Abstract
Presentation and comparison of tomotherapeutic intensity-modulated techniques for planning and delivery of stereotactic body radiation therapy. Serial tomotherapeutic SBRT has been planned and delivered at our institution since 8/2001. Since 12/2005, 12 patients have been treated using a helical tomotherapy unit. For these 12 patients both helical and serial tomotherapy plans were computed and clinically approved. Techniques and considerations of tomotherapy SBRT planning, associated image-guidance, and delivery are presented. The respective treatment plans were compared based on dosimetric parameters as well as time to develop a treatment plan and delivery times. Also the associated quality of megavoltage CT (MVCT) image-guidance inherent to the helical tomotherapy unit was assessed. Tumor volumes averaged 9.3, 9.8, and 58.7 cm3 for liver, lung, and spinal targets. Helical and serial tomotherapy plans showed comparable plan quality with respect to maximum and average doses to the gross tumor and planning target volumes. Time to develop helical tomotherapy plans averaged 3.5 h while serial tomotherapy planning consistently required less than one hour. Treatment delivery was also slower using helical tomotherapy, with differences of less than 10 min between modalities. MVCT image-guidance proved satisfactory for bony and lung targets, but failed to depict liver lesions, owing to poor soft-tissue contrast. SBRT planning and delivery is clinically feasible using either tomotherapeutic modality. While treatment planning time was consistently shorter and more readily accomplished in a standardized approach using the serial tomotherapy modality, actual plan quality and treatment delivery times are grossly comparable between the modalities. MVCT volumetric image-guidance, was observed to be valuable for thoracic and spinal target volumes, whereas it proved challenging for liver targets.
Two commercial modalities for planning and delivery of tomotherapeutic intensity-modulated stereotactic body radiation therapy (SBRT) are clinically available today, serial and helical tomotherapy.
At the University of Texas Health Science Center at San Antonio and the Cancer Therapy & Research Center, San Antonio, Texas, USA, serial tomotherapy provided via the Nomos Peacock system (Nomos Corp, Cranberry Township, PA, USA) has been utilized to plan and deliver SBRT to over 180 patients since August 2001. The key feature of this tomotherapy system is a rotational slice-by-slice delivery of radiation. The associated beam modulator or multi-leaf collimator is an add-on pneumatically driven binary slit collimator (MIMiC, Nomos Corp, Cranberry Township, PA, USA) mounted onto a linear accelerator. Two rows of tungsten vanes enable simultaneous treatment of two independent “slices” of 4, 8.5 or 17 mm length each. Precise table indexing from delivery slice to delivery slice is enabled by a couch mounted indexing device (Crane/AutoCrane, Nomos Corp, Cranberry Township, PA, USA) Citation[1].
Since December 2005, SBRT has also been delivered using a helical tomotherapy unit (HiArt, TomoTherapy Inc., Madison, WI, USA). While helical tomotherapy shares the basic concept of rotational beam delivery via a binary pneumatically driven beam modulator with serial tomotherapy, significant differences between the modalities exist. The HiArt system is a slip-ring based dedicated tomotherapy unit, resembling the doughnut style of a computed tomography scanner. Radiation is delivered in a continuous rotational fashion with dynamic table movement enabling a helical mode of radiation administration. Similar to diagnostic CT image acquisition, the pitch can be selected to more finely assume the shape of a targets outline via fixed pencil beam lengths of 11, 25 or 50 mm. Built into this delivery system is a megavoltage CT detector array allowing the acquisition of megavoltage CT images (MVCT) for image-guidance Citation[2], Citation[3].
Based on 12 patients treated by SBRT using the helical tomotherapy unit, we aim to present the specific treatment planning and delivery processes for serial and helical tomotherapy and to compare resulting SBRT treatment plans with regard to dosimetric parameters, and time to compute and deliver the treatment plans. We also attempt to assess the quality and feasibility of MVCT image guidance compared with the clinically established process of diagnostic CT based volumetric image-guidance.
Materials
Patient and tumor characteristics
Patients treated by SBRT were diagnosed with inoperable stage 1 non small-cell lung cancer (n = 5), lung metastases (n = 1), liver metastases (n = 3), and vertebral body/paraspinal metastases (n = 3).
