fir has been grown in the south of Sweden to a limited extent since the early 1900s (Martinsson & Winsa Citation1986; Lemoine & Wirtén Citation1988). More extensive use has probably been constrained by its known susceptibility to damage by frost, pine weevils, browsing and other pests. Limited access to vital seedlings of suitable provenances has also restricted its more widespread growth (Witte Citation1948; Kardell Citation2013, Wallertz & Malmqvist Citation2013, Wallertz et al. Citation2014).
Spring and autumn frosts are among the most common causes of damage to Douglas fir seedlings, both in Sweden and in their natural range in Canada (Newsome et al. Citation1990; Reich Citation1990; Wallertz et al. Citation2013). However, their susceptibility to frosts differs widely among different provenances (Snäll Citation2000; O’Neill et al. Citation2001; Hansen Citation2007). If Douglas fir are to be used widely in Sweden they will be transferred several degrees of latitude northwards. A combination of environmental factors, for example, photoperiod length, air and soil temperature, have been shown to affect growth cessation, bud formation and frost tolerance (Wareing Citation1956; Ekberg et al. Citation1979; Lavender Citation1981; Dormling Citation1993; Hannerz Citation1999; Hannerz & Westin Citation2000). The thermoperiod is especially important during hardening phase and exposure to low temperatures is needed in order to obtain maximum freezing tolerance (see e.g. Sakai & Larcher Citation1987). The difference in the timing of growth cessation is substantial between coastal and interior Douglas fir, the former having a much later cessation of growth (Rehfeldt Citation1977). In Sweden it is mostly coastal Douglas fir provenances that are used in practical plantations, whereas in trials, both coastal and interior provenances have been tested (Martinsson Citation1990; Wallertz et al. Citation2013).
An essential prerequisite for the successful establishment of Douglas fir is that nursery routines should provide seedlings that are vital for planting. Spring vitality of seedlings is often associated with the conditions the seedlings have experienced during storage (Lindström et al. Citation2014). To reduce the risk of roots being exposed to harmful temperatures due to the lack of protective snow cover on open land, it has become common practice in Swedish nurseries to keep seedlings in frozen storage (−3°C to −5°C) during winter. The seedlings are normally lifted in October–November and put into storage until planting, in the beginning of April until midsummer. Such frozen storage will probably be a necessary tool to preserve vitality in Douglas fir seedlings destined for planting. In British Columbia, Canada, Douglas fir seedlings are usually lifted in December or January and stored frozen at −2°C until planting starts in February (Turner & Mitchell Citation2003).
McKay (Citation1993) concluded that bare-root seedlings of Douglas fir did not perform well after being in cold storage for more than one month due to partial damage to fine roots. In Britain however, satisfactory survival of Douglas fir bare-root seedlings after 16 weeks in cold storage has been reported (Mason & Sharpe Citation1992). In order to cope with storage conditions, seedlings need to be freeze-tolerant when first put into sub-zero storage (Stattin et al. Citation2000; Bigras & Colombo Citation2001). Evaluating storability as a function of shoot damage as measured by electrolytic leakage caused by freezing down to −25°C has worked well for Norway spruce seedlings (Lindström & Håkansson Citation1996; Stattin et al. Citation2012). However, to be sure that the seedlings are ready for storage, roots should also be tested for their tolerance to freezing (Stattin et al. Citation2000). Roots are more sensitive than shoots to freezing (Colombo et al. Citation1995) and freezing tolerance of roots increases with low temperatures in the soil (Stattin & Lindström Citation1999). Under some circumstances, shoots may be ready for storage while roots may still not have become sufficiently tolerant to freezing (Stattin et al. Citation2000). As little is known concerning how different provenances of Douglas fir might cope with the autumn conditions and routines in Nordic nurseries, the three-fold objective of this study was: (1) to investigate freezing tolerance of seven Douglas fir provenances; (2) to evaluate storability in freezing conditions; (3) to compare the Douglas fir data with those of the native Norway spruce.
Materials and methods
Plant material, growing conditions and experimental design
Seven provenances of Douglas fir from British Columbia, Canada, and one Swedish provenance of Norway spruce were raised, first in a green house and then in an open field at Vassbo research station, Sweden, (60° 31′ N, 15° 31′ E), see . Seeds were sown on 8 April 2013 in 90 ml containers (Svepot Air 90, Svenska Skogsplantor AB, Sweden), filled with a peat-based compost (420 F, Kekkilä Oy, Tuusula, Finland). The container units have 40 cavities per tray with 547 pots per square meter. Three weeks after sowing, the seedlings were thinned to one seedling per pot. During germination, the pots were watered with tap water. After approximately three weeks, as the seed coats had dropped, the seedlings were fertilized twice a week with a complete fertilizer (N:P:K, 100:13:65 w/v; Wallco, Sweden), rendering a total nitrogen supply of 3 g m−2 week−1. The seedlings were grown in a greenhouse at an average day/night temperature of 25/20°C until 28 June (). Hereafter the seedlings were moved outdoors. On the 25 September 2013, the seedlings were moved back into the greenhouse (air temperature > 5°C and natural lightning) avoiding sub-zero temperatures. Air temperatures (outside and in the greenhouse) were measured and logged every 5 min, using copper-constantan thermocouples attached to an automated data logger (Campbell Scientific CR 1000, UK). Each of the seven provenances of Douglas fir and the Norway spruce were grown in eight container units, resulting in approximately 320 seedlings per seed origin. However the Douglas fir provenance Bowser Heaman produced only approximately 160 seedlings because of poor germination, resulting in lack of seedlings for testing. The containers were randomly distributed.
