Abstract
The purpose of this study was to estimate the radiation dose to laterally transposed ovaries from external beam radiotherapy for cervical cancer. Dose measurements were performed in a modified humanoid phantom using a 6 MV photon beam. The dependence of the ovarian dose upon the field size, the distance from the primary irradiation field and the presence of wedges or gonadal shielding was determined. For a tumor dose of 45 Gy, ovarian dose was 0.88–8.51 Gy depending on the field size employed and the location of the transposed ovary in respect to the treatment field. Positioning of 7 cm thick shielding reduced the dose to ovary by less than 19%. The use of wedges increased the ovarian dose by a factor up to 1.5. Accurate radiographic localization of the ovaries allows the use of the presented dosimetric results to obtain a reasonable prediction of the ovarian dose.
Pelvic irradiation using external beam radiotherapy and/or brachytherapy is often recommended to female patients suffering from carcinoma of the uterine cervix. Almost one-half of women with cervical cancer are premenopausal and one-third of them are under the age of 40 years Citation[1]. For these patients of childbearing age, the preservation of ovarian function is of major concern taking into account the high survival rate for cervical cancer Citation[2]. The radiation dose absorbed by the ovaries during cancer treatment has been considered to be the most important predictive factor for ovarian damage Citation[3]. External radiotherapy for cervical cancer may result in direct ovarian exposure with radiation doses exceeding 20 Gy and in permanent gonadal failure Citation[4]. The use of lateral ovarian transposition, in which one or both ovaries are fixed outside the irradiated area, is currently employed to reduce ovarian dose Citation[5–7]. However, the risk of ovarian failure still remains because of the amounts of radiation received by the repositioned ovaries Citation[1], Citation[8].
Publications on the radiation dose to transposed ovaries for individual patients undergoing radiotherapy for carcinoma of the cervix are present in the literature Citation[1], Citation[3], Citation[6], Citation[9–14]. Most of the studies have provided limited data regarding one or more of the following treatment parameters: energy of the megavoltage machine, tumor dose, use of beam modifying absorbers, field arrangement and dimensions of the treatment fields Citation[1], Citation[3], Citation[6], Citation[10–14]. Insufficient information about factors affecting ovarian dose makes the applicability of the previous dosimetric results problematic. Covens et al. Citation[9] have described in detail the parameters of the irradiation technique used. Their ovarian dose estimations, obtained using an indirect method based on the beam profiles of the linear accelerator, refer to a specific position of a transposed ovary irradiated with a single pelvic field size. No attempt was made to study the dependence of the ovarian dose on the extent of the irradiated area and on the distance from the primary treatment field.
In the current study, the radiation dose received by laterally transposed ovaries during radiotherapy for cervical cancer was estimated by performing direct dose measurements on an anthropomorphic phantom with realistic anatomic proportions. Dosimetric data are provided to anticipate the ovarian dose resulting from therapeutic external irradiation for carcinoma of the uterine cervix.
Material and methods
A commercially available Alderson-Rando anthropomorphic phantom (Alderson Research Labs, Stanford, CA) was employed to simulate the real characteristics of female patients undergoing external beam radiotherapy for cervical cancer. The phantom consists of a human skeleton embedded in tissue-equivalent material. It is sliced into 2.5 cm sequential sections numbered 0–35. Each section contains a matrix of 5 mm diameter holes, 3 cm apart.
Reported experience has suggested that the ovaries are transposed in paracolic gutters, lateral to the colon and superior to the iliac crest Citation[5], Citation[7]. For unilateral transposition, the right ovary is selected to be repositioned because it is not hampered by the colon Citation[11]. Dose measurements of this study refer to a transposed ovary situated in the right side of the phantom. Based on the internal anatomy of the Rando phantom, a transposed ovary may be positioned in sections 25 or 26. For each Rando section, a single point located 4.5 cm deep from the anterior phantom surface and 9 cm from the midline of the phantom may correspond to the ovarian position. However, the distance between the ovary and the patient midline may vary considerably among individuals Citation[6]. To obtain a reliable ovarian dose mapping, the sections 25 and 26 were replaced by two 2.5 cm thick sections constructed from Lucite. The external contours of the Lucite sections were the same with those of Rando sections 25 and 26. Each Lucite section was drilled at the level where a transposed right ovary is lying in order to accommodate an ionization chamber. A hole of 4.5 cm length was constructed. The distance separating an ionization chamber placed in the above hole from the phantom midline was 7 cm (). To alter the distance between the chamber and the phantom midline, plugs of 1, 2 and 3 cm length could be inserted into the hole. For both Lucite sections, off-axis dose measurements were carried out at points located 7, 8, 9 and 10 cm from the phantom midline. The above measurement points may correspond to all possible positions of a transposed ovary.