SBRT: Immobilization, simulation, and treatment concept
All patients were immobilized using the BodyFix whole body double-vacuum immobilization system (Medical Intelligence, Schwabmuenchen, Germany) according to published experience Citation[4], Citation[5].
Simulation imaging was acquired on a GE Lightspeed RT big-bore 4-slice CT simulator (General Electric Healthcare, Waukesha, WI, USA). All patients underwent one free breathing CT scan and additional four-dimensional CT imaging (4DCT). Image data were reconstructed in 2.5 mm slice thickness. The 4DCT datasets were processed on a GE Advantage Workstation and reconstructed in maximum intensity projection (MIP). The free-breathing scan as well as the MIP reconstructed image data were exported to the Corvus 5.0/6.0 treatment planning station (Nomos Corp, Cranberry Township, PA, USA) for data co-registration and target and organ-at-risk segmentation. The target, defined as a gross tumor volume (GTV), was based on the 4D MIP image data and compared with target rendering in the free-breathing scan to ensure full target coverage. The 4D-GTV was expanded into a PTV by addition of 5 mm safety margins. Tomotherapy treatment planning for both serial and helical tomotherapy was planned on these identical datasets, with the exception of added tuning structures for helical tomotherapy planning as explained separately.
Dose prescription was 3×12 Gy (total dose 36 Gy) as the minimum dose to 95% of the PTV for liver metastases and the lung metastasis. Dose prescription for inoperable stage 1 NSCLC was 3×20 Gy and for spinal metastases 5×5 Gy, respectively. Both serial and helical tomotherapy plans had to satisfy these constraints for clinical approval. Following typical dose prescription for radiosurgery planning with an emphasis on dose conformality and steep dose gradients, we aimed for an inhomogeneous dose distribution with maximum doses approaching or exceeding 150% Citation[6].
From a clinical standpoint, the helical tomotherapy plan was considered the primary treatment plan and every attempt was made to actually deliver the entire treatment course on the helical tomotherapy unit. The respective serial tomotherapy plan was considered a backup plan to assure that all scheduled fractions would be delivered timely in case of unforeseen difficulties treating on the helical tomotherapy unit or technical unavailability of the unit. Also, only the generation of a serial tomotherapy plan did assure that the established process of volumetric image-guidance using the simulation CT scanner as previously reported Citation[4], could be maintained.
Serial tomotherapy SBRT planning
Inverse SBRT for serial tomotherapy requires the numeric entry of parameters of the desired resulting treatment plan into a template. The prescribed dose (PD), a percentage of the target volume allowed to receive a lesser dose (3%), the minimum dose (95% of PD), and the maximum allowed dose to the target (200% of PD) were defined. Similarly, dose constraints to normal tissues and organs at risk are entered. In order to emphasize dose conformality and steepness of dose gradient over in-target dose homogeneity, software inherent intensity-modulated radiosurgery (IMRS) target and tissue types were selected. In clinical routine, these parameters are scripted and can be recalled for standardized treatment planning of similar cases once defined. In addition, the dimensions of the pencil beams, here always 8.5×10 mm, and the range of rotation about the patient (here always 340 arcing degrees) was defined. Treatment plans were optimized using simulated annealing optimization. All plans were normalized so that 95% of the PTV was exposed to the PD. Typical resulting dose distributions are depicted in .
Figure 1. Display of serial (top figures) and helical tomotherapy planned dose distributions for a liver metastasis, primary lung tumor, and spinal lesion. Doses are displayed as 100% of prescribed dose in blue, 95% in red, 70% in yellow, and 50% in green. Note the planning tuning structures in helical tomotherapy plans; yellow hull structure for both lung and liver lesion, and purple wedge for spinal lesion.