Figure 1. Air temperature, daily average, maximum and minimum from 8 May 2013 to 31 January 2014. Temperatures were measured in the greenhouse from 8 May 2013 to 28 June 2013 and at the outdoor holding area from 29 June 2013 to 25 September 2013. Greenhouse temperatures were logged as seedlings were moved indoors 25 September 2013.
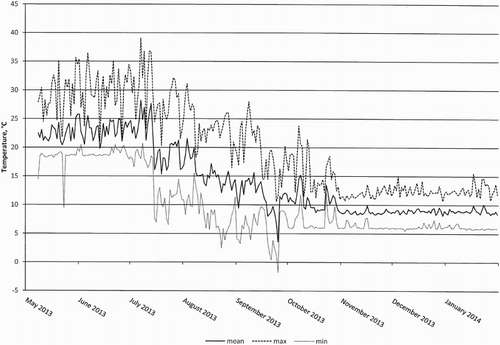
Table 1. The origin of Douglas fir seeds collected in British Columbia, Canada, and Norway spruce seeds collected in Sweden.
Freezing tolerance was evaluated by measuring electrolytic leakage of shoots and roots caused by freezing. Timing of sub-zero storage was assessed by survival and root growth in a post-storage cultivation test. For the measurements of shoot electrolytic leakage after freezing, 18 seedlings per seed origin were lifted randomly on each of 5 occasions, see . The seedlings were divided into six replicates, each containing three seedlings. Three replicates were dedicated for freezing and three for unfrozen control. Freezing of roots were limited to Norway spruce and the Douglas fir provenances Three Valley (interior) and Ladysmith (coastal) on three occasions (). On three occasions (), 20 seedlings from each seed origin, except Bowser Heaman (due to lack of seedlings), were randomly picked for frozen storage. The seedlings were put into four sealed plastic bags each containing five seedlings forming four replicates per seed origin for the frozen storage and forthcoming post-storage regrowth test.
Table 2. Lifting dates of seedlings for determining freezing tolerance (F) by measuring electrolytic leakage (see ). Shoot-freezing tests included all species and provenances, except Bowser Heaman (see ), which were only tested for freezing tolerance on the first three occasions. Root-freezing tests were only conducted with Norway spruce and the Douglas fir provenances Three Valley and Ladysmith. Shoots were frozen to −20°C, roots frozen to −5°C or −10°C. All species and provenances, except Bowser Heaman, were exposed to long-term frozen storage (S) started on three different dates. For freezing tests 18 seedlings per provenance and freezing occasion were used of which 9 were frozen and 9 served as unfrozen control. Each seedling lot that was tested for frozen storage contained 20 seedlings.
Freezing tolerance measurements
Samples of shoots were frozen to −20°C and roots to −5°C and −10°C. The target temperature for the shoots, −20°C, diverges from the standardized SELdiff−25 method where −25°C is used (Lindström et al. Citation2014). The argument for departing from the standardized temperature was that the method was developed for Norway spruce. In other experiments, Sakai and Okada (Citation1971) showed that shoots of Norway spruce tolerated −50°C. From experiments where interior Douglas fir tolerated temperatures down to −40°C, Sakai and Weiser (Citation1973) found coastal Douglas fir to be much more sensitive showing a maximum freezing tolerance of −20°C. If seedlings of Norway spruce tolerate shoot freezing to −25°C, the probability of successful frozen storage is very high (Lindström & Håkansson Citation1996). For the shoot freezing, stem sections of 2 cm were cut 2 cm and 4 cm from the top of the apical shoot. Normally, when testing freezing tolerance to find out storability, the upper 2 cm of the shoot is used (see e.g. Stattin et al. Citation2012; Lindström et al. Citation2014). The reason for not using the upper part of the shoot was a frost event in September (), before the measurements started. Among the coastal Douglas fir provenances, between 69% and 88% of the seedlings showed signs of frost injuries to the upper part of the apical shoot. Corresponding figures for the interior Douglas fir provenances were between 25% and 34% while the Norway spruce did not show any visible injuries. In order to secure the lapse, both upper and lower stem sections were tested for Norway spruce and for the least frost injured Douglas fir provenance, Three Valley.