Figure 1. Schematic diagram showing the measurement geometry. The Lucite section was drilled at the right side to allow the placement of an ionization chamber at points corresponding to ovarian positions.
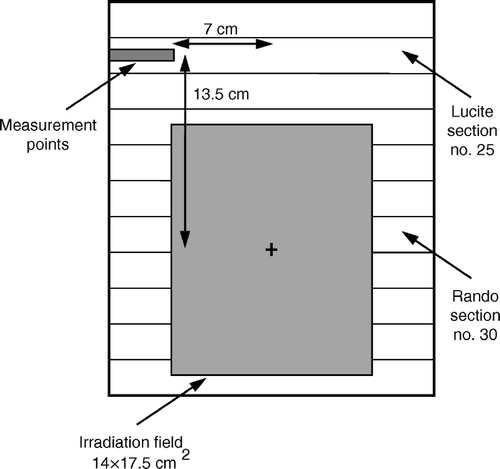
The Rando phantom was irradiated using a four field arrangement consisting of an anteroposterior (AP), a posteroanterior (PA), a left lateral (LL) and a right lateral (RL) treatment fields. The above box technique has been considered as the most common one applied during external beam radiotherapy for cervical cancer Citation[15]. The treatment planning process was performed on the simulator (SIMVIEW NT, Siemens, Germany) of our department by an experienced radiotherapist in treatment of gynecological cancer. Dose measurements were carried out for three different sizes of AP/PA and lateral fields which may correspond to the minimum, medium and maximum field dimensions applied during irradiation for carcinoma of the cervix (). To simulate typical treatment conditions, lead blocks were used to shield healthy tissues during AP/PA and lateral field irradiations. The blocks were designed to cover the tumor site and the regional lymph nodes Citation[15]. The Lucite sections 25 and 26 were located at a distance of 13.5 and 11 cm from the field isocenter, respectively ().
Table I. Dimensions of the anteroposterior (AP), posteroanterior (PA), right lateral (RL) and left lateral (LL) treatment fields.
Additional measurements were performed to evaluate the effect of wedges and gonadal shielding on the ovarian dose. Wedges may be introduced in the lateral beams to secure homogeneity of the delivered radiation dose throughout the treatment volume. The linear accelerator of our department has a single universal wedge of 60°. Ovarian dose was measured using the maximum wedge angle of 60°. Furthermore, a lead block of 7 cm thickness on a shadow tray was used to reduce the ovarian dose. The block was placed over the location of the transposed ovary and it was aligned with the border of the primary field. All the above dose measurements were performed at the four possible ovarian locations in phantom sections 25 and 26 using the medium field size.
Dose measurements were carried out using a 0.125 cm3 ionization chamber (M31002, PTW, Freiburg). Charge was collected by an electrometer (UNIDOS, PTW, Freiburg). The ionization chamber and the electrometer had calibration factors traceable to the National Physical Laboratory. The repeatability of the ionization measurements was found to be better than 1%. Variations in the linear accelerator output were checked and corrected daily before each set of ovarian dose measurements. All phantom exposures were generated with a 6 MV photon beam produced by a linear accelerator (Philips SL 75/5, The Netherlands). To obtain significant readings, each exposure was set to 1000 monitor units.