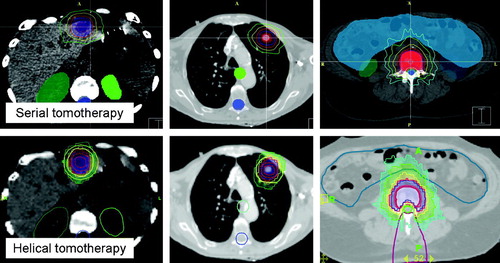
Helical tomotherapy SBRT planning
CT image data and associated structure sets were exported from Corvus to the helical tomotherapy planning station by use of RTOG DICOM RT export. Prior to this structure export, the simulator table was delineated and added to the structure set. Upon import of the CT and structure set data, this simulator table is virtually replaced by the TomoTherapy HiART specific table for treatment planning to account for changes in attenuation. Following a ranking of target structures and organs at risk which defines prioritization for voxels with potentially shared denomination, the inverse planning process requires numeric population of a prescription template. The GTV was prioritized over the PTV so that a differential dose prescription to the target structures was enabled. The system requires an importance setting for targets which is initially set to 50, admittedly an arbitrary number. For the GTV we stipulated 100% coverage by the PD, whereas the PD for the PTV was prescribed to 95% of the volume. Also, the minimum dose (95% of PD), and the maximum allowed dose to the target (200% of PD for the GTV and 150% of PD for the PTV) were defined. The system allows to enter a penalty for exceeding the maximum and minimum doses to the defined volumes which was set to 1, the lowest setting for the maximum dose and to 100 for the minimum dose. Thus, the maximum dose constraint was set very loosely, whereas the requirement to expose 100 and 95% of GTV and PTV, respectively, was constrained tightly. Similarly, organs-at-risk were ranked, and dose constraints were entered.
Utilizing these parameters, initial treatment plans showed maximum doses not exceeding 105%, and were, thus, inconsistent with our previous practice and experience in planning and delivering tomotherapeutic IMRT. In order to achieve dose distributions similar to the ones delivered for more than four years of SBRT practice, additional “tuning structures” were developed. For lung and liver targets, we use a “hull” structure around the PTV with a distance of approximately 10 mm from the surface of the PTV (see ). This hull structure is delineated in addition to existing target and organ-at-risk structures and exported to the helical treatment planning station. For SBRT planning, this hull structure is assigned a higher importance than the target volume (twice the targets importance). Dose limitations for this hull structure are set at maximum doses of no more than 25% of PD, with maximum dose penalties of 1 000 times the maximum dose penalty for the target. Also, only 20% of this structure is allowed to receive more than 15% of the PD. This strategy of asking the inverse planning system for a solution that can realistically not be satisfied, results in an inhomogeneous dose within the target volume with maximum dose consistently approaching or exceeding 150% of the PD. For spinal targets, a tuning structure that creates a wedge of tissue extending from the posterior surface of the spinal cord to the posterior surface of the patient is created. This structure also caps the target volume with a distance of two slices of tissue between tuning structure and cranial/caudal aspect of the target volume. The intent here is avoidance of islands of high dose behind the spinal cord or a circumferential high dose region around the cord and high dose conformality on the cranial and caudal aspects of the target, by limiting the maximum dose to this tuning structure to 80% of the PD. In addition to dose prescription parameters, the physical pencil beam size and pitch are selected. The dose modulation factor, which defaults to a value of 2.0, is typically raised to 2.5.
The actual treatment planning process for helical tomotherapy is split into two phases: phase 1 during which the plan is submitted and the beamlets, these are all pencil beams that expose the target and organs at risk, are identified and their individual dose contribution is calculated, and phase 2 during which the user or planner interacts with the inverse treatment planning system based on a preliminary DVH and dose distributions displayed.
Treatment delivery and image-guidance
All patients were immobilized in the BodyFix system for SBRT delivery on the simulator CT treatment table and underwent control CT imaging as reported Citation[4], Citation[6–8]. The acquired CT data were transferred to the Corvus treatment planning system for co-registration with the original planning CT study and assessment of patient and target setup Citation[4]. When a target setup to within 5 mm of planned position was assured, the patients were transferred in the BodyFix system onto the helical tomotherapy unit treatment table. Following alignment to room lasers, a MVCT study was acquired and a console inherent registration module was used to assess patient and target setup Citation[3], Citation[9], Citation[10].