Shoot samples were prepared for freezing () by first being cleaned in deionized water before being placed into three screw-capped plastic bottles, three shoots in each bottle. The same numbers of samples were prepared for unfrozen seedlings for each seedling lot. The root samples to be frozen (), approximately 4 g in total, consisted of fine roots cut from the tap root of the same seedlings as used for the shoot samples. The roots were washed in cold tap water, rinsed in deionized water and put into screw-capped plastic bottles. As with the shoots, three bottles were prepared for freezing and for unfrozen control with each bottle containing roots from three seedlings. The bottles were then placed in the freezer. The temperature was lowered by 2.5°C per hour to the target temperature, which was held for 1 h. Roots and shoot samples removed from the freezer were placed in pre-chilled boxes filled with styrofoam pellets and placed in cold storage (+ 3°C) for 20 h to ensure slow thawing. After thawing, 40 ml of deionized water were added to each bottle, and the bottles were sealed. Frozen samples and controls were then shaken for 24 h in darkness at a temperature of + 20°C. The electrical conductivity (EC) after freezing to the target temperature (ECfrozen) was measured using a conductivity meter (Model Hach SensIon 5, USA) with built-in temperature compensation. The samples were then autoclaved for 20 min at 1.2 bars pressure and allowed to cool to room temperature. After cooling, the conductivity was measured again (ECtotal) and relative shoot and root electrolytic leakage (SEL and REL) was calculated as (ECfrozen/ECtotal) × 100. The natural leakage was calculated as the ratio between EC-values from unfrozen and boiled samples (ECcontrol/ECtotal) × 100. Shoot and root electrolyte leakage caused by freezing (SELdiff and RELdiff, respectively) were calculated as SEL and REL after freezing subtracted from the natural leakage. SEL−20 and SELcontrol were considered independent within each freezing occasion, as well as REL−5, REL−10 and RELcontrol (see Olsson, Citation2011; Lindström et al. Citation2014).
Cultivation test
The lifted seedlings intended for storage () were placed in sealed plastic bags, placed in cardboard boxes, plastic-coated on the inside and patched together with adhesive tape. The boxes were kept for 19 days in cool storage at + 3°C before being put in a commercial plant freezer at Sör Amsberg nursery in Borlänge. The temperature in the freezer was −4°C. The first two storage batches from October and December () were taken out of the freezer on 5 May 2014, and the third batch, stored in January, was taken out of the freezer on 2 June 2014. This resulted in 167, 124 and 117 days of frozen storage, respectively, for the three batches, before they were tested for regrowth. After thawing at 5°C for one day, the frozen stored seedlings were planted in a completely randomized design with four replicates each containing five seedlings in order to determine root growth capacity (RGC) and survival. The method described fully by Mattson (Citation1986) was followed. The seedlings were planted in a mixture of sand and peat (50% v/v) and cultured for 3 weeks with air/root zone temperature 20–25°C and a photoperiod of 18 h. During daytime seedlings were exposed to natural light in the greenhouse compartment whereas during early morning and night the light source was provided by high-pressure sodium lamps giving a photon flux density of 325 µmol m−2 s−1 at plant level. After the three-week test, the seedlings were carefully lifted and examined for new root growth. All new roots were cut off, dried at 105°C for 24 h and weighed. A seedling was considered dead if no new roots was detected after exhuming and examining the root system.
Statistical analyses
Mean values for shoot and root electrolytic conductance leakage after freezing and root growth after storage were calculated for each seed origin and occasion before the analyses of variance were performed. All variables followed a normal distribution with equal variances. The following model was used for the analyses:
where μ was the general mean, αi was the effect of seedling origin and εij was the experimental error. When significant differences between treatment means occurred, they were separated using least square means adjusted according to Tukey–Kramer. Differences in survival after storage were analyzed with Fischer’s exact test, which fit our experimental setup with four replicates, each containing five seedlings. SAS software (SAS Institute, Cary, NC, USA) was used for all analyses of the acquired data. An α-level of 0.05 was used for all analyses.
Results
Shoot electrolytic leakage
There was a significant difference in shoot-freezing tolerance between Norway spruce and Douglas fir in the first freezing in early October (7 October 2013), see , whereas this was not found between provenances of Douglas fir. All Douglas fir provenances showed very high shoot electrolytic leakage caused by freezing to −20°C. Data from the next freezing occasion (28 October 2013) showed the difference in freezing tolerance between Norway spruce and Douglas fir provenances to be less. Caycuse River, Ladysmith, Bowser Heaman and Larch Hills were significantly separated from Norway spruce but none of the Douglas fir provenances could be separated from each other. In November the freezing tolerance of Bowser Heaman was not only significantly lower compared to Norway spruce, but also to Three Valley and Larch Hills. Data from the December and January occasions showed no significant differences between species and provenances. Natural leakage for all species and provenances varied within 12.5% and 29.5%. The shoot electrolytic leakage caused by freezing to −20°C of the upper stem sections of Norway spruce and the Douglas fir provenance, Three Valley, was not as high as for the lower stem section samples, but showed the same development over time ().
Table 3. Shoot electrolytic leakage at different dates, caused by freezing to −20°C, SELdiff–20 (EL (%) after freezing to –20°C−EL (%) from unfrozen shoots). N = 3.
Root electrolytic leakage
The Douglas fir provenance, Ladysmith, was more sensitive to root freezing than Three Valley. Roots of Norway spruce seedlings were the most tolerant in the October root freezing (28 October 2013), see . In the December freezing (9 December 2013), Norway spruce roots were still significantly more tolerant to freezing than the Douglas fir at both −5°C and −10°C, although there was no significant difference between the Douglas fir provenances on this occasion (). When roots were frozen to −5°C in the third freezing (13 January 2014), no significant differences were obtained. When frozen to −10°C, Norway spruce showed the highest root-freezing tolerance followed by the Three Valley provenance. Ladysmith showed the lowest tolerance when roots were frozen to −10°C.
Figure 2. REL caused by freezing of roots to −5°C, RELdiff–5 (REL (%) after freezing to −5°C−REL (%) from unfrozen roots) and −10°C, RELdiff–10 (REL (%) after freezing to −10°C−REL (%) from unfrozen roots). Roots of the Douglas fir provenances Ladysmith (coastal), Three Valley (interior) and also for the Norway spruce were frozen. Root freezing to −5°C was done on 28 October 2013 (top left), 9 December 2013 (top middle) and 13 January 2014 (top right). Root exposure to −10°C was also performed on 9 December 2013 (bottom middle) and 13 January 2014 (bottom right). N = 3. Mean values indicated with a common letter indicates no significant differences at p = .05 according to Tukey’s test.
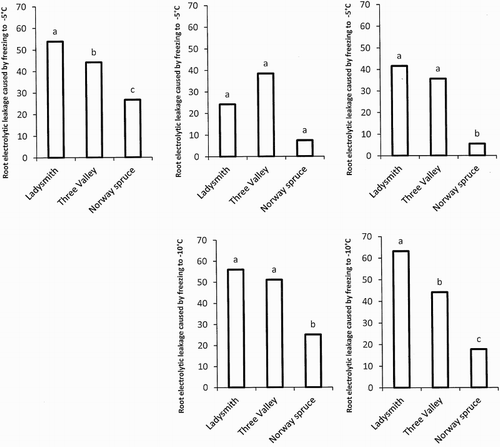
Post-storage performance
According to Fischer’s exact test, survival of Norway spruce, Three Valley and Larch Hills were significantly higher than Ladysmith and Caycuse River (p = .0286) on the first storage occasion. On the following storage occasions no differences in survival after storage between the Douglas fir provenances or Norway spruce were evident (). Regarding root growth, significant differences could only be proved between Norway spruce and Ladysmith at the first storage occasion. On the second storage occasion the northern coastal provenance, Bella Coola, showed vigorous growth during the three weeks of cultivation and was significantly higher than Anstey Arms, one of the interior provenances. No differences in root growth could be seen when comparing provenances of Douglas fir seedlings and Norway spruce ().
Figure 3. Survival (%) (above) and mg root growth per 5 seedlings (below) in 3-week regrowth tests for seedlings stored 28 October 2013 (left), 9 December 2013 (middle) and 13 January 2014 (right). First regrowth test for seedlings stored during 2013 started 5 May 2014 while the regrowth test for seedlings stored 2014 started 2 June 2014. Root growth is given as the mean of four replicates each containing five seedlings. N = 4. Regarding root growth, means with the same letter indicate no significant differences at p = .05 according to Tukey’s test.
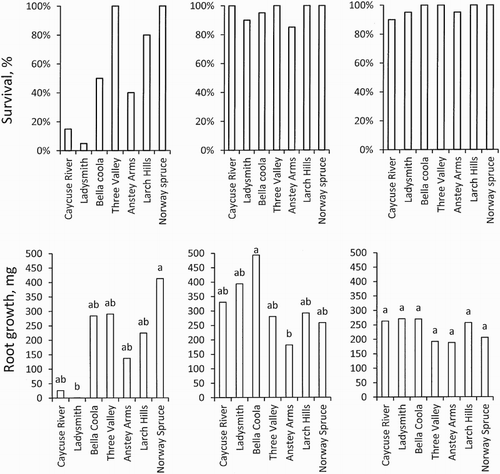
Discussion
In this study Norway spruce reached the target value for safe frozen storage (SELdiff−25 ≤ 5) on the first freezing occasion. This was in accordance with other comparable studies (e.g. Lindström et al. Citation2014). According to Stattin et al. (Citation2000) Norway spruce seedlings can safely be transferred to frozen storage when REL caused by freezing to −5°C differs by less than 7 from that of unfrozen roots. The REL of the Norway spruce and the two Douglas fir provenances tested exceeded this value considerably on the first root-freezing occasion. Nevertheless, all the seedlings of Norway spruce and the interior Douglas fir provenance, Three Valley, survived long-term frozen storage. A probable reason for the seedlings performing well during storage is that the seedlings were cold-stored at + 3°C for three weeks after the measurement of REL caused by freezing to −5°C, which was long enough for them to increase their root-freezing tolerance sufficiently to tolerate the subsequent frozen storage at Sör Amsberg nursery. The main environmental factor enhancing cold acclimation of roots is decreasing soil temperature (Ryyppö et al. Citation1998; Stattin & Lindström Citation1999). As we may encounter warmer autumns due to climate change, there is a risk that the seedlings will develop freezing tolerance even later in the autumn. To put the seedlings in cold storage for a short period prior to frozen storage may be a necessary procedure to avoid root injuries during storage. In spite of the cold storage before being put into frozen storage, just a few of the Ladysmith seedlings survived freezing though.
