Abstract
The management of prostate cancer patients with a significant risk of pelvic lymph node involvement is controversial. Both whole pelvis radiotherapy and dose escalation to the prostate have been linked to improved outcome in such patients, but it is unclear whether conventional whole pelvis doses of only 45–50 Gy are optimal for ultimate nodal control. The purpose of this study is to examine the dosimetric and clinical feasibility of combining prostate dose escalation via hypofractionation with conformal avoidance-based IMRT (H-CAD) dose escalation to the pelvic lymph nodes. One conformal avoidance and one conventional plan were generated for each of eight patients. Conformal avoidance-based IMRT plans were generated that specifically excluded bowel, rectum, and bladder. The prostate and lower seminal vesicles (PTV 70) were planned to receive 70 Gy in 2.5 Gy/fraction while the pelvic lymph nodes (PTV 56) were to concurrently receive 56 Gy in 2 Gy/fraction. The volume of small bowel receiving ≥45 Gy was restricted to 300 ml or less. These conformal avoidance plans were delivered using helical tomotherapy or LINAC-based IMRT with daily imaging localization. All patients received neoadjuvant and concurrent androgen deprivation with a planned total of two years. The conventional, sequential plans created for comparison purposes for all patients consisted of a conventional 4-field pelvic box prescribed to 50.4 Gy (1.8 Gy/fraction) followed by an IMRT boost to the prostate of 25.2 Gy (1.8 Gy/fraction) yielding a final prostate dose of 75.6 Gy. For all plans, the prescription dose was to cover the target structure. Equivalent uniform dose (EUD) analyses were performed on all targets and dose-volume histograms (DVH) were displayed in terms of both physical and normalized total dose (NTD), i.e. dose in 2 Gy fraction equivalents. H-CAD IMRT plans were created for and delivered to all eight patients. Analysis of the H-CAD plans demonstrates prescription dose coverage of >95% of both the PTV 70 (prostate) and PTV 56 (nodes). The EUDs for PTV 70 and PTV 56 were greater than prescription dose for all eight plans. Analysis of bio-effective DVHs demonstrated similar amounts of small bowel receiving ≥45 Gy for H-CAD and sequential plans, in spite of the significantly higher dose to which H-CAD treated the pelvic nodes. The treatment was well tolerated in the eight treated patients in that no grade 2 or higher acute gastrointestinal toxicities were seen. Prostate hypofractionation with concurrent conformal avoidance-based pelvic IMRT for high risk prostate cancer represents an efficient and promising method for achieving dose escalation both of pelvic lymph nodes and the prostate with modest acute toxicity. Unlike a vascular-guided targeting approach, conformal avoidance has the potential advantage of also encompassing at-risk nodes that are not contained within major nodal chains. A phase II trial to more thoroughly examine this treatment approach is currently underway.
Prostate cancer is the most common non-skin cancer in American men, resulting in over 30 000 deaths annually in this country Citation[1]. The optimal treatment approach for localized, prostate cancer remains controversial. Radiation therapy has established a major role in the management of localized disease, a role supported by long term disease control rates that are comparable to radical prostatectomy but commonly achieved with less toxicity Citation[2].
Higher risk prostate cancer patients have a significant risk of regional lymph node metastasis, likely representing a major cause of biochemical failure. However, ultimate treatment outcomes have not shown consistent improvement with whole pelvis radiation. Radiation doses necessary to sterilize small volume disease (1 ml) (>90% probability) in regional lymph nodes in squamous cell carcinoma of the head and neck are on the order of 54–60 Gy, as opposed to the 45–50.4 Gy commonly delivered to prostate adenocarcinoma cases with pelvic radiotherapy Citation[3]. Highly conformal techniques, such as intensity modulated radiation therapy (IMRT), afford the potential to safely increase dose to at risk lymph nodes and may provide better potential for eradicating microscopic lymph node disease in patients with high risk prostate cancer. In this study, we examine hypofractionation of the prostate with concurrent treatment of the pelvis using conformal avoidance as a simple, reproducible method for pelvic IMRT. This novel hypofractionation/conformal avoidance target definition (H-CAD) scheme is described with preliminary acute toxicity results.
Materials and methods
Comprehensive high dose nodal and prostatic radiation therapy was planned and delivered to eight patients, two of whom were status post radical prostatectomy. All patients had biopsy proven prostate cancer. Eligibility requirements mandated that definitive patients have a risk of lymph node metastasis exceeding 15% by standard nomograms Citation[4], Citation[5] and post-operative patients had pre-operative criteria meeting lymph node risk ≥15% and biochemical failure. Staging with bone scan and CT of the abdomen and pelvis was performed to rule out radiographically apparent nodal or distant metastases. Routine blood tests including complete blood count, creatinine, and electrolytes were obtained.