Analysis
Data collected for plan comparison include volumes of GTVs and PTVs, the maximum and average plan doses to GTV and PTV, ratios between maximum and average plan doses and prescribed dose, the actual pencil beam dimensions used, pitch for helical tomotherapy plans, and total monitor units (MU). Times to calculate treatment plans are not closely tracked by either modality; thus, a close clinical estimate was attempted. Time to deliver the helical tomotherapy treatment plans was derived from the planning system output plus a fixed component of 5 min setup time. In those cases in which a complete SBRT fraction had to be delivered in two or three repeats due to exceeding maximum delivery dose limits inherent to the unit, additional cool down and setup check times of 5 min for each repeat were added. Serial tomotherapy treatment times were calculated as total MU/600 times number of repeats or table indices times 0.5 min plus 5 min fixed setup time (the delivery unit was a 600 MU/minute Varian G600 linear accelerator, Varian, Palo Alto, CA, USA; repeat arc delivery and/or table indexing between treatment slices requires typically no more than 20 s in clinical routine). Delivery times derived disregard the time component associated with image-guidance, as all patients underwent volumetric image-guidance on the diagnostic CT unit and setup assessments, but only helical tomotherapy deliveries would be subjected to additional MVCT image guidance accounting for an additional 7 to 12 min, and includes MVCT acquisition, MVCT/KVCT co-registration, assessment and positional corrections.
Analysis of MVCT image quality was based on the value of MVCT imaging for actual image-guidance, and a side by side comparison with original planning CT study and control CTs. Scored was image quality as acceptable/unacceptable to identify the target volume and discriminate from organ background for MVCT and diagnostic CT, and suitability/non-suitability for image guidance.
Results
Volumes of targets
Average GTV volume of liver lesions, primary lung tumors and spinal targets was 9.3 (range 3.8 to 15.8 cm3), 9.8 cm3 (range 3.6 to 20.2 cm3), and 58.7 cm3 (range 49.7 to 74.6 cm3), respectively. The sole lung metastasis had a GTV and PTV volume of 3.8, and 20.7 cm3, respectively. The respective average PTVs (GTV plus margins) for liver, primary lung and spinal targets were 27.8 (range 26.9 to 41.7 cm3), 29.8 cm3 (range 14.8 to 51.9 cm3), and 82.7 cm3 (range 68.6 to 105.8 cm3).
Plan parameters
Pencil beam sizes utilized were the 11 mm pencil beam used in all but three primary NSCLC cases for helical tomotherapy planning for which the larger 25 mm pencil beams were used. Pitch ranged between 0.108 (equivalent to 9.26 rotations for a full pencil beam length table increment) and 0.3 (3.33 rotations for pencil beam length increment), with 0.287 being the most commonly used pitch (7/12 cases). Pitch smaller than 0.287 was used when larger pencil beams (25 mm) were used and on a case by case basis when tighter pitch provided for tangible improvements in treatment plan quality.
All serial tomotherapy plans were calculated using the 8.5 mm pencil beam. Pitch cannot be selected but is by default always 1, thus, the table is incremented by the pencil beam length as projected at machine isocenter. The number of table indices is defined by target length in cranio-caudal direction and ranged from 2 to 3.
Average maximum plan doses as well as mean GTV and PTV doses for the respective target sites are summarized in .
Table I. Dose parameters for serial and helical tomotherapy plans
Total MU per serial tomotherapy plan averaged 8 848 MU for 25 Gy plans (spine), 10 108 MU for 36 Gy plans (lung and liver metastases), and 18 717 MU for primary lung tumor plans (PD 60 Gy). The respective helical tomotherapy plans averaged 17 858 MU, 17 170 MU, and 33 819 MU.
Treatment planning and plan delivery times
Times required for treatment planning are reported in excluding the time required to import the CT image data, image data co-registration, and target delineation as these steps are common to either tomotherapy modality, owing to the fact that structure delineation capabilities are not available in the helical tomotherapy planning system.
Table II. Summary of times required to plan and deliver serial and helical tomotherapeutic SBRT
Typical SBRT treatment planning using the serial tomotherapy unit requires filling in templates (dose prescription, optimizer settings, PTV margins, and machine delivery parameters), most of the entries are typically scripted. Owing to our extensive experience in serial tomotherapeutic SBRT planning, a second or third iteration to further improve a treatment plan is rarely needed, limiting total treatment planning time.