According to the post-storage cultivation tests, all provenances of Douglas fir used here became storable at −4°C for about four months. The highest survival and root growth among Douglas fir was accomplished when seedlings were lifted for storage at the beginning of December. Apart from Norway spruce and the Three Valley Douglas fir provenance, the earlier storage date resulted in low survival for the Douglas fir provenances. However, the seedlings stored on the first storage occasion were also those that were stored for the longest time; the lower survival rates among these five Douglas fir provenances could therefore be due to a combination of low freezing tolerance and a long storage time, as noted by L’Hirondelle et al. (Citation2006).
Long night treatment is used to induce early growth cessation and bud set, and to speed up dormancy induction and the development of freezing tolerance in order to prepare seedlings either for cold storage or for autumn planting (Grossnickle Citation2000, Turner & Mitchell Citation2003; Jacobs et al. Citation2008; Fløistad & Granhus Citation2013). The use of automatically controlled blackout curtains to prolong the nights has been common practice in Swedish nurseries for about 40 years (Rosvall-Åhnebrink Citation1982), in particular to render southern provenances of Norway spruce ready for storage at an earlier date than would be the case at natural night lengths. Treating Douglas fir with long night treatment might well be an option for improving the possibility of their safe long-term storage in northern nurseries. However, if the length of storage time is crucial for the vitality of the seedlings after storage, blacking-out may not be an appropriate solution. There is a need to evaluate the effects of different long night treatment programs to elucidate the optimum treatments for different provenances. The long night treatment effects on time of bud break the following spring under Scandinavian conditions should also be studied and compared to similar investigations, for example, Jacobs et al. (Citation2008).
According to their survival rates after the long-term frozen storage, most Douglas fir provenances reached storability six weeks later than Norway spruce. The same disparity in time was not so evident in the data concerning the shoot electrolytic leakage. For safe lifting, L’Hirondelle et al. (Citation2006) suggest a shoot electrolytic leakage threshold value of 25–26% for seedlings frozen to −18°C. In that study, however, shoot electrolytic leakage was measured only on frozen samples and was not related to natural leakage, that is, electrolytic leakage of unfrozen samples, as we did in our study. Consequently the level of this recommended threshold might not be applicable. The provenances Bella Coola, Three Valley and Anstey Arm performed better in the cultivation test than the southern coastal provenances Caycuse River and Ladysmith in late October. The interior provenance, Larch Hills, from this occasion is somewhat puzzling: despite having higher shoot electrolytic leakage of about the same value as Caycuse River and Ladysmith, Larch Hills performed just as well in storage as the other interior provenances. This study indicates that the results from shoot electrolytic leakage are somewhat unreliable for predicting storability and post-storage survival in Douglas fir seedlings. To be sure of their readiness for frozen storage, the freezing tolerance of shoots should be complemented with that of root as suggested by Lindström et al. (Citation2014). All samples showed higher levels of natural leakage from shoots than normally found in autumn (see e.g. Lindström & Håkansson Citation1996; Lindström et al. Citation2014), suggesting that the seedlings were already stressed before being frozen. The frost exposure that accidentally occurred on the open land in September could be a reason for disturbances within the cell structure of shoots causing leakage. However, since the results from the freezing performed on the upper (presumably undamaged) stem section did not differ substantially from the results from the freezing of the lower stem section, the results may still be considered reliable in that respect.
In this study the interior Douglas fir provenances showed higher survival and better root growth after storage than did the southern coastal Douglas fir provenances when stored early. The northern coastal Douglas fir provenance, Bella Coola, had an intermediate performance concerning both post-storage survival and root growth. The development of freezing tolerance by roots within species has been shown to be related to the latitude from where the seed originates; for Norway spruce Lindström and Nyström (Citation1987) have shown that northern provenances have a faster rate of increase of freezing tolerance during autumn and early winter. Roots of Sitka spruce (Picea sitchensis (Bong.) Carr.) exhibit similar pattern (McKay Citation1994). However, elevation and continentality are also factors to take into consideration. In a storability test in British Columbia, Canada, frost hardiness increased earlier in interior Douglas fir compared to coastal Douglas fir (L’Hirondelle et al. Citation2006). As for increasing latitudes, genotypes at higher elevations have adapted to the harsh climate by exhibiting early growth cessation and early development of frost hardiness (Heide Citation1974; Dormling & Lundkvist Citation1983; Rehfeldt Citation1989). The high elevation of the interior Douglas fir provenance, Three Valley, may explain why this provenance showed good storage results even for the earliest stored batch. The Three Valley provenance was the only one of the six tested provenances that coped with the frozen storage environment just as good as the Norway spruce. The freezing tolerance of roots is not only important for the seedling storability, but also for how the seedlings cope with soil temperatures in the field during late autumn and winter. Lindström and Troeng (Citation1995) measured the winter temperature in scarified patches, and in organic and mineral mounds with and without snow cover, and noted many hours of temperatures below −5°C in both organic and mineral snowless mounds, and the presence of low temperatures in snowless scarified patches as well. As shown in this study, freezing tolerance of roots is generally lower for Douglas fir seedlings compared with Norway spruce seedlings. Low soil temperature could be a problem during snow-free cold periods for this species, making the provenance selection even more important.
Since all tested provenances of Douglas fir in this study became enough freezing tolerant to survive frozen storage at −4°C for about four months it should be possible to store Douglas fir seedlings using the same routines and equipment as is currently practiced for Norway spruce in Sweden. However, freezing tolerance occurred later for Douglas fir in the autumn than for Norway spruce, indicating a possible risk for the Douglas fir seedlings to suffer from early frost before putting in frozen storage, as well as in the field. The shoot electrolytic leakage method for assessing storability which is generally used for Norway spruce in Sweden would also be suitable for Douglas fir, provided measurements of REL are also undertaken. To obtain a reliable threshold value for storability further studies are necessary.
Acknowledgments
The authors gratefully acknowledge laboratory and greenhouse work provided by Marianne Vemhäll and Elisabeth Wallin. We appreciate the cooperation of Johan Henriksson and Göran Örlander at Södra for donating seeds to this project, and thank Oskar Skogström at Bergvik for providing seedling storage during the experiment. We also thank Owe Martinsson and Harald Säll for their valuable comments and improvements of the manuscript and Jan-Eric Englund for help with the statistics. Special thanks are also due to the English editing service Sees-Editing Ltd. for invaluable help to improve the language of the paper.
Disclosure statement
No potential conflict of interest was reported by the authors.
References
- Bigras FJ, Colombo SJ, editors. 2001. Conifer cold hardiness. Dordrecht: Kluwer Academic Publishers.
- Christiansen L, editor. 2014. Skogsstatistisk årsbok Swedish statistical yearbook of forestry. Jönköping: Swedish Forest Agency. In Swedish with English summary.
- Colombo SJ, Zhao S, Blumwald E. 1995. Frost hardiness gradients in shoots and roots of Picea mariana seedlings. Scan J For Res. 10:32–36. doi: 10.1080/02827589509382864
- Dormling I. 1993. Bud dormancy, frost hardiness, and frost drought in seedlings of Pinus sylvestris and Picea abies. In: Li PH, Christersson L, editors. Advances in plant cold hardiness. Uppsala: CRC Press; p. 285–298.
- Dormling I, Lundkvist K. 1983. Vad bestämmer skogsplantors tillväxt och härdighet i plantskolan? Skogsfakta, Biologi och skogsskötsel nr 8, 6s. [Causes for growth and hardiness of seedlings in nursery practice]. Swedish.
- Ekberg I, Eriksson G, Dormling I. 1979. Photoperiodic reactions in conifer species. Holarctic Ecol. 2:255–263.
- Fløistad IS, Granhus A. 2013. Timing and duration of short-day treatment influence morphology and second bud flush in Picea abies seedlings. Silva Fenn. 47:1–10. doi: 10.14214/sf.1009
- González-García S, Bonnesoeur V, Pizzi A, Feijoo G, Moreira MT. 2013a. Assessing the influence of different forest management systems on the environmental impacts of Douglas-fir roundwood production in France. Sci Total Environ. 461–462:681–692. doi: 10.1016/j.scitotenv.2013.05.069
- González-García S, Krowas I, Becker G, Feijoo G, Moreira MT. 2013b. Cradle-to-gate life cycle inventory and environmental performance of Douglas-fir roundwood production in Germany. J Clean Prod. 54:244–252. doi: 10.1016/j.jclepro.2013.05.012
- Grossnickle SC. 2000. Ecophysiology of Northern spruce species: the performance of planted seedlings. Ottawa: NRC Research Press.
- Hannerz M. 1999. Early testing of growth rythm in Picea abies for prediction of frost damage and growth in the field [doctoral dissertation]. Acta Univesitatis Agriculturae Sueciae. Silvestria 85.
- Hannerz M, Westin J. 2000. Growth cessation and autumn-frost hardiness in one-year-old Picea abies progenies from seed orchards and natural stands. Scand J For Res. 15:309–317. doi: 10.1080/028275800447931
- Hansen JK. 2007. Dyrkningssikker Douglasgran – en evaluering af forsög med douglasgranfrökilder. Skov & Landskab: Köbenhavns Universitet. Danish.
- Hansson J. 2014. Marknadsanalys av douglasgran (Pseudotsuga menziesii Mirb.) Franco) i Sverige, Danmark och norra Tyskland. Market analysis of douglas fir (Pseudotsuga menziesii (Mirb.) Franco) in Sweden, Denmark and northern Germany, SLU Department of Forest Products.
- Heide OM. 1974. Growth and dormancy in Norway spruce ecotypes (Picea abies). I. Interaction of photoperiod and temperature. Physiol Plant. 30:1–12. doi: 10.1111/j.1399-3054.1974.tb04983.x
- Hermann RK, Lavender DP. 1999. Douglas-fir planted forests. New For. 17:53–70. doi: 10.1023/A:1006581028080
- IPCC. 2014. Climate change 2014: synthesis report. In: Core Writing Team, Pachauri RK, Meyer LA, editors. Contribution of working groups I, II and III to the fifth assessment report of the intergovernmental panel on climate change. Geneva: IPCC; p. 1–151.
- Isaac-Renton MG, Roberts DR, Hamann A, Spiecker H. 2014. Douglas-fir plantations in Europe: a retrospective test of assisted migration to address climate change. Global Change Biol. 20:2607–2617. doi: 10.1111/gcb.12604
- Jacobs DF, Davis AS, Wilson BC, Dumroese RK, Goodman RC, Salifu KF. 2008. Short-day treatment alters Douglas-fir seedling dehardening and transplant root proliferation at varying rhizosphere temperatures. Can J For Res. 38:1526–1535. doi: 10.1139/X08-020
- Kardell L. 2013. Åkermarksplanteringar på Tagel, Steninge och Em 1988–2012. Afforestation of abandoned fields at Tagel, Steninge and Em 1988–2012. Uppsala: Dept. of Environmental Forestry, Sveriges lantbruksuniversitet. Rapport / Sveriges lantbruksuniversitet, Institutionen för skoglig landskapsvård. 118. Swedish.
- Karlberg S. 1961. Development and yield of Douglas fir (Pseudotsuga taxifolia (Poir.) Britt.) and Sitka spruce (Picea sitchensis (Bong.) Carr.) in southern Scandinavia and on the Pacific Coast. Bulletin of the Royal School of Forestry. 34. Stockholm.
- Lavender DP. 1981. Environment and shoot growth of woody plants. Oregon State University, School of Forestry, Forest Research Lab. Research paper 45.
- L’Hirondelle SJ, Simpson DG, Binder WD. 2006. Overwinter storability of conifer planting stock: operational testing of fall frost hardiness. New For. 32:307–321. doi: 10.1007/s11056-006-9005-8
- Lemoine K, Wirtén H. 1988. Douglasgran i Sverige – förekomst och produktion. Douglas fir in Sweden – distribution and yield. Umeå: Swedish University of Agricultural Scinences. Department of Forest Management. Swedish.
- Lindström A, Håkansson L. 1996. EC-metoden – ett sätt att bestämma skogsplantors lagringsbarhet [The EC-method – a way to assess storability of forest tree seedlings]. Garpenberg: Swedish University of Agricultural Sciences, Department of Forest Yield Research Report 95. Swedish.
- Lindström A, Nyström C. 1987. Seasonal variation in root hardiness of container-grown Scots pine, Norway spruce, and Lodgepole pine seedlings. Can J For Res. 17:787–793. doi: 10.1139/x87-126
- Lindström A, Stattin E, Gräns D, Wallin E. 2014. Storability measures of Norway spruce and Scots pine seedlings and assessment of post-storage vitality by measuring shoot electrolyte leakage. Scan J For Res. 29:717–724. doi: 10.1080/02827581.2014.977340
- Lindström A, Troeng E. 1995. Temperature variations in planting mounds during winter. Can J For Res. 25:507–515. doi: 10.1139/x95-057
- Lundmark T, Bergh J, Hofer P, Lundström A, Nordin A, Poudel BC, Sathre R, Taverna R, Werner F. 2014. Potential roles of Swedish forestry in the context of climate change mitigation. Forests. 5:557–578. doi: 10.3390/f5040557
- Martinsson O. 1990. Research on Douglas fir in Sweden. Umeå: Swedish University of Agricultural Sciences, Department of Silviculture, Research Report 42.
- Martinsson O, Winsa H. 1986. Främmande trädslag i svenskt skogsbruk [Non-native species in Swedish forestry]. Sveriges Lantbruksuniversitet, Skogsvetenskapliga fakulteten. Swedish university of agricultural sciences, Faculty of Forestry. Rapport. 3. Swedish.
- Mason WL, Sharpe AL. 1992. Some methods of cold storage can seriously affect root growth potential and root moisture content and subsequent forest performance of Sitka spruce and Douglas fir transplants. Forestry. 65:463–472. doi: 10.1093/forestry/65.4.463
- Mattson A. 1986. Seasonal variation in root growth capacity during cultivation of container grown Pinus sylvestris. Scand J For Res. 1:473–482. doi: 10.1080/02827588609382438
- McKay HM. 1993. Tolerance of conifer fine roots to cold storage. Can J For Res. 23:337–342. doi: 10.1139/x93-049
- McKay HM. 1994. Frost hardiness and cold-storage tolerance of the root system of Picea sitchensis, Pseudotsuga menziesii, Larix kaempferi and Pinus sylvestris bare-root seedlings. Scand J For Res. 9:203–213. doi: 10.1080/02827589409382832
- Newsome TA, Sutherland DC, Vyse A. 1990. Establishing Douglas fir plantations in the dry belt of interior British Columbia, In: Interior Douglas-fir, the species and its management, symposium proceedings.
- Nord-Larsen T, Meilby H, Skovsgaard JP. 2009. Site-specific height growth models for six common tree species in Denmark. Scand J For Res. 24:194–204. doi: 10.1080/02827580902795036
- Olsson U. 2011. Statistics for life science. 1st ed. Lund: Studentlitteratur AB.
- O’Neill GA, Adams WT, Aitken SN. 2001. Quantitative genetics of spring and fall cold hardiness in seedlings from two Oregon populations of coastal Douglas-fir. For Ecol Manage. 149:305–318. doi: 10.1016/S0378-1127(00)00564-8
- Rehfeldt G. 1977. Growth and cold hardiness of intervarietal hybrids of Douglas-fir. Theor Appl Genet. 50:3–15. doi: 10.1007/BF00273790
- Rehfeldt GE. 1989. Ecological adaptions in Douglas-fir (Pseudotsuga menziesii var. glauca): a synthesis. For Ecol Manage. 28:203–215. doi: 10.1016/0378-1127(89)90004-2
- Reich RW. 1990. Causes of dieback of Douglas-fir in the interior of B.C. Department of Forestry. Vancouver: The University of British Columbia.
- Rosvall-Åhnebrink G. 1982. Practical application of dormancy induction techniques to greenhouse-grown conifers in Sweden. In: Scarratt JB, Glerum C, Plexman CA, editors. Proceedings, Canadian containerised tree seedling symposium. Canadian Forestry Service. COJFRC symposium proceedings. O-P-10; p. 163–170.
- Ryyppö A, Repo T, Vapaavuori E. 1998. Development of freezing tolerance in roots and shoots of Scots pine seedlings at nonfreezing temperatures. Can J For Res. 28:557–565. doi: 10.1139/x98-022
- Sakai A, Larcher W. 1987. Frost survival of plants. Responses and adaption to freezing stress. Ecological studies 62. Heidelberg: Springer-Verlag; p. 321.
- Sakai A, Okada S. 1971. Freezing resistance of conifers. Silvae Genet. 20:91–97.
- Sakai A, Weiser CJ. 1973. Freezing resistance of trees in North America with reference to tree regions. Ecology. 54:118–126. doi: 10.2307/1934380
- Snäll K. 2000. Frosttolerans hos douglasgran av olika ursprung och tillväxttyp [Frost tolerance of Douglas fir from different origins]. Examensarbete i matematisk statistik. Uppsala: Uppsala University, Department of Mathematics. Swedish.
- Spiecker H, Mielikäinen K, Köhl M, Skovsgaard J, editors. 1996. Growth trends in European forests. European Forest Institute Research Report. 5. Berlin: Springer.
- Stattin E, Hellqvist C, Lindström A. 2000. Storability and root freezing tolerance of Norway spruce seedlings. Can J For Res. 30:964–970. doi: 10.1139/cjfr-30-6-964
- Stattin E, Lindström A. 1999. Influence of soil temperature on root freezing tolerance of Scots pine (Pinus sylvestris L.) seedlings. Plant Soil. 217:173–181. doi: 10.1023/A:1004676514537
- Stattin E, Verhoef N, Balk P, van Wordragen M, Lindström A. 2012. Development of a molecular test to determine the vitality status of Norway spruce (Picea abies) seedlings during frozen storage. New For. 43:665–678. doi: 10.1007/s11056-012-9320-1
- Turner J, Mitchell SJ. 2003. The effect of short day treatments on containerized Douglas-fir morphology, physiology and phenology. New For. 26:279–295. doi: 10.1023/A:1024406704381
- Wallertz K, Frisk J, Johansson U, Örlander G. 2013. Odlingstester och proveniensförsök med douglasgran i Södra Sverige. Dokumentation av etablering och tidig utveckling för planteringar 2009 och 2010. Swedish University of Agricultural Sciences. Enheten för skoglig fältforskning. Report 6. Asa. In Swedish with English summary.
- Wallertz K, Malmqvist C. 2013. The effect of mechanical site preparation methods on the establishment of Norway spruce (Picea Abies (L.) Karst.) and Douglas fir (Pseudotsuga Menziesii (Mirb.) Franco) in southern Sweden. Forestry. 86:71–78. doi: 10.1093/forestry/cps065
- Wallertz K, Nordenhem H, Nordlander G. 2014. Damage by the pine weevil Hylobius abietis to seedlings of two native and five introduced tree species in Sweden. Silva Fenn. 48:1–14. doi: 10.14214/sf.1188
- Wareing PF. 1956. Photoperiodism in woody plants. Ann Rev Plant Physiol. 7:191–214. doi: 10.1146/annurev.pp.07.060156.001203
- Werner F, Taverna R, Hofer P, Thürig E, Kaufmann E. 2010. National and global greenhouse gas dynamics of different forest management and wood use scenarios: a model-based assessment. Environ Sci Policy. 13:72–85. doi: 10.1016/j.envsci.2009.10.004
- Witte A. 1948. Skadegörelse på Douglas-gran. Svenska Skogsvårdsföreningens Tidskrift. 46:10–18. Swedish.