Simulation/planning CT
Patients were not allowed to eat for four hours prior to a planning CT scan. Patients are given five cups of oral contrast (Gastrografin, Bristol-Myers Squibb) two hours prior to simulation along with metoclopramide 10 mg orally. The bladder was filled with 200 ml of contrast diluted with saline following foley catheter placement. In order to localize the targets a pretreatment MVCT is obtained. A rectal displacement balloon was employed both during planning and treatment as previously described Citation[8]. Intravenous contrast was used in all patients with a normal serum creatinine level. A planning CT was obtained with the patient placed in the prone position in a bowel displacement device (bellyboard) Citation[9]. The CT study was acquired with a 2.5 mm slice thickness. Scanning borders extend from the top of the L4 vertebral body to the bottom of the lesser trochanter.
Conformal avoidance target delineation
All contouring was performed on a Pinnacle3 Planning System (Philips Medical Systems, San Milipitas, CA).
Organs-at-risk (OARs)
Normal tissues structures were contoured as follows: Rectum: The rectal wall volume (outer minus inner) was contoured for a total length of 11 cm beginning at approximately the inferior-most portion of the ischial tuberosities and extending to the sigmoid colon (, green). Bladder: The bladder was delineated by auto contouring the contrast within the bladder on the planning CT (200 ml) (, gray). Small bowel: For the purposes of planning, the small bowel region of interest was defined as any small bowel within the peritoneal cavity from the top of L4 superiorly to one slice above the uppermost contour of the rectum inferiorly. Contrast enhancing loops of bowel including intervening fat were contoured as a single structure (, brown). Skin/ring of avoidance: For patients being treated with helical tomotherapy, skin was auto-contoured on all CT slices. These contours were then contracted 5 mm resulting in a 5 mm thick skin structure identified for avoidance. Urethra: The urethra was contoured as visualized by the foley catheter on axial CT slices. Vessels: Pelvic vessels (common, external and internal iliacs) were contoured as visualized by contrast enhancement (, light blue).
Figure 1. Conformal avoidance based target definition. Column on the left shows creation of the 4-field box at different levels in the pelvis (in red). Column on the right shows modifications and subtraction of normal volumes to create the nodal PTV (blue). Bowel is shown in brown, bladder in gray, prostate in red, and rectum in green.
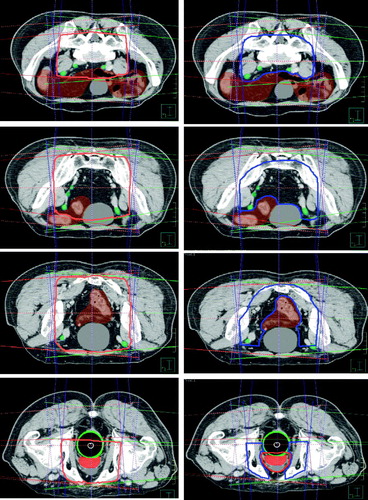
Prostate CTV and PTV 70 (prostate)
The CTV included the entire prostate gland as visualized on CT scan. Seminal vesicles were included in the prostate CTV for at least 75% of the prescription dose if the estimated risk for seminal vesicle involvement was ≥15%. For situations where there was less than 15% risk of seminal vesicle involvement, only the base of the seminal vesicles was included in the GTV. The CTV 70 represented the same volume as the prostate CTV plus a 2 mm margin in all dimensions except at the prostate-rectal interface, for which a zero margin was used. The prostate PTV included the CTV with a margin of 3 mm to account for image guidance uncertainties consistent with previously published reports Citation[6], Citation[10]. Image guidance was employed using either transabdominal ultrasound Citation[6] or megavoltage CT (using Tomotherapy) Citation[10]. Dosimetric margins were chosen to provide adequate coverage of the PTV with no more than 10% dose heterogeneity across the PTV. At least 95% of the prostatic PTV received the prescription dose or higher.