Helical tomotherapy treatment planning requires initial dataset preparation as outlined earlier, and selecting treatment parameters including dose requirements and constraints. The key parameters of SBRT planning are again scripted. Remote beamlet calculation time depends on pencil beam size selected and pitch. Smaller pencil beams, tighter pitch, and longer target volumes in cranio-caudal direction force longer beamlet calculation times. The third step of helical tomotherapy inverse planning is interactive; thus, the planner(s) (physician and dosimetrists) ideally reside at the planning console. Interactions with the planning system are based on the developing DVH and preliminary dose distributions displayed. While target volume dose requirements are often fulfilled in as little as 25 optimization iterations of 30 s or less, normal tissue or organ-at-risk constraints may require staged interactions and manual changing of parameter settings. Ending this interactive process is a full dose calculation, which takes scatter contributions into consideration. This iterative component of treatment planning consumed up to 2.5 h, with 1.5 h being a realistic average. Total planning times summed up to about 3.5 h, with less than half of this time not requiring direct user interaction.
Treatment delivery times are summarized in .
Image guidance: comparison of image quality
With the image quality or depiction of the patients anatomy rendered by the simulator CT as the gold-standard, MVCT rendered bony anatomy and lesions in the low density environment of the lung sufficiently to rate image quality acceptable and possibly also suitable for simulation imaging (). Soft tissue lesions, here limited to liver lesions, were poorly rendered and could not or only poorly be discriminated from the harboring organ. Of three liver lesions treated, only the largest liver metastasis depicted in was appreciable in MVCT. As a result of this assessment, MVCT image-guidance was considered suitable for lesions embedded into lung parenchyma (no mediastinal lesions were assessed), or lesions in close relationship with the patients bony anatomy. As MVCT may fail to provide suitable image-guidance for liver lesions, corrective shifts needed to be derived from other image-guidance modalities such as the diagnostic grade control CT study as to our established clinical practice.
Figure 2. Volumetric CT based image-guidance for liver, lung and spinal targets. The top row depicts image-guidance provided by the simulation kilovoltage CT scanner (KVCT). The lower figures show the corresponding megavoltage CT (MVCT) images acquired on the helical tomotherapy unit. The arrow indicates the liver metastasis in KVCT imaging. Depicted here are the various strategies to evaluate patient setup for image-guidance. Image-data are co-registered to the original simulation imaging and corrective shifts can be derived by assessing the fit between the imaging studies, and/or superimposing isodose distributions or structure outlines onto the control CT scan.
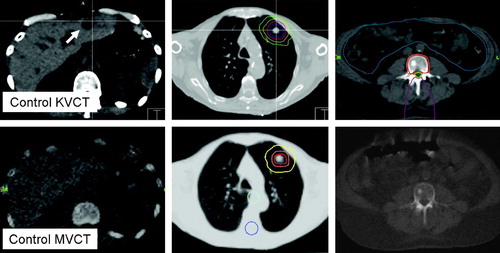
Discussion
This is the first and preliminary report of clinical experience in the use of a helical tomotherapy unit for planning and delivery of SBRT and its comparison with serial tomotherapy. We focused on the workflow and feasibility of planning, delivery and quality of associated image-guidance. The theoretical advantage of an integrated planning, image-guidance, and delivery unit providing volumetric MVCT image data and an inherent image-guidance workflow, was found to provide clinically acceptable SBRT plans that could be delivered in a reasonable time frame. The obvious advantage of helical over serial tomotherapy is the potential to conduct the image-guidance process on the actual treatment table without need to relocate the patient from one imaging modality onto the treatment table. This eliminates the associated risk for positional variability of the patient within the immobilization system between two setups. This advantage might be negated by placing the serial tomotherapy hardware on a linear accelerator fitted with on-board cone-beam CT imaging capabilities.
The helical tomotherapy provided MVCT imaging was found suitable for depiction of bony anatomy and targets embedded into the low-density environment of the lung, consistent with the experience in animals as well as humans in prior reports Citation[9], Citation[10]. Despite the fact that the SBRT targets in the present series were much smaller than targets treated in a more conventional fractionation, both lung metastasis and primary lung tumors could be clearly discriminated from the lung background providing for a high level of confidence in the image-guidance process. However, MVCT image-guidance for liver lesions failed to render the lesions reliably. Owing to a poor soft-tissue contrast, image-guidance for liver lesions will require additional measures such as the possible insertion of radio-opaque fiducial markers. Alternatively, means of ultrasound-based image-guidance need to be implemented Citation[11–14] or the surrogate process of using the simulator CT for volumetric image-guidance needs to be maintained Citation[4], Citation[6–8].
Plan quality was grossly comparable between helical and serial tomotherapy plans, under the provisions of adding tuning structures for helical tomotherapy planