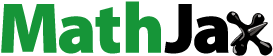
ABSTRACT
Autumn sown small seedlings for later transplanting into large containers have been introduced in Swedish forest tree nurseries. Containerized transplants of Norway spruce (Picea abies (L.) Karst.) from three Swedish nurseries were frozen stored during the autumn of 2014 to find out storability and post-storage vitality. Seedling storability was determined by measuring electrolyte leakage after freezing shoots to −25°C (SE L diff−25), by measurements of dry matter content (DMC) of seedling shoots and by the commercial molecular test ColdNSure™. Vitality of seedlings after storage was determined by measuring the leakage of electrolytes from shoots (SEL), and seedlings were also tested in regrowth tests. All three methods for storability assessment gave similar predictions, except in one case where DMC showed “not storable” for successfully stored seedlings. Our results indicated that young transplants can be successfully short term stored before reaching the target levels for safe long-term storage of conventional seedlings. Early storage of young transplants resulted in low post-storage survival and vitality expressed as root growth capacity and shoot electrolyte leakage (SEL). A prolonged duration in storage generally resulted in lower survival as well as lower root growth capacity and higher levels of SEL, especially for seedlings stored at earlier dates.
Introduction
Planting is the dominating method for reforestation after commercial felling in Northern Europe (Nilsson et al. Citation2010). Therefore, the regeneration of forest stands is largely dependent on production and delivery of large quantities of seedlings from tree nurseries. In Sweden, the mean annual area of final felling in a recent 5-year period (seasons 2011/2012–2015/2016) was approximately 196,000 ha, and 80% of this area were regenerated by planting seedlings of mainly Norway spruce (Picea abies (L.) Karst.) and Scots pine (Pinus sylvestris L.) (Anonymous Citation2017).
In conventional Scandinavian forest tree nurseries, seeds are sown (March–August) in 25–120 ml containers with densities of 300–1600 seedlings per m−2, cultivated in greenhouses for 10–12 weeks, and thereafter transferred outdoors for further growth (Nilsson et al. Citation2010; Mattsson Citation2016). Even though autumn planting is practiced, a majority of the seedlings are put in frozen storage and planted during the following spring (Wennström et al. Citation2016). By using climate-controlled storage facilities, the risks of causing damages to seedlings are minimized compared to outdoor storage (Colombo et al. Citation2001).
The techniques for the production of conifer transplants (i.e. young small seedlings intended for transplanting to outdoor beds or to larger containers) are well known in bare root production (see, e.g. Duryea and Landis Citation1984). In Sweden, small transplants have recently been introduced in containerized nurseries to facilitate more efficient use of greenhouses and storage environments and to save energy (Mattsson et al. Citation2010; Mattsson Citation2016). In 2017, approximately 42 million small transplants were produced in Swedish nurseries (A Lindström personal communication, 1 December 2017). Seeds are sown in small germination containers (3.5–13.0 cm3) (Ersson Citation2015) with densities of 1800–3500 seedlings per m−2 and placed in conventional greenhouses equipped with artificial light (Riikonen Citation2016) or in climate chambers (Mattsson et al. Citation2010; Mattsson Citation2016). Sowing is commonly performed from summer until early autumn and seedlings are cold stored (at approximately +2°C) or frozen stored (at approximately −3°C) from late autumn until spring, thereafter transplanted into larger containers and then transferred outdoors for further growth. Due to recent developments in automation, there are now technical solutions available for mechanized transplanting of seedlings in the containerized nursery business (Ersson Citation2015; Mattsson Citation2016). Another option is to exclude the transplanting procedure after storage and plant the small seedlings directly in the field (Lindström et al. Citation2005; Danielsson et al. Citation2008).
Sowing performed late in the season (i.e. in late summer or early autumn) may lead to delayed dormancy induction as well as slower development of freezing tolerance (Lindström and Nyström Citation1987). Lindström and Stattin (Citation2010a, Citation2010b) found that late sown seedlings seem to require additional time to develop storability compared to seedlings sown early in the season based on survival after frozen storage.
Methods based on determination of dry matter content (DMC) of shoots (Rosvall-Åhnebrink Citation1985; Calmé et al. Citation1993) and freezing tolerance expressed as shoot electrolyte leakage (SEL) after freezing (Colombo Citation1997; Brönnum Citation2005; L’Hirondelle et al. Citation2006; Lindström et al. Citation2014) are currently available to measure storability of conventional seedlings. In addition, genes involved in the development of freezing tolerance have been identified (Joosen et al. Citation2006; Balk et al. Citation2007; Balk et al. Citation2008), and a molecular test (ColdNSureTM) is available for assessment of seedling storability (Stattin et al. Citation2012). The standard threshold values required for safe storage when using the DMC, SEL and gene activity methods are all based on studies performed on containerized pine or spruce seedlings grown for one whole season, or one and a half season. Root growth capacity (RGC) tests (Mattsson Citation1986, Citation1991) can be used to assess seedling root growth ability after frozen storage and post-storage vitality. The RGC method is time-consuming (Lindström et al. Citation2014), but gives a reliable prediction of seedling vitality and field establishment (Mattsson Citation1986, Citation1991). Another way for determining post-storage vitality is based on measurements of the natural electrolyte leakage from roots (McKay Citation1992), REL (root electrolyte leakage) and shoots (Lindström et al. Citation2014), SEL. For Norway spruce, Lindström et al. (Citation2014) found that seedlings with an SEL-value of 0–5% showed good vitality and reached high survival rates compared to seedlings with an SEL-value >10%. The methods for determination of storability as well as post-storage vitality after frozen storage have been developed for the stock types normally used in Swedish forest nurseries, i.e. 1–1.5-year-old seedlings.
It is well known that cold acclimation of, e.g. conifers is a complex physiological process (Levitt Citation1980) that includes several time-consuming developmental stages resulting in a final ability to withstand intercellular freezing during the winter period. Compared to cold acclimation during the autumn, deacclimation in spring is however a much shorter process (Levitt Citation1980; for references see Bigras et al. Citation2001). Today there is an increased interest in growing early autumn sown transplants for containerized production. As these seedlings have a short time for growth and maturation compared with the early sown commonly used stock types, it is important to evaluate the standard methods for determining storability and post-storage vitality.
Materials and methods
Seedling material
Three batches of containerized young transplants of Norway spruce of three different origins () were sown in 2014 and cultivated at three Swedish forest nurseries (). Each nursery used different containers filled with different substrates ().
Table 1. Batch number, seed orchard, origin latitude, location of nurseries, sowing date, name and manufacturer of container types, type of substrate, manufacturer of substrate, information about supplemental light and short-day treatment for each batch of Norway spruce.
All three batches were fertilized with complete mineral solutions dissolved in the irrigation water, based on measurements of the leachate conductivity (target value 0.8–1.0 mS cm−1). For Batch 1, no supplemental light was used in the greenhouse. In order to keep seedlings growing, and not reach growth cessation too early, the day length was extended for seedlings in Batch 2 by supplemental light between 22:00 and 24:00 and between 04:00 and 06:00. The supplemental light regime ended on 6 October. For Batch 3 no supplemental light was used in the greenhouse and from the middle of September the day length was shortened to 8 h by using black curtains covering the seedlings in order to stimulate dormancy development (Dormling Citation1973; Heide Citation1974; Dormling Citation1993: Fløistad and Granhus Citation2013; Wallin et al. Citation2017).
During the autumn 2014, each nursery sent about 200 seedlings (split into four replicates) at four occasions (16 September, 6 October, 27 October, and 17 November) to the research station in Vassbo. From each replicate, 37 seedlings were randomly sampled (in total 148 seedlings).
Determination of storability
Upon arrival, three methods were used in order to assess seedling storability. The methods used were freezing tests, determination of dry matter content (DMC) and a molecular test (the ColdNSure™ test). The freezing tests were performed according to a standardized protocol (Lindström and Håkansson Citation1996; Lindström et al. Citation2014). Storability and freezing tolerance was evaluated by freezing shoots to −25°C in a programmable freezer. The freezing tests were performed on eight seedlings from each of the four replicates, for each storage occasion (), to determine the development of storability of each batch during the period 16 September–17 November. The upper 2 cm of the shoots were cut off, cleaned in deionized water and put into plastic bottles, one bottle per replicate containing four shoots for freezing and one bottle per replicate containing four shoots for unfrozen control. The shoots in bottles intended for freezing were slowly frozen (2.5°C h−1) to −25°C. These samples were kept at the target temperature for one hour before they were slowly thawed (2.5°C h−1) to +5.0°C. The total length of the freezing test procedure was approximately 28 h. Then 40 ml of deionized water was poured into the bottles containing shoots previously frozen as well as in bottles with unfrozen shoots. The unfrozen shoots were stored in a cooler (at +5°C) until the freezing test procedure had ended. Thereafter the samples were put in a shaker in a dark room at +20°C for 24 h. The electrical conductivity (EC) was measured in each bottle using a conductivity meter (Hach SensIon 5) with built-in temperature compensation. All samples were boiled in an autoclave at 1.2 bar for 10 min to release the total amount of electrolytes within the shoots. The relative shoot electrolyte leakage after freezing to −25°C (SEL−25) was calculated as the ratio between the measured electrolyte leakage from shoots after freezing to −25°C and the total amount of electrolytes from shoots measured after boiling. The natural leakage from cells can be explained as the exchange of ions through the outer cell membrane during cell metabolism, and the natural leakage (SELcontrol) was calculated as the ratio between EC-values from unfrozen (control) shoots and the total amount of electrolytes from non-frozen shoots after boiling. The natural leakage (SELcontrol) from unfrozen (control) seedlings was then subtracted from the leakage of shoots frozen to −25°C (SEL−25) in order to isolate the effect of freezing:(1)
(1)
Table 2. Storability estimates using the SELdiff−25 method (shoot electrolyte leakage following freezing shoots to −25°C subtracted by leakage from unfrozen controls), DMC (%), and the ColdNSure™ method based on the activity of freezing tolerance-related genes at the time of storage.
Assessment of storability was also performed by measuring DMC (Rosvall-Åhnebrink Citation1985) of samples consisting of shoots from 20 seedlings in four replicates for each storage occasion. The upper 2 cm of the shoots were cut off and weighed (Weightfresh) and then dried at 105°C for 20–24 h. The samples were cooled to room temperature in a desiccator and then weighed again (Weightdry). The DMC was calculated as a percentage:(2)
(2)
Freezing tolerance and storability of the young seedlings was also analyzed by using the commercial test ColdNSure™ (Joosen et al. Citation2006; Balk et al. Citation2007; Balk et al. Citation2008; Stattin et al. Citation2012; Wallin et al. Citation2017). For each storage occasion, two buds from each of the four replicates were collected and combined into one general sample. Bud samples were frozen in liquid nitrogen, stored in a freezer at −80°C and later sent to the NSure laboratory. The procedure used to isolate RNA and synthesize cDNA to determine the expression of different sets of genes responsible for, e.g. development of freezing tolerance is presented in Stattin et al. (Citation2012). The genes measured were CO1, CO4, CO5, CO8 and CO9. Similar to the model presented for Douglas-fir (Pseudotsuga menziesii (Mirb.) Franco) (Balk et al. Citation2008) the corresponding freezing tolerance status was calculated by NSure using a model for Norway spruce and expressed as four different phases: ColdNSure™ Phase 0 = cold sensitive and the indicator profiles match the profiles of lots that are actively growing; no sign of freezing tolerance can be recognized. Phase 1 = developing freezing tolerance; early signs of freezing tolerance development can be recognized. Phase 2 = developing freezing tolerance; approaching full freezing tolerance. Phase 3 = freezing tolerant and storable; the indicator profiles match the profiles of lots that have ceased growth and that are fully tolerant, ready for lifting and storage.
Determination of post-storage vitality
For each of the three batches and four storage occasions, two separate sets of 40 seedlings (10 seedlings per replicate) were collected, packed in sealed plastic bags, and placed in a freezer at −4°C for shorter (uptake 26 January 2015) or longer (uptake 8 April 2015) time in storage. Following freezer storage, seedlings were thawed at +3°C for 24 h and then post-storage vitality was evaluated for each batch, storage occasion and date of uptake by measuring natural SEL using methodology described in Lindström et al. (Citation2014).
In January and April seedlings from all the storage occasions (five seedlings in four replicates) were taken out of frozen storage to be evaluated for RGC (Mattsson Citation1986, Citation1991). Seedlings were planted in a mix of sand and peat (50:50 by volume) and grown in a growth chamber using similar techniques and environmental conditions as described by Mattsson (Citation1986). After three weeks, RGC as well as survival were measured. All new roots that had grown out in the peat and sand mixture were cut off, cleaned, dried at +105°C for 24 h, and weighted after cooling. Seedlings not showing signs of new root growth were classified as dead.
Statistical analysis
Statistical analysis of differences in survival based on RGC tests in January and April for seedlings with different levels of post-storage SEL was performed by using the chi-square test (Zar Citation2010). The Chi-square test was also used when comparing survival rates in the RGC test for seedlings kept in storage for a shorter (uptake in January) and a longer (uptake in April) period. For treatments resulting in 100% survival, differences in root growth between seedlings exposed to shorter and longer periods in storage were tested with the independent samples t-test with equal variances assumed according to Leven’s test for equality of variances, and the analyses were performed using IBM SPSS Statistics version 23. Differences in terms of post-storage SEL (%) between January and April for each storage occasion for all three batches were analyzed using a general linear mixed hierarchical model with a nested design:(3)
(3) where Yijk is the dependent variable (the SEL observation of the kth seedling of the jth replication in ith treatment), µ is the overall mean for SEL, Ti is the fixed effect of the ith treatment (i = 1, 2, … , 8) where the treatments used are the four different storage occasions for shorter (until January) or longer (until April) durations for each batch, Rij is the random effect of the jth unit of replication (the jth container unit) (j = 1, 2, … , 4) within treatment i, from each replication 5 seedlings were measured (k = 1, 2, … , 5), and еijk is the random error ∼NID
. Where ANOVA indicated significant treatment differences (p < 0.05), Tukey’s studentized range test was used for pairwise comparisons of treatment means (α = 0.05) (Minitab 17 Statistical Software 2016). When comparing differences in root growth related to length of storage, root growth data were only used for treatments showing 100% survival in order to fulfill the requirements for using ANOVA.
Results
Storability
For the three batches stored at four different occasions, the SELdiff−25 method, based on standard threshold values for conventional 1-year old seedlings (SELdiff−25 ≤ 4), indicated that storability had not been obtained for any of the seedlings, except for the seedlings from Batch 1 and Batch 3 at the final storage occasion (17 November) (). In one case only (Batch 1, 17 November), seedlings were considered storable according to the DMC method with target levels developed for conventional 1-year-old seedlings (≥35%).
For Batch 1 and Batch 3, the activity of genes followed the known pattern of upregulation of CO1, CO4, CO8, and CO9, and downregulation of CO5 as freezing tolerance increased with later storage indicating improved freezing tolerance (). As for the SELdiff−25 method, the ColdNSure™ test indicated that seedlings within Batch 1 and Batch 3 were storable at the final storage occasion. However, the DMC method indicated that seedlings from Batch 3 still were not ready for storage on 17 November (). The gene expression patterns observed for Batch 2 differed from the other two batches () with slow progression of freezing tolerance, reaching only the earliest stage (ColdNSure™ Phase 1) at the final storage occasion ().
Figure 1. Gene activity profiles of freezing tolerance-related genes CO1, CO4, CO8, CO9 that is known to be upregulated when seedlings become freezing tolerant, and CO5 which is known to be downregulated (Stattin et al. Citation2012), for three different batches (1, 2 and 3) measured at four different storage dates (1 = 16 September, 2 = 6 October, 3 = 27 October, and 4 = 17 November 2014). At each sampling date eight apical buds were collected from each batch and analyzed for gene activity as a general sample.
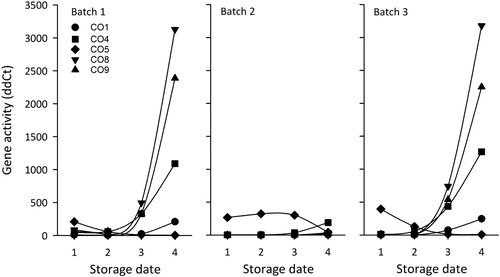
In spite of the storability tests indicating that seedlings were not ready for storage, seedlings from Batch 1 showed high post-storage survival in the regrowth tests for all storage occasions. This was also the case for later stored seedlings (27 October and 17 November) belonging to Batch 3 (). Low survival was recorded for Batch 2, especially for seedlings exposed to longer periods in storage.
Post-storage vitality of seedlings
The general trend was that when survival decreased, leakage from seedling shoots increased. When comparing values of the natural leakage from shoots (SEL) after frozen storage with seedling mortality in the 3-week regrowth test in January, a significant difference was observed between the SEL intervals 0–5% and 5–10% (p < 0.01) (). Also, significant differences in survival were obtained between the SEL intervals 10–20% and 20–30% (p < 0.001) (). In April, significant differences in survival were only obtained between the SEL intervals 10–20% and 20–30% (p < 0.001). The young transplants within SEL interval 10–20% generally showed higher survival rates compared to seedlings within the SEL interval 20–30% (), where only a few seedlings survived. However, when considering individual batches survival rates showed large variation ().
Figure 2. The relation between shoot electrolyte leakage (SEL %) and seedling mortality (%) for each of three batches (1–3) measured after frozen storage. The three different batches were frozen stored at four occasions () and stored until January or April. Shoot electrolyte leakage (SEL %) was measured on 20 seedlings (5 seedlings in 4 replicates) from each batch before planting seedlings in a regrowth test (RGC) starting on 27 January and 9 April, respectively. Post-storage mortality (%) determined 3 weeks after planting was based on 20 seedlings (5 seedlings in 4 replicates) for each batch and storage occasion. For the relation between SEL (%) and mortality (%) logarithmic regressions for all observations resulted in R2 = 0.635.
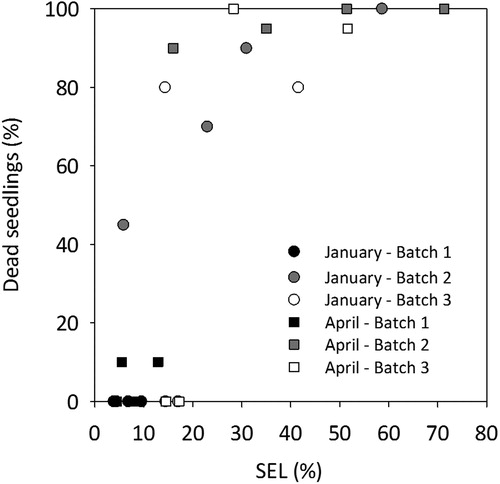
Table 3. Pairwise comparisons of post-storage survival for different SEL (%) intervals.
Clear trends were observed for the relationship between SEL (%) after frozen storage and mortality () and seedling vitality measured as root growth after a 3-week growth test (). Low SEL-values corresponded to high root growth () indicating vital seedlings after frozen storage, whereas at SEL-values >20% there was almost no observed root growth at all.
Figure 3. The relation between SEL (%) and root growth (mg/5 seedlings), for all three batches, all four storage occasions and the two dates for uptake (). Shoot electrolyte leakage (SEL %) was measured on 5 seedlings from 4 replicates, in total 20 seedlings from each batch just before planting seedlings in a RGC test on 27 January and 9 April, respectively. Root growth capacity determined 3 weeks after planting was based on 20 seedlings (5 seedlings from 4 replicates) for each batch and storage occasion. For the relationship between SEL (%) and root growth a logarithmic regression function gave R2 = 0.608.
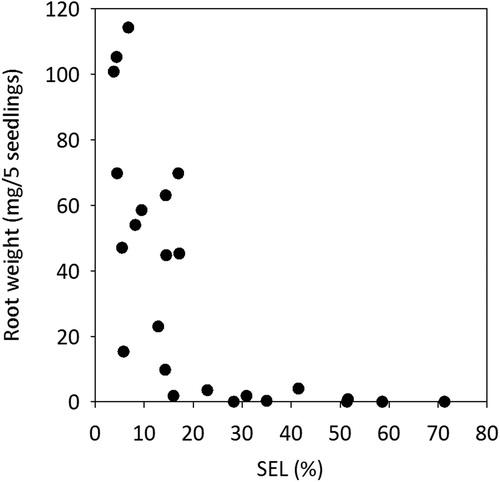
Time in storage
Generally, mean values of post-storage electrolyte leakage (SEL) reached higher levels when the duration of storage was extended from January to April (). Significant differences in SEL between the uptake in January and April were found for Batch 1 for the first three dates of storage, at one storage occasion for Batch 2 (27 October) and at one occasion for Batch 3 (6 October) (). For Batch 1, the two first dates of storage resulted in significantly higher leakage compared to the two later occasions. For Batch 2, only the last date of storage showed lower levels of leakage compared to the early storage occasions. For Batch 3, only seedlings from the first storage occasion resulted in significantly higher leakage from seedlings compared to the three later storage occasions ().
Figure 4. Post-storage shoot electrolyte leakage (SEL %) for all three batches for each date of storage (1 = 16 September, 2 = 6 October, 3 = 27 October, 4 = 17 November), measured after uptake in January (black bars) and April (gray bars). Total number of seedlings in each batch = 20, n = 4 (each replicate contains 5 seedlings). Data were analyzed separately within each batch and for all four storage occasions and the two dates for uptake, and different letters above bars indicate significant differences among treatments at p ≤ 0.05 according to Tukey’s test.
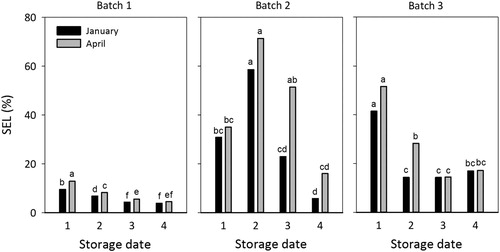
For the different storage occasions and across all three batches the RGC test showed equal or somewhat higher rates of survival when seedlings were sampled in January (70–132 days of storage) compared to April (142–204 days of storage) (). The general trend was that root growth capacity was lowest for earliest stored transplants and that the later uptake (April) resulted in lower root growth capacity compared to the early uptake (January) (). For the treatments in Batch 1 and Batch 3 with 100% survival () significant differences (p < 0.001) were obtained in root growth between uptake in January and April.
Figure 5. Post-storage vitality measured as root growth (dry weight mg/5 seedlings) in 3-week regrowth tests starting on 27 January (black bars) and 9 April (gray bars) for Batch 1, Batch 2 and Batch 3. Measurements based on 20 Norway spruce seedlings (5 seedlings from 4 replicates) for each batch and storage date (1 = 16 September, 2 = 6 October, 3 = 27 October, 4 = 17 November). Vertical lines represent the standard error.
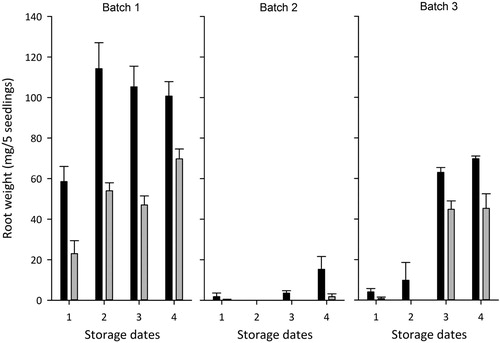
Discussion
Storability tests
According to standard threshold values previously defined for conventional 1–1.5-year-old seedlings, the SELdiff−25 method (Lindström and Håkansson Citation1996; Lindström et al. Citation2014), the DMC method (Rosvall-Åhnebrink Citation1985) and the ColdNSure™ test (Joosen et al. Citation2006; Balk et al. Citation2007; Balk et al. Citation2008; Stattin et al. Citation2012) gave similar predictions of storability status of young transplants for most of the investigated batches and storage occasions. In some cases, the young transplants were successfully stored (100% survival in January and April) in spite of not reaching the standard target levels for storability. We could also notice that an extension of storage duration negatively affected root growth capacity and survival (, ). The time in frozen storage is usually as short as 3–4 months for young transplants due to the transplanting process which normally is performed in February or March. This suggests that the target levels for safe storage should be adjusted for the short period in storage compared to conventional seedlings (normally stored for 6–8 months). Considering the loss in vitality when storage was prolonged, a further extended storage period (e.g. 8 months which is a common length for storage of early sown stock) could be detrimental for young, late sown seedlings.
Besides differences in age, these young late sown seedlings intended for transplanting are raised under different conditions. Light intensity, for example, is usually much lower as these seedlings are sown late in the season when day length is shorter, and there is scarcity of natural light. Also, the daily temperature variation may be unnatural as these young late sown seedlings usually are kept in the greenhouse. Growth cessation, development of dormancy and freezing tolerance normally occurs outdoors for early sown stock, while these processes for young transplants take place in the greenhouse. For successful storage results, it is therefore necessary to optimize the climate control in the greenhouse, which is a challenge and requires detailed knowledge of physiological responses to temperature and light regimes, especially during the hardening phase. As this is complicated, it is necessary to have access to reliable methods to assess storability.
Even though the electrolyte leakage caused by freezing indicated that the seedlings were not storable according to the standard threshold values developed for conventional seedlings, the young transplants in some cases showed surprisingly high root growth capacity and survival rates after frozen storage. This is in accordance with an earlier study by Lindström and Håkansson (Citation1996) who performed storability tests by freezing shoots of young and older spruce seedlings to −20°C. Their results indicated that the younger seedlings were actually storable at higher electrolyte leakage levels after freezing compared to older seedlings.
A possible modification of the SELdiff−25 method for testing young transplants could be to use a higher test temperature (e.g. −20°C or −15°C) than the currently used −25°C or to adjust the target levels for storability. According to , the expression of freezing tolerance-related genes had reached a modest level by 27 October, which corresponds to the ColdNSure™ Phase 2 (). As the outcome of frozen storage was successful in terms of survival rates and root growth, our study indicates that ColdNSure™ Phase 2 could be sufficient to predict safe short time storage of young transplants.
Except for a slight increase in SELdiff−25 for Batch 1 between 16 September and 6 October, the SELdiff−25 values generally decreased with later storage for Batch 1 and Batch 3, indicating that the seedlings from these two batches were storable (conventional seedling threshold values) on 17 November. These relationships between development of freezing tolerance based on SELdiff−25 and dates of storage have earlier been reported for young late sown seedlings by Lindström and Stattin (Citation2010a, Citation2010b) and for conventional seedlings (Stattin et al. Citation2012; Lindström et al. Citation2014). Batch 2 remained at high SELdiff−25 values () indicating that seedlings were not storable at the four storage occasions in this study. The reason for this was probably that this batch had received supplemental light for 2 h in the late evening and 2 h in the early morning until 6 October in order to keep the seedlings in growing conditions and to avoid growth cessation. In the beginning of October, all three storability tests indicated that the seedlings still were in a growing phase. After 5 weeks without supplemental light, the gene test indicated the start of the development of freezing tolerance for Batch 2 (, ). The seedlings from this batch needed about 9 weeks for developing freezing tolerance and were considered storable in the middle of December according to an additional ColdNSure™ test (personal communication, R Anngren, 23 February 2017).
Even though previous studies have shown weak relationships between DMC and storability (Colombo Citation1990; Dormling Citation1990; Lindström et al. Citation2014), the DMC results in this study were consistent with the other two storability tests except for one case (Batch 3 stored on 17 November) where the other methods clearly indicated storability. If the DMC method is to be used for the young transplants, it could be expected that the standard threshold values could be set considerably lower than the values recommended for conventional seedlings (Rosvall-Åhnebrink Citation1985). Unlike the DMC test, the use of freezing tolerance and analysis of the activity of genes indicating freezing tolerance showed the same pattern in predicting storability. This is expected as the ColdNSure™ method is based on results from freezing tests. The expression of the selected freezing tolerance genes when seedlings are able to tolerate −25°C determines the target level (Phase 3) (Stattin et al. Citation2012).
It is well known that there are specific problems related to avoiding the root freezing damage of young seedlings in forest nurseries both in outdoor and frozen storage. As reported by Lindström and Nyström (Citation1987), roots of young seedlings are more sensitive to freezing than root systems of older seedlings. Young transplants are usually grown in containers smaller than a tenth the size of conventional seedling containers. When seedlings are transferred to frozen storage, the small container volume results in rapid freezing and high risks for root damages (Lindström Citation1987) which can cause low post-storage survival (Stattin et al. Citation2000). The larger substrate volume in conventional containers delays the freezing which gives the roots time to better adapt to low temperature (Stattin et al. Citation2000; Lindström and Stattin Citation2010a). One way to increase post-storage survival could be pretreatment of the young transplants in cold storage (+5°C) before transfer to the freezer (−3°C) to give the roots time for hardening. This has previously been shown to be an efficient way of making conventional seedlings storable (Stattin Citation1999; Stattin et al. Citation2000). As no root freezing tests were performed in this study, we cannot tell if limited freezing tolerance of roots has been a cause of the low survival results from early storage occasions. Stattin et al. (Citation2000) found that the electrolyte leakage from roots after freezing to −5°C accurately indicated when Norway spruce seedlings are ready for storage. Our conclusion is that it is important to consider tests of root freezing tolerance for young transplants to ensure safe storage.
Vitality tests
For young transplants to be considered vital after storage and planting, our study indicates that the maximal acceptable electrolyte leakage from shoots (post-storage SEL) can be higher compared to conventional seedlings. For January, the SEL interval 0–5% when seedlings were taken out of storage, corresponded to 100% survival in the regrowth test (, ) compared to 88% in the study of conventional 1-year-old seedlings performed by Lindström et al. (Citation2014). The corresponding values for SEL interval 5–10% in these two studies were 85% and 50%, respectively. Also, for SEL intervals 10–20%, and for 20% or more, the survival rates were higher for the young transplants than for conventional seedlings (Lindström et al. Citation2014). The relation between SEL and survival rates in April followed a similar pattern as for January, but survival was generally lower. Besides high survival for seedlings in the SEL interval 0–5% they also showed high root growth capacity in January and April ().
Generally, the SEL level after storage and seedling mortality () was clearly correlated. This was also the case for the relationship between SEL and root growth (). A correlation between post-storage SEL and the two variables mortality and root growth was also demonstrated for older seedlings in the study by Lindström et al. (Citation2014). In the study with young transplants, we observed a variation between the batches. When adapting a logarithmic function for the relationship between SEL and seedling mortality with pooled data from all batches the R2-value of 0.635 is somewhat lower than for conventional 1-year-old Norway spruce seedlings (R2 = 0.831) as previously reported by Lindström et al. (Citation2014). This could be expected due to the variation between batches in our study. For example, Batch 1, which was 5 weeks older than Batch 2 and 3, generally had lower SEL and lower mortality compared to the other batches for all storage occasions (, ). Our data shows that measuring SEL after storage gives a good prediction of the vitality status of frozen stored young transplants of Norway spruce as previously shown for older seedlings (Lindström et al. Citation2014). Generally, for January and April, there was a statistically significant difference in terms of survival between SEL interval 10–20% and 20–30% (p ≤ 0.001) (). Our study of young transplants indicates that they show a better survival at higher leakage levels compared to older conventional seedlings (Lindström et al. Citation2014). This is also the case for the ability to grow new roots (Figures and ). Lindström et al. (Citation2014) concluded that seedlings with SEL of 0–5% had high survival rate, whereas SEL over 10% indicated low survival and growth capacity after storage. Unlike the older seedlings, the young transplants in this study performed rather well also in the interval 10–20% showing better survival and growth than older seedlings. In most cases, seedlings from all three batches stored on 16 September reached higher levels of SEL compared to seedlings stored later in autumn, especially on 17 November (). A prolonged storage (until April) generally resulted in higher or equivalent levels of SEL for seedlings from all three batches (), indicating lower post-storage vitality. Batch 2 generally showed high leakage from seedlings, especially compared to seedlings from Batch 1 (). High leakage levels for Batch 2 can be explained by the fact that they were still in a growth stage until as late as 6 October by the prolonged artificial light regime. These seedlings were actually still growing at the two earliest storage occasions. On the contrary, seedlings from Batch 3 were long night treated for 16 h starting in the middle of September and ending in November. Probably this treatment had no effect on the development of storability for Batch 3 as the critical night length for bud set for the provenance Saleby is approximately 7–8 h night (Dormling Citation1973), and the natural night lengths in the middle of September at the location of the actual nursery is about 12 h. According to results by Riikonen and Luoranen (Citation2018), keeping the natural night length of approximately 12 h would have been better for seedling post-storage regrowth than to artificially extend the night length to 16 h.
In most cases, the survival rates and root growth for seedlings in Batch 1 and Batch 3 were higher in January than in April (, ). This pattern was also observed for shoot growth (data not presented), which confirms the previous findings that longer periods of frozen storage result in lower vitality of seedlings (Stattin et al. Citation2012). The consistently low storability for Batch 2 resulted in high mortality both in January and April. Seedlings’ carbohydrate reserves are known to gradually deplete during cold conditions (Ritchie Citation1982) which could be a reason for the decline in root () and shoot (data not shown) growth after the longer duration in storage. Luoranen et al. (Citation2012) reported changes in sugar concentrations in seedlings as a result of freezer storage. In addition to this, young transplants have smaller carbohydrate reserves compared to conventional seedlings which could strengthen this effect. Otherwise, rather limited information is available regarding the effects of storage on young transplants. For 9–15-week-old Norway spruce and Scots pine seedlings Lindström and Stattin (Citation2010a) hypothesized that the limited amount of carbohydrates stored in the seedlings were spent through respiration during frozen storage resulting in lower post-storage vitality.
Conclusions
Our findings indicate that when applying current standard threshold values for safe long-term storage using SELdiff−25, DMC and the ColdNSure™ test there are good margins for the young seedlings to successfully endure storage before transplanting. As transplanting normally occurs in early spring, the time in storage for transplants is shorter than for conventional seedlings. Our results indicate that seedlings intended for shorter periods in storage reach a storable status before the previously determined target levels for safe storage are reached. This means that these levels should be modified in order to optimize the time span required to obtain transplants ready for storage. Further research is needed to identify these threshold values to create consistent standards for different stock types. Measurements of shoot electrolyte leakage after storage of young transplants proved to be a reliable method to rapidly forecast seedling vitality as previously shown for older seedlings. Seedling vitality in terms of survival and root growth capacity after planting decreased as the natural leakage from shoots increased, but young seedlings show good vitality at higher levels of leakage than conventional, older seedlings (Lindström et al. Citation2014). Therefore, the level indicating deteriorated seedlings can possibly be set higher than for conventional seedlings. As young transplants are usually grown late in autumn in an artificial environment, it is particularly important for the nurseries to set up routines for storability tests as well as vitality tests, especially for those seedlings intended for longer durations in frozen storage. Without such routines, there are obvious risks for failure with this new way of raising containerized seedlings. Besides a need for further biological evaluation of different methods to raise, store and transplant these young seedlings it is also important to evaluate the economic consequences and risks when applying this complex technique on a large scale.