Conformal avoidance PTV 56 (at-risk nodal regions)
A conformal avoidance approach used to define the nodal PTV to be treated to 56 Gy is outlined below: 1. Four field box: A conventional four-field box beam arrangement was placed for the initial volume definition only () and was not used in the actual delivery of H-CAD treatments. The four-field box was designed to cover the pelvic vessels with a 2 cm margin. Generally, the four field arrangement could also be classically defined based on bony anatomy, as long as pelvic vessels were adequately covered. The superior border of the four-field box was set at middle of the L5 vertebral body and the inferior border at the ischial tuberosities. The AP and PA fields defined the lateral extents of the volume. Field edges were placed just lateral to the SI joints and the obturator foramina and otherwise were 2 cm outside the pelvic rim. The femoral heads were shielded on the AP/PA fields. The lateral fields defined the anterior and posterior volume limits. Generally, the posterior border was set behind the bony sacrum as inferiorly as S3 for coverage of presacral nodes. At S3, the posterior border was brought anteriorly to partially shield the rectum. The posterior block was brought no closer that 2 cm from the posterior prostate. The anterior block was defined by a line 1 cm anteriosuperior to an imaginary line from the sacral prominence to the superior pubic symphysis. Following the placement of the four-field box, the intersection of the beams was then visualized on axial CT slices. This intersection was manually contoured (, red contours, left column), forming the initial nodal planning volume. 2. Subtracting organs at risk (OARs) from the four-field box initial volume: To define the raw PTV 56, structures overlapping into the four-field box were subtracted from the initial planning volume. These structures were the PTV 70, bladder, rectum, and small bowel. 3. Editing the PTV 56: Following the subtraction of OARs, PTV 56 was then further trimmed to inside the true pelvis. Other low risk areas (anterior to the bladder) are also subtracted from the final PTV 56 (, blue contours, right column).
Dose prescription
For patients treated definitively, pelvic nodal regions received 56 Gy delivered in 2 Gy/fraction, delivered concurrently while the prostate PTV receives 70 Gy delivered in 2.5 Gy fractions in a total of 28 fractions. In patients treated post-operatively, pelvic nodal regions received 54 Gy delivered in 2.08 Gy/fraction, delivered concurrently while the prostatic fossa PTV received 65 Gy delivered in 2.5 Gy fractions in a total of 26 fractions. The dose constraints placed on the bladder and small bowel are described in , consistent with those specified in Roeske et al. Citation[11] but modified for the current fractionation scheme using standard linear quadratic formulation. Patients were treated five days per week.
Table I. Dosimetric goals*: Iso-effective normal tissue parameters.
Intensity modulated treatment plans
These treatment volumes and regions of conformal avoidance were then transferred to the IMRT module of the treatment planning system (TPS) (either the Tomotherapy TPS or the Pinnacle3 (v. 7.4f) TPS). Both of these treatment planning systems use the convolution superposition method for a full 3D dose calculation.
Linac-based plans were created on the Pinnacle3 (v. 7.4f) TPS IMRT planning module. First, dose objectives for each region of interest were defined. The plans were optimized using a Quasi-Newton gradient-based optimization engine imbedded in the ORBIT Citation[12], Citation[13] framework, which is an iterative method that continually evaluates the overall objective function and the derivative of the objective function with respect to each optimized parameter (for IMRT, this parameter is a beamlet). After optimization and iterative dose computation, treatment delivery is performed with a “Step and Shoot technique” using a Varian 600C/D equipped with a 120-leaf Millennium Multileaf Collimator and our Image Guided Patient Localization System Citation[14].
Tomotherapy plans were generated on the Tomotherapy TPS, a stand alone IMRT planning system. A field width, pitch value and modulation factor were first selected for helical treatment delivery. The selection of these parameters depends on the superior-inferior target length and the shape of the target and the proximity of OARs to the target. Next dose objectives were defined for each region of interest. Once all the planning parameters have been selected, the treatment plan was optimized. Finally, a deliverable treatment plan was created in which leaf open times for each MLC leaf at each projection are corrected for leaf latency and any leaves that are open for times less than the leaf latency time at a particular projection are closed. Because this last step can alter the DVH, the final deliverable plan has to be carefully reviewed.
When using the Varian Linac-based delivery, the length of the pelvic nodal field almost always exceeds the allowed maximal MLC leaf separation of adjacent leaves in the superior-inferior direction. Therefore, using this large-field IMRT approach necessitates the splitting of each IMRT portal into two subfields. This approach requires a total treatment time delivery of about 36 min using a dose rate of 300MU/min. This limitation does not exist with Tomotherapy or other dynamic forms of IMRT, which delivers radiation with a total treatment time of less than 8 minutes.
Treatment delivery
Patients were usually treated prone in a bowel displacement device (bellyboard) Citation[9]. A rectal displacement balloon was used Citation[8] and daily pretreatment prostate and pelvis localization required. Clearly, prostate alignment and the bony anatomy alignment are not necessarily correlated Citation[6]. As pointed out above the prostate is immobilized using a rectal balloon. Furthermore, the prostate represents the primary target, while the CTV56 represents a secondary target since it contains likely microscopic disease. Therefore, prostate localization is most critical and takes precedence over CTV56 alignment should they not be correlated. However, in order to ensure that the CTV56 is adequately localized we have chosen a margin between the CTV56 and PTV56 that is based 95% confidence interval that we have derived form prostate localizations using 3D Ultrasound Citation[7]. Treatments were carried out using Intensity Modulated Radiation Therapy with 6 MV, using either a LINAC or helical tomotherapy.
Sequential (control) plans
Control plans using standard sequential pelvic and prostatic boost fields were generated for all patients. The four field beam arrangement described above was prescribed to a total dose of 50.4 Gy (28 fractions, 1.8 Gy/fraction). A 7-field IMRT boost was then planned for the prostate PTV for an additional 25.2 Gy (14 fractions, 1.8 Gy/fraction) yielding a final tumor dose of 75.6 Gy.
Volume analysis
Volumes of small bowel receiving ≥45 and 50 Gy were calculated for H-CAD and sequential plans for each patient. Because the two plans were compared to each other for each patient, we performed a paired, two-tail, t-test for statistical significance.
Bio-effective DVH analysis and fractionations schedule comparisons
DVH analysis and fractionation schedule comparisons were performed. Because of the significant fraction size difference between the H-CAD IMRT and sequential schedules, bio-effective DVH's were created in which dose and fractionation schedules are reported as normalized total dose (NTD), the equivalent dose if delivered in 2 Gy fractions. NTD is calculated using linear-quadratic formulation Citation[15] as follows:n = number of fractions
d = dose per fraction
nd = total dose
Assumptions:
Prostate: α/β = 1.5 Citation[16], 3.1 Citation[17], or 10
Nodal: α/β = 10
Small bowel/ rectum: α/β = 3
Acute side effects are reported using modified RTOG scoring criteria (GI and GU) Citation[18] and the NCI-CTC v.3.0. (skin).
Results
Plans of eight treated patients are analyzed. The creation of the conformal avoidance derived pelvic nodal PTV for patient 2 is shown in . Dose distributions from the treatment plan of the same patient are shown in and demonstrate excellent prescription isodose coverage of the target volumes. The deliverable IMRT plans were analyzed by calculating equivalent uniform dose (EUD) to the PTVs and the small bowel volume ≥45 Gy (NTD) and were then compared to the control sequential plans. For all plans, the EUD was greater than the prescription dose Citation[19]. In spite of the higher dose to lymph nodes with the H-CAD plans, the volumes of small bowel receiving a ≥45 and 50 Gy appeared to be lower for the H-CAD plans in comparison to the sequential plans (p = 0.024 and 0.006 respectively), as seen in .
Table II. Small bowel dosimetry.
The physical dose DVH from the Tomotherapy TPS for patient 2 shows a deliverable plan with steep curves for PTV 70 and PTV 56 (). A bio-effective DVH for the same patient is also shown (). PTV 70 is plotted on this bio-effective DVH using prostate α/β assumptions of 1.5 and 10 to demonstrate the zone of potential efficacy (in red) of the fractionation regimen used. Dose to the normal structures from the H-CAD plans are compared to the sequential plans on the bio-effective DVH as well .
Figure 4. Bio-effective DVH from H-CAD pelvic IMRT plan for patient 2. Doses are expressed in 2 Gy equivalents. A zone of possible efficacy for this plan based on a range of possible α/β values for prostate cancer is shaded in red.
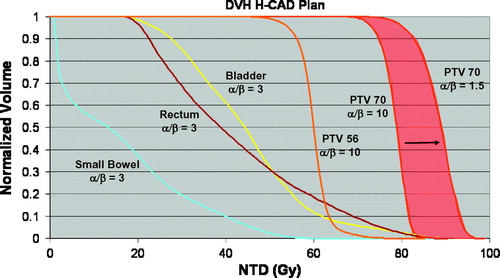
Figure 5. Bio-effective DVH from patient 2 comparing H-CAD plan with sequential plan. Doses are expressed in 2 Gy equivalents.
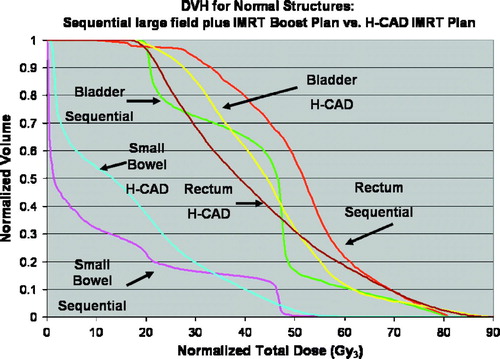
Treatments with H-CAD plans were very well tolerated with minimal acute toxicities for the first eight patients treated (). All patients had at least one month follow-up and no toxicities ≥ grade 3 were noted in these patients. One patient treated with helical tomotherapy did develop a grade 2 skin reaction. After analyzing his plan, all subsequent plans imposed constraints to keep the skin dose below 35 Gy. No subsequent grade 2 or higher skin reactions were seen. All patients’ symptoms, which were mild, had resolved completely by one month of follow-up.