Results
Ovarian dose measurements
Ovarian dose measurements carried out at distances of 11 and 13.5 cm from the field center are shown in and , respectively. For AP/PA field irradiations, the reduction of the distance from the phantom midline led to an increased proximity of the measurement point to the treatment field and to an increase of the ovarian dose. Regarding the lateral treatment fields, changes in the distance from the phantom midline may indicate changes in the depth of the measurement point from the entrance side of the beam. For phantom exposures with RL fields, the measurement points located at distances of 7, 8, 9 and 10 cm from the phantom midline were at depths of 4.5, 3.5, 2.5 and 1.5 cm, respectively. The corresponding depths for LL field irradiations were 18, 19, 20 and 21 cm, respectively. Our measurements revealed that the ovarian dose increases with depth during both RL and LL field irradiations. This is consistent with previously reported data suggesting the increase of peripheral dose with depth close to the beam edge Citation[16], Citation[17].
Figure 2. Ovarian dose measurements obtained at a distance of 11 cm from the field isocenter for (a) AP, (b) PA, (c) RL and (d) LL field irradiations. Dose values are expressed as a percentage of the given tumor dose.
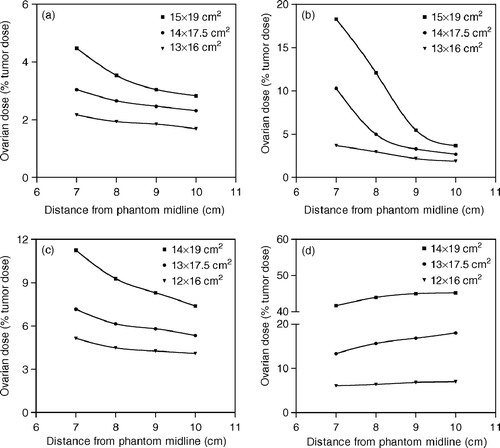
Figure 3. Ovarian dose measurements obtained at a distance of 13.5 cm from the field isocenter for (a) AP, (b) PA, (c) RL and (d) LL field irradiations. Dose values are expressed as a percentage of the given tumor dose.
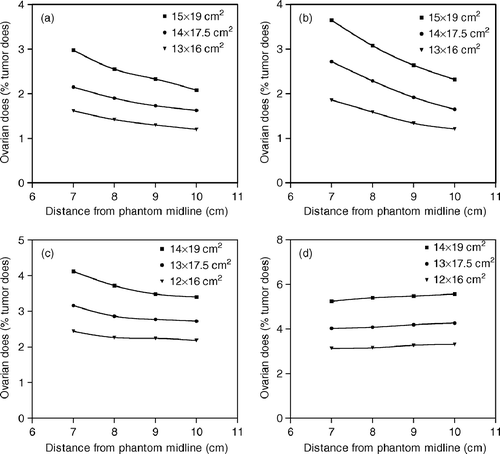
The total ovarian dose attributable to external beam radiotherapy for cervical cancer was calculated by assuming a tumor dose of 45 Gy given by evenly weighted four field treatments Citation[15]. Off-axis measurements performed at a section located 11 cm from the field ioscenter resulted in a total ovarian dose of 1.65–8.51 Gy depending upon the dimensions of the applied treatment fields and the distance from the phantom midline. For a distance of 13.5 cm from the field center, the corresponding radiation dose to a transposed ovary was 0.88–1.83 Gy.
Effect of wedge and gonadal shielding on the ovarian dose
Dose measurements obtained at a distance of 13.5 cm from the field isocenter showed that the presence of gonadal shielding during AP/PA field irradiations may result in a reduction of the ovarian dose by 9 to 26% depending upon the field direction and the distance separating the ovary from the phantom midline. The corresponding dose reduction during lateral field irradiations was 9–19%. Based on the above results, the total radiation dose to a shielded ovary resulting from a four field technique may be reduced by 11–19% compared with the open field arrangement. The use of lead block to shield ovaries located 11 cm from the field center provided a reduction of the gonadal dose by 5–14% and 4–9% for AP/PA and RL field treatments, respectively. The presence of lead shielding had no effect on the ovarian dose resulting from LL field irradiations. The reduction of the total ovarian dose was less than 6%. The introduction of a wedge of 60° into lateral beams increased the ovarian dose by a factor of 1.1–1.5 depending upon the location of the transposed ovary in respect to the phantom midline and the primary irradiation field.