Acknowledgements
Thanks to Claes Hellqvist for creating the figures and to Claudia von Brömssen for her help with the statistics. Thanks to Marianne Vemhäll for help with laboratory work. We appreciate the constructive comments given by the anonymous reviewers.
Disclosure statement
No potential conflict of interest was reported by the authors.
Additional information
Funding
References
- Anonymous. 2017. Forest statistics 2017. Umeå: Official Statistics of Sweden, Swedish University of Agricultural Sciences.
- Balk PA, Brönnum P, Perks M, Stattin E, van der Geest LJM, van Wordragen MF. 2007. Innovative cold tolerance test for conifer seedlings. USDA Forest Service Proceedings RMRS-P-50. Fort Collins, CO: U.S. Department of Agriculture, Forest Service, Rocky Mountain Research Station. p. 9–14.
- Balk PA, Haase DL, Van Wordragen MF. 2008. Gene activity test determines cold tolerance in Douglas-fir seedlings. USDA Forest Service Proceedings RMRS-P-57. Fort Collins, CO: U.S. Department of Agriculture, Forest Service, Rocky Mountain Research Station. p. 140–148.
- Bigras FJ, Ryyppö A, Lindström A, Stattin E. 2001. Cold acclimation and deacclimation of shoots and roots of conifer seedlings. In: Bigras FJ, Colombo SJ, editors. Conifer cold hardiness. Dordrecht: Kluwer Academic; p. 57–89.
- Brönnum P. 2005. Assessment of seedling storability of Quercus robur and Pinus sylvestris. Scand J For Res. 20:26–35. doi: 10.1080/02827580410019526
- Calmé S, Margolis HA, Bigras FJ. 1993. Influence of cultural practices on the relationship between frost tolerance and water content of containerized black spruce, white spruce, and jack pine seedlings. Can J For Res. 23:503–511. doi: 10.1139/x93-068
- Colombo SJ. 1990. Bud dormancy status, frost hardiness, shoot moisture content, and readiness of black spruce container seedlings for frozen storage. J Am Soc Hortic Sci. 115:302–307. doi: 10.21273/JASHS.115.2.302
- Colombo SJ. 1997. Frost hardening spruce container stock for overwintering in Ontario. New For. 13:449–467. doi: 10.1023/A:1006571300025
- Colombo SJ, Menzies MI, O’Reilly C. 2001. Influence of nursery cultural practices on cold hardiness of coniferous forest tree seedlings. In: Bigras FJ, Colombo SJ, editors. Conifer cold hardiness. Dordrecht: Kluwer; p. 223–252.
- Danielsson M, Kännaste A, Lindström A, Hellqvist C, Stattin E, Långström B, Borg-Karlsson AK. 2008. Mini seedlings of Picea abies are less attacked by Hylobius abietis than conventional ones: is plant chemistry the explanation? Scand J For Res. 23:299–306. doi: 10.1080/02827580802203560
- Dormling I. 1973. Photoperiodic control of growth and growth cessation in Norway spruce seedlings. IUFRO Division 2, Working Party 2.01.4 Growth Processes, International Symposium on Dormancy in Trees; September 5–9, Kórnik, Poland. p. 1–16.
- Dormling I. 1990. Temperatur, ljus och odlingstidens längd påverkar plantornas möjlighet att härdas [The hardening of seedlings is affected by temperature, light, and growth time]. In: Länneholm K, editor. Fytotronen: 25 års forskning i plantodlingens och skogsträdsförädlingens tjänst. Jubileumskonferens februari 28; Sveriges lantbruksuniversitet Stockholm [The Phytotrone: 25 years of research supporting plant production and forest tree improvement. Anniversary Conference February 28; Stockholm: Swedish University of Agricultural Sciences]. Skogsfakta konferens nr 14. Uppsala: Swedish University of Agricultural Sciences. p. 15–19. Swedish.
- Dormling I. 1993. Bud dormancy, frost hardiness, and frost drought in seedlings of Pinus sylvestris and Picea abies. In: Li PH, Christersson L, editors. Advances in plant cold hardiness. Boca Raton, FL: CRC Press; p. 285–298.
- Duryea ML, Landis TD. 1984. Forest nursery manual: Production of bareroot seedlings. The Hague: Dr. Junk Publishers.
- Ersson T. 2015. Review of transplanting and seedling packaging systems in Swedish tree nurseries. Pointe Claire, QC: FPInnovations. Technical Report.
- Fløistad IS, Granhus A. 2013. Timing and duration of short-day treatment influence morphology and second bud flush in Picea abies seedlings. Silva Fenn. 47(3). Article 1009. doi: 10.14214/sf.1009
- Heide OM. 1974. Growth and dormancy in Norway spruce ecotypes (Picea abies). 1. Interaction of photoperiod and temperature. Physiol Plant. 30:1–12. doi: 10.1111/j.1399-3054.1974.tb04983.x
- Joosen RVL, Lammers M, Balk PA, Brönnum P, Konings MCJM, Perks M, Stattin E, Van Wordragen MF, Van der Geest LHM. 2006. Correlating gene expression programs to physiological parameters and environmental conditions during cold acclimation of pine (Pinus sylvestris). Tree Physiol. 26:1297–1313. doi: 10.1093/treephys/26.10.1297
- Levitt J. 1980. Responses of plants to environmental stresses. 2nd ed. New York (NY): Academic Press.
- L’Hirondelle SJ, Simpson DG, Binder WD. 2006. Overwinter storability of conifer planting stock: operational testing of fall frost hardiness. New For. 32:307–321. doi: 10.1007/s11056-006-9005-8
- Lindström A. 1987. Winter storage and root hardiness of containerized conifer seedlings [dissertation]. Garpenberg: Swedish University of Agricultural Sciences, Department of Forest Yield Research.
- Lindström A, Håkansson L. 1996. EC-metoden- ett sätt att bestämma lagringsbarhet [The EC-method- a way to assess storability of forest tree seedlings]. Garpenberg: Swedish University of Agricultural Sciences. Department of Forest Yield Research Report 95. Swedish.
- Lindström A, Hellqvist C, Stattin E. 2005. Mini seedlings – a new forest regeneration system. In Colombo JS, editor. The Thin Green Line – a symposium on the state-of-the-art in reforestation – proceedings (Forest Research Information Paper No. 160). Sault Ste Marie, ON. p. 59–61.
- Lindström A, Nyström C. 1987. Seasonal variation in root hardiness of container-grown Scots pine, Norway spruce and lodgepole pine seedlings. Can J For Res. 17:787–793. doi: 10.1139/x87-126
- Lindström A, Stattin E. 2010a. Vinterlagring av miniplantor i frys – vintern 06/07 och 07/08. Högskolan Dalarna, Akademin Industri och Samhälle, Plantproduktion/Skogsetablering, stencil nr 68. Swedish.
- Lindström A, Stattin E. 2010b. Vinterlagring av miniplantor i frys och kyl – vintern 08/09. Högskolan Dalarna, Akademin Industri och Samhälle, Plantproduktion/Skogsetablering, stencil nr 69. Swedish.
- Lindström A, Stattin E, Gräns D, Wallin E. 2014. Storability measures of Norway spruce and Scots pine seedlings and assessment of post-storage vitality by measuring shoot electrolyte leakage. Scand J For Res. 29:717–724. doi: 10.1080/02827581.2014.977340
- Luoranen J, Riikonen J, Rikala R, Sutinen S. 2012. Frost hardiness, carbohydrates and bud morphology of Picea abies seedlings after different lengths of freezer storage. Scand J For Res. 27:414–419. doi: 10.1080/02827581.2012.666566
- Mattsson A. 1986. Seasonal variation in root growth capacity during cultivation of container grown Pinus sylvestris. Scand J For Res. 1:473–482. doi: 10.1080/02827588609382438
- Mattsson A. 1991. Root growth capacity and field performance of Pinus sylvestris and Picea abies seedlings. Scand J For Res. 6:105–112. doi: 10.1080/02827589109382653
- Mattsson A. 2016. Reforestation challenges in Scandinavia. Reforesta. 1:67–85. doi: 10.21750/REFOR.1.05.5
- Mattsson A, Radoglou K, Kostopoulou P, Bellarosa R, Simeone M C, Schirone B. 2010. Use of innovative technology for the production of high-quality forest regeneration materials. Scand J For Res. 25:3–9. doi: 10.1080/02827581.2010.485825
- McKay HM. 1992. Electrolyte leakage from fine roots of conifer seedlings: a rapid index of plant vitality following cold storage. Can J For Res. 22:1371–1377. doi: 10.1139/x92-182
- Nilsson U, Luoranen J, Kolstrom T, Orlander G, Puttonen P. 2010. Reforestation with planting in Northern Europe. Scand J For Res. 25:283–294. doi: 10.1080/02827581.2010.498384
- Riikonen J. 2016. Pre-cultivation of Scots pine and Norway spruce transplant seedlings under four different light spectra did not affect their field performance. New For. 47:607–619. doi: 10.1007/s11056-016-9533-9
- Riikonen J, Luoranen J. 2018. Use of short-day treatment in the production of Norway spruce mini-plug seedlings under plant factory conditions. Scand J For Res. 33:625–632. doi: 10.1080/02827581.2018.1492011
- Ritchie GA. 1982. Carbohydrate reserves and root growth potential in Douglas-fir seedlings before and after cold storage. Can J For Res. 12:905–912. doi: 10.1139/x82-132
- Rosvall-Åhnebrink G. 1985. Invintring av plantor för höstplantering eller vinterlagring [Preparing seedlings for autumn planting or winter storage]. In: Eriksson A, Zimmerman J, editors. Skogsskötsel i södra Sverige jönköping januari 16–17 [Silviculture in southern Sweden jönköping January 16–17]; Skogsfakta konferens nr 7. Uppsala: Swedish University of Agricultural Sciences; p. 33–37. Swedish.
- Stattin E. 1999. Root freezing tolerance and storability of Scots pine and Norway spruce seedlings [dissertation]. Uppsala: Swedish University of Agricultural Sciences.
- Stattin E, Hellqvist C, Lindström A. 2000. Storability and root freezing tolerance of Norway spruce (Picea abies) seedlings. Can J For Res. 30:964–970. doi: 10.1139/x00-016
- Stattin E, Verhoef N, Balk P, van Wordragen M, Lindström A. 2012. Development of a molecular test to determine the vitality status of Norway spruce (Picea abies) seedlings during frozen storage. New For. 43:665–678. doi: 10.1007/s11056-012-9320-1
- Wallin E, Gräns D, Jacobs DF, Lindström A, Verhoef N. 2017. Short-day photoperiods affect expression of genes related to dormancy and freezing tolerance in Norway spruce seedlings. Ann For Sci. 74:1–14. doi: 10.1007/s13595-017-0655-9
- Wennström U, Hjelm K, Lindström A, Stattin E. 2016. Skogsskötselserien nr 2 Produktion av frö och plantor. Skogsstyrelsen.
- Zar JH. 2010. Biostatistical analysis. 5th ed. Upper Saddle River (NJ): Pearson Prentice Hall.