Table III. Acute toxicities.
Discussion
This study examined the dosimetric and clinical feasibility of IMRT-based dose escalation to the pelvic lymph nodes with concurrent prostate hypofractionation. An increased total dose was delivered to the pelvic lymph nodes (56 Gy at 2 Gy/fraction) while the prostate received hypofractionated “short course” radiation to 70 Gy at 2.5 Gy/fraction Citation[20] in 28 fractions.
In this current study, we have used conformal avoidance target delineation. Highly conformal radiation techniques such as IMRT carry the inherent risk of omitting clinically important regions from radiation therapy. The practice of placing margins around major vessels (common, internal, and external iliacs) may omit less distinct at-risk nodal regions including the peri-prostatic, obturator, and pre-sacral nodes. Conformal avoidance allows the treatment of all regions that would be conventionally treated with a standard 4-field pelvic field, but with the exclusion of dose-limiting normal tissues, thus potentially providing a more comprehensive coverage of nodal targets. For this reason, conformal avoidance has been proposed for head and neck IMRT as well Citation[21].
We explored dose escalation to the pelvic nodal regions in high risk patients both because of emerging evidence regarding the potential importance of treatment of occult lymph node metastases and because of concern that conventional doses may be suboptimal. Tables Citation[4] and formulas Citation[5] employing clinical parameters have been commonly used to estimate the risk of lymph node disease, butemerging data based upon more comprehensive lymph node dissections suggest that these calculated risks of lymph node metastases may be underestimated Citation[22], Citation[23]. These data suggest that a greater than previously appreciated proportion of PSA failures in post-prostatectomy or “prostate-only” RT patients (external beam or brachytherapy) may be due to unaddressed disease within the pelvic lymph nodes.
Studies examining the use of whole pelvis radiotherapy in high risk patients have shown conflicting results, however, with some studies supporting its use Citation[24–30] and others not Citation[31–35]. The lack of consistent success in trials of whole pelvis radiotherapy may reflect an inability to deliver nodal sterilizing doses or an inescapable association between nodal metastases and subsequent distant failure. However, the presence of nodal metastasis, particularly micrometastases, does not invariably predict for incurable disease. Patients with nodal metastases with head and neck, breast, colon, cervical, and endometrial cancer are curable and, in prostate, a study by Bader and colleagues, for example, found the risk of PSA relapse to correlated significantly with number of positive nodes Citation[36],suggesting that some patients with micrometastases may be potentially curable. However, even for tumors in the head and neck with a more radiosensitive squamous cell histology, doses of approximately 60 Gy are required to eradicate involved 1 cm nodes Citation[3]. Given less radiosensitive adenocarcinoma histology and barring a major synergy between androgen deprivation and radiotherapy, 45 Gy may well be insufficient to sterilize microscopic nodal prostate cancer.
A significant concern with increasing dose to the nodal basin of the whole pelvis is that toxicities, particularly GI, GU, and hematologic, will become excessive. While bowel displacement techniques Citation[9], Citation[37] have assisted in limiting side effects, it is unclear if they are sufficient to allow a meaningful increase in elective nodal dose without increasing GI toxicity. Furthermore, conventional 4-field pelvic radiotherapy does not allow for significant sparing of rectum and bladder. Hence, the ability to accomplish nodal dose escalation with conventional pelvic field techniques may be limited. However, several studies in gynecologic malignancies have clearly demonstrated the potential of IMRT to improve pelvic dosimetry and reduce GI toxicities through a reduction in small bowel dose Citation[32], Citation[47]. Roeske et al. examined outcomes in 50 patients treated with WP-IMRT Citation[11] and their NTCP analysis demonstrated that acute GI toxicity increased from 9.9 to 48.2% when volume of small bowel receiving 45 Gy or higher increased from 200 to 400 ml. In our experience reported here, small bowel dose-volume parameters were well below these limits even with high dose nodal irradiation, and correspondingly low rates of acute toxicity were seen.
Other institutions have explored use of pelvic IMRT specifically in the management of prostate cancer, either in studies involving actual patient treatment Citation[38], Citation[39] or in planning-only analyses Citation[40], Citation[41]. These other studies, however, generally employed lower dose nodal irradiation and did not use conformal avoidance strategies.
The hypofractionated schedule delivered in our study is particularly attractive given the increasing evidence for a low α/β ratio for prostate cancer, implying that hypofractionation should increase the therapeutic ratio. Cleveland Clinic investigators, in a study limited, however, to prostate only irradiation, have employed this same hypofractionation schedule of 28 fractions of 2.5 Gy to 70 Gy and have demonstrated PSA recurrence-free survival rates at least equivalent to that seen with standard 2 Gy fractionation to 76 Gy Citation[42]. compares the effective dose (NTD) of this hypofractionated schedule with the control, sequential pelvis-prostate schedule as well as with other commonly employed prostate only fractionation schedules [48–50]. The effective dose to the pelvic nodes is also displayed and is simply the total dose, since nodal treatment is in 2 Gy fractions. While the strongest evidence appears to suggest an α/β ratio of about 1.5 Citation[16], Citation[43], Citation[44], even α/β ratios of 3 Citation[17] and 10 will result in adequately high predicted prostate NTDs
Table IV. Comparison of fractionation schedules. (a) Prostate
Prostate hypofractionation with concurrent 2 Gy fractions to the nodes significantly shortens treatment duration requiring only 5½ weeks to deliver a comprehensive prostate plus nodal irradiation compared with the typical 8–9 weeks required with a sequential pelvis then prostate regimen.
Our dosimetric and clinical results suggest that dose escalation to 56 Gy in 2 Gy/fraction to pelvic lymph nodes can be delivered in a well tolerated fashion. While much of the organ-sparing advantage may derive from IMRT, simple bowel displacement techniques with prone positioning as used here can also contribute significantly to bowel sparing. Daily image guidance using transabdominal ultrasound or megavoltage CT scanning, can also reduce risk by decreasing required set-up margins around the prostate Citation[47], while Tomotherapy MVCT or LINAC on-board CT imaging specifically permits such imaging while patients are optimally positioned prone in a bowel displacement device.
Conclusions
Conformal avoidance based target delineation with hypofractionation of the prostate (H-CAD) represents an integration of prostate dose escalation using hypofractionation and conformal avoidance to significantly escalate the dose to the pelvic lymph nodes. The conformal avoidance-based approach minimizes uncertainties inherent in attempting to directly identify all nodal areas at risk, while the use of simultaneous integrated prostate hypofractionation produces an intense, time and resource efficient treatment. Currently, a phase II study in high-risk patients using this technique is actively accruing at our center.
References
- Jemal A, Tiwari RC, Murray T, Ghafoor A, Samuels A, Ward E, et al. Cancer statistics, 2004. CA Cancer J Clin 2004; 54: 8–29
- Kupelian PA, Elshaikh M, Reddy CA, Zippe C, Klein EA. Comparison of the efficacy of local therapies for localized prostate cancer in the prostate-specific antigen era: A large single-institution experience with radical prostatectomy and external-beam radiotherapy.[see comment]. J Clin Oncol 2002; 20: 3376–85
- Mendenhall WM, Million RR, Bova FJ. Analysis of time-dose factors in clinically positive neck nodes treated with irradiation alone in squamous cell carcinoma of the head and neck. Int J Radiat Oncol, Biol, Phys 1984; 10: 639–43
- Partin AW, Kattan MW, Subong EN, Walsh PC, Wojno KJ, Oesterling JE, et al. Combination of prostate-specific antigen, clinical stage, and Gleason score to predict pathological stage of localized prostate cancer. A multi-institutional update. [see comment] [erratum appears in JAMA 1997 Jul 9;278:118]. JAMA 1997; 277: 1445–51
- Roach M 3rd, Marquez C, Yuo HS, Narayan P, Coleman L, Nseyo UO, et al. Predicting the risk of lymph node involvement using the pre-treatment prostate specific antigen and Gleason score in men with clinically localized prostate cancer. [see comment]. Int J Radiat Oncol, Biol, Phys 1994; 28(1)33–7
- Chinnaiyan P, Tomee W, Patel R, Chappell R, Ritter M. 3D-ultrasound guided radiation therapy in the post-prostatectomy setting. Tech Cancer Res Treat 2003; 2: 455–8
- Tome W, Orton N. On the use of optically guided 3D-ultrasound for daily in room target localization. Med Phys 2005; 32: 2066–7
- Patel RR, Orton N, Tome WA, Chappell R, Ritter MA. Rectal dose sparing with a balloon catheter and ultrasound localization in conformal radiation therapy for prostate cancer. Radiother Oncol 2003; 67: 285–94
- Shanahan TG, Mehta MP, Bertelrud KL, Buchler DA, Frank LE, Gehring MA, et al. Minimization of small bowel volume within treatment fields utilizing customized “belly boards”. Int J Radiat Oncol, Biol, Phys 1990; 19: 469–76
- Langen KM, Zhang Y, Andrews RD, Hurley ME, Meeks SL, Poole DO, et al. Initial experience with megavoltage (MV) CT guidance for daily prostate alignments. Int J Radiat Oncol, Biol, Phys 2005; 62: 1517–24
- Roeske JC, Bonta D, Mell LK, Lujan AE, Mundt AJ. A dosimetric analysis of acute gastrointestinal toxicity in women receiving intensity-modulated whole-pelvic radiation therapy. Radiother Oncol 2003; 69: 201–7
- Löf J. Development of a general framework for optimization of radiotherapy. PhD thesis. Stockholm: Department of Medical Radiation Physics, Stockholm University; 2000.
- Löf J, Lind B, Brahme A. Optimal radiation beam profiles considering the stochastic process of patient positioning in fractionated radiation therapy. Inverse Problems 1995; 11: 1189–209
- Orton NP, Tome WA. The impact of daily shifts on prostate IMRT dose distributions. Med Phys 2004; 31: 2845–48
- Fowler JF. Brief summary of radiological principles in fractionated radiation therapy. Semin Radiat Oncol 1992; 2: 16–21
- Fowler J, Chappell R, Ritter M. Is alpha/beta for prostate tumors really low?. Int J Radiat Oncol, Biol, Phys 2001; 50: 1021–31
- Wang JZ, Li XA, Yu CX, DiBiase SJ. The low alpha/beta ratio for prostate cancer: What does the clinical outcome of HDR brachytherapy tell us?. Int J Radiat Oncol, Biol, Phys 2003; 57: 1101–8
- Schultheiss TE, Lee WR, Hunt MA, Hanlon AL, Peter RS, Hanks GE. Late GI and GU complications in the treatment of prostate cancer. Int J Radiat Oncol, Biol, Phys 1997; 37: 3–11
- Tome WA, Fowler JF. On cold spots in tumor subvolumes. Med Phys 2002; 29: 1590–8
- Kupelian PA, Reddy CA, Klein EA, Willoughby TR. Short-course intensity-modulated radiotherapy (70 GY at 2.5 GY per fraction) for localized prostate cancer: Preliminary results on late toxicity and quality of life. [see comment]. Int J Radiat Oncol, Biol, Phys 2001; 51: 988–93
- Song S, Tome WA, Mehta MP, Harari PM. Emphasizing conformal avoidance versus target definition for IMRT planning in H&N cancer. Int J Radiat Oncol, Biol, Phys 2003; 57S: s299–2300
- Heidenreich A, Varga Z, Von Knobloch R. Extended pelvic lymphadenectomy in patients undergoing radical prostatectomy: high incidence of lymph node metastasis. J Urol 2002; 167: 1681–6
- Heidenreich A, Von Knobloch R, Varga Z, Hofmann R, Engelmann V. Extended pelvic lymphadenectomy in men undergoing radical prostatectomy – unupdate of >300 cases. J Clin Oncol 2004;22:4095 (abst 4612).
- Seaward SA, Weinberg V, Lewis P, Leigh B, Phillips TL, Roach M 3rd. Identification of a high-risk clinically localized prostate cancer subgroup receiving maximum benefit from whole-pelvic irradiation. Cancer J Sci Am 1998; 4: 370–7
- Bagshaw MA, Cox RS, Ray GR. Status of radiation treatment of prostate cancer at Stanford University. NCI Monographs 1988; 7: 47–60
- McGowan DG. The value of extended field radiation therapy in carcinoma of the prostate. Int J Radiat Oncol, Biol, Phys 1981; 7: 1333–9
- Perez CA, Michalski J, Brown KC, Lockett MA. Nonrandomized evaluation of pelvic lymph node irradiation in localized carcinoma of the prostate. IntJournal of Radiat Oncol, Biol, Phys 1996; 36: 573–84
- Ploysongsang S, Aron BS, Shehata WM, Jazy FK, Scott RM, Ho PY, et al. Comparison of whole pelvis versus small-field radiation therapy for carcinoma of prostate. Urology 1986; 27: 10–6
- Rangala N, Cox JD, Byhardt RW, Wilson JF, Greenberg M, Lopes da Conceicao A. Local control and survival after external irradiation for adenocarcinoma of the prostate. Int J Radiat Oncol, Biol, Phys 1982; 8: 1909–14
- Roach M 3rd, DeSilvio M, Lawton C, Uhl V, Machtay M, Seider MJ, et al. Phase III trial comparing whole-pelvic versus prostate-only radiotherapy and neoadjuvant versus adjuvant combined androgen suppression: Radiation Therapy Oncology Group 9413. [see comment]. J Clin Oncol 2003; 21: 1904–11
- Asbell SO, Krall JM, Pilepich MV, Baerwald H, Sause WT, Hanks GE, et al. Elective pelvic irradiation in stage A2, B carcinoma of the prostate: Analysis of RTOG 77–06. Int J Radiat Oncol, Biol, Phys 1988; 15: 1307–16