Discussion
The importance of ovarian transposition in young women suffering from carcinoma of the uterine cervix has been well documented Citation[3], Citation[5–7], Citation[9–14]. Ovarian dose estimation prior to irradiation allows the radiotherapists to make reasonable decisions regarding the management of the female patients. The results of this study may be adopted to anticipate the radiation dose to laterally transposed ovaries from external beam radiotherapy for cervical cancer. A detailed graphical data set presents ovarian dose measurements at all possible positions of a transposed ovary using three different dimensions of AP, PA, RL and LL irradiation fields. For individual patients undergoing radiotherapy for carcinoma of the cervix, therapy simulators are currently employed either for the treatment planning process or for the verification of a plan based on computed tomography scans. The simulator may provide an accurate localization of the transposed ovary because of the radio-opaque clips placed on each pole of the ovary at the time of surgical procedure. An AP simulator film of the pelvic region may simultaneously depict the borders of the radiation field and the location of the ovaries. This film may be used to determine the distances separating a transposed ovary from both the field isocenter and the phantom midline taking into account the magnification factor of the radiograph. Knowledge of the above distances allows the use of the presented dosimetric data to predict the ovarian dose with reasonable accuracy.
Simulated phantom measurements showed that the use of wedged lateral beams may result in an ovarian dose up to 1.5 times higher than that obtained with open beam setup. For all phantom exposures, the measurement points were in close proximity to the wedge thick end. The wedge orientation caused the relatively small increase of gonadal dose because the additional scattered radiation produced by the beam modifier was partly counterbalanced by the attenuation of the primary beam in the thick edge of the wedge. The above are in agreement with a previous study of McParland Citation[18] concerning the effect of universal wedges upon peripheral doses.
The use of gonadal shielding reduced the total ovarian dose by less than 19%. The small dose reductions should be mainly attributed to the very close anatomical position of a transposed ovary to the field edge resulting in an increased contribution of the scatter from the phantom to the total ovarian dose. Our dosimetric results indicate that the placement of conventional gonadal shielding on a shadow tray during external beam radiotherapy for cervical cancer might be considered as ineffective. At all positions of a right transposed ovary, the contribution of radiation dose from LL fields to the total ovarian dose was high in comparison with that from AP, PA and RL fields. For unilateral ovarian transposition, the possibility of irradiating carcinoma of the uterine cervix using an AP, a PA and a single lateral treatment field should be investigated since it may result in a significant ovarian dose reduction. The entrance region of the above lateral beam should be at the same side of the transposed ovary.
The preservation of ovarian function is associated with the sensitivity of the ovaries to ionizing radiation. Haie-Meder et al. Citation[3] have reported that almost 85% of women undergoing radiotherapy for a wide variety of pelvic malignancies following lateral ovarian transposition will continue to demonstrate gonadal function for ovarian doses of less than 5 Gy. Other studies have suggested that an ovarian dose lower than 5 Gy is required to induce ovarian damage Citation[10], Citation[12], Citation[14], Citation[19]. Ash Citation[19] has reported that a fractionated dose to ovaries of 4 Gy may cause a 30% incidence of sterility in women aged 15–40 years. Chambers et al. Citation[10] and Van Eijkeren et al. Citation[14] found a steep dose response effect at 3 Gy for induction of early menopause in women undergoing radiotherapy for cervical cancer after lateral ovarian transposition. Husseinzadeh et al. Citation[12] reported that the radiation dose to transposed ovaries should be limited to 3 Gy for ovarian function preservation in young patients irradiated for invasive cervical cancer. To be conservative, a radiation dose of 3 Gy was considered as the threshold value for ovarian damage in this study. Dose measurements obtained at a distance of 11 cm from the field center using the medium or maximum field sizes resulted in ovarian doses similar to those associated with radiation-induced infertility or menopause.
Sources of inaccuracy in ovarian dose estimation using the data presented here are mainly related to the uncertainty of the ionization measurements. Moreover, possible differences either in the depth of a laterally transposed ovary relative to the anterior surface or in the distance between the ovary of an individual patient and the primary irradiation field from those employed during our measurements might introduce unknown errors in the estimated dose.