- Aristizabal SA, Steinbronn D, Heusinkveld RS. External beam radiotherapy in cancer of the prostate. The University of Arizona experience. Radiother Oncol 1984; 1: 309–15
- Perez AA, Pilepich MV, Zivnuska F. Tumor control in definitive irradiation of localized carcinoma of the prostate. Int J Radiat Oncol, Biol, Phys 1986; 12: 523–31
- Rosen E, Cassady JR, Connolly J, Chaffey JT. Radiotherapy for prostate carcinoma: The JCRT experience (1968–1978). II. Factors related to tumor control and complications. Int J Radiat Oncol, Biol, Phys 1985; 11: 723–30
- Zagars GK, von Eschenbach AC, Johnson DE, Oswald MJ. The role of radiation therapy in stages A2 and B adenocarcinoma of the prostate. Int J Radiat Oncol, Biol, Phys 1988; 14: 701–9
- Bader P, Burkhard FC, Markwalder R, Studer UE. Disease progression and survival of patients with positive lymph nodes after radical prostatectomy. Is there a chance of cure? [see comment]. J Urol 2003; 169: 849–54
- Das IJ, Lanciano RM, Movsas B, Kagawa K, Barnes SJ. Efficacy of a belly board device with CT-simulation in reducing small bowel volume within pelvic irradiation fields. Int J Radiat Oncol, Biol, Phys 1997; 39: 67–76
- Nutting CM, Convery DJ, Cosgrove VP, Rowbottom C, Padhani AR, Webb S, et al. Reduction of small and large bowel irradiation using an optimized intensity-modulated pelvic radiotherapy technique in patients with prostate cancer. Int J Radiat Oncol, Biol, Phys 2000; 48: 649–56
- Sanguineti G, John B, Franzone P, Culp L, Sosa M, Cavey M, et al. IMRT to treat the pelvic nodes while escalating the dose to the prostate gland: Acute toxicity data in 45 consecutive patients. Int J Radiat Oncol, Biol, Phys 2005; 60(1S)s446(abstract 2217)
- Li XA, Wang JZ, Jursinic PA, Lawton CA, Wang D. Dosimetric advantages of IMRT sumultanueous integrated boost for high-risk prostate cancer. Int J Radiat Oncol, Biol, Phys 2005;61(4).
- Price RA, Hannoun-Levi J, Ma CC, Pollack A. Pelvic lymphatic coverage in the treatment of prostate cancer with intensity modulated radiation therapy: A feasibility study. Int J Radiat Oncol, Biol, Phys 2004; 60(S1)s635(abstract 2491)
- Kupelian PA, Reddy CA, Carlson TP, Altsman KA, Willoughby TR. Preliminary observations on biochemical relapse-free survival rates after short-course intensity-modulated radiotherapy (70 Gy at 2.5 Gy/fraction) for localized prostate cancer. Int J Radiat Oncol, Biol, Phys 2002; 53: 904–12
- Fowler JF, Ritter MA, Chappell RJ, Brenner DJ. What hypofractionated protocols should be tested for prostate cancer?. Int J Radiat Oncol, Biol, Phys 2003; 56: 1093–104
- Bentzen SM, Ritter MA. The alpha/beta ratio for prostate cancer: What is it, really? [comment]. Radiother Oncol 2005; 76: 1–3
- Pollack A, Zagars GK, Starkschall G, Antolak JA, Lee JJ, Huang E, et al. Prostate cancer radiation dose response: Results of the M. D. Anderson phase III randomized trial.[see comment]. Int J Radiat Oncol, Biol, Phys 2002; 53: 1097–105
- Zelefsky MJ, Fuks Z, Hunt M, Yamada Y, Marion C, Ling CC, et al. High-dose intensity modulated radiation therapy for prostate cancer: Early toxicity and biochemical outcome in 772 patients. Int J Radiat Oncol, Biol, Phys 2002; 53: 1111–6
- Keller H, Tome W, Ritter MA, Mackie TR. Design of adaptive treatment margins for non-negligible measurement uncertainty: Application to ultrasound-guided prostate radiation therapy. Phys Med Biol 2004; 49: 69–86