External beam radiotherapy for cervical cancer may result in an ovarian dose of 0.88–8.51 Gy depending upon the field size used and the location of a transposed ovary in respect to the treatment volume. Therefore, patients undergoing pelvic irradiation after lateral ovarian transposition have the potential of receiving a gonadal dose of more than 3 Gy which might be associated with the induction of sterility or early menopause. The current study provides dosimetric data enabling the reasonable prediction of the ovarian dose and the subsequent assessment of the risk of ovarian damage attributable to external beam radiotherapy for carcinoma of the uterine cervix.
References
- Anderson B, LaPolla J, Turner D, Chapman G, Buller R. Ovarian transposition in cervical cancer. Gynecol Oncol 1993; 49: 206–14
- Talback M, Stenbeck M, Rosen M, Barlow L, Glimelius B. Cancer survival in Sweden 1960–1998 – developments across four decades. Acta Oncol 2003; 42: 637–59
- Haie-Meder C, Mlika-Cabanne N, Michel G, Briot E, Gerbaulet A, Lhomme C, et al. Radiotherapy after ovarian transposition: Ovarian function and fertility preservation. Int J Radiat Oncol Biol Phys 1993; 25: 419–24
- Lushbaugh CC, Casarett GW. The effects of gonadal irradiation in clinical radiation therapy: A review. Cancer 1976; 37: 1111–25
- Belinson JL, Doherty M, McDay JB. A new technique for ovarian transposition. Surg Gynecol Obstet 1984; 159: 157–60
- Hadar H, Loven D, Herskovitz P, Bairey O, Yagoda A, Levani H. An evaluation of lateral and medial transposition of the ovaries out of radiation fields. Cancer 1994; 74: 774–9
- McCall ML, Keaty EC, Thompson JD. Conservation of ovarian tissue in treatment of the carcinoma of the cervix with radical surgery. Am J Obstet Gynecol 1958; 75: 590–600
- Feeney DD, Moore DH, Look KY, Stehman FB, Sutton GP. The fate of the ovaries after radical hysterectomy and ovarian transposition. Gynecol Oncol 1995; 56: 3–7
- Covens AL, Van der Putten HW, Fyles AW, O'Brien PF, Murphy KJ, DePetrillo AD. Laparascopic ovarian transposition. Eur J Gynaec Oncol 1996; 17: 177–82
- Chambers SK, Chambers JT, Kier R, Peschel RE. Sequelae of lateral ovarian transposition in irradiated cervical cancer patients. Int J Radiat Oncol Biol Phys 1991; 20: 1305–8
- Clough KB, Goffinet F, Labib A, Renoleau C, Campana F, De la Rochefondiere A, et al. Laparoscopic unilateral ovarian transposition prior to irradiation. Prospective study of 20 cases. Cancer 1996; 77: 2638–45
- Husseinzadeh N, Van Aken ML, Aron B. Ovarian transposition in young patients with invasive cervical cancer receiving radiation therapy. Int J Gynecol Cancer 1994; 4: 61–5
- Morice P, Castaigne D, Haie-Meder C, Pautier P, El Hassan J, Duvillard P, et al. Laparoscopic ovarian transposition for pelvic malignancies: Indications and functional outcomes. Fertil Steril 1998; 70: 956–60
- Van Eijkeren MA, Van Der Wijk I, El Sharouni SY, Heintz APM. Benefits and side effects of lateral ovarian transposition (LOT) performed during radical hysterectomy and pelvic lymphadenectomy for early stage cervical cancer. Int J Gynecol Cancer 1999; 9: 396–400
- Eifel PJ. The uterine cervix. Radiation oncology: Rationale, technique, results8th ed, JD Cox, KK Ang. Mosby, St Louis 2003; 681–723
- Kase KR, Svensson GK, Wolbarst AB, Marks MA. Measurements of dose from secondary radiation outside a treatment field. Int J Radiat Oncol Biol Phys 1983; 9: 1177–83
- McParland BJ, Fair HI. A method of calculating peripheral dose distributions of photon beams below 10 MV. Med Phys 1992; 19: 283–93
- McParland BJ. Peripheral doses of two linear accelerators employing universal wedges. Br J Radiol 1990; 63: 295–8
- Ash P. The influence of radiation on fertility in man. Br J Radiol 1980; 53: 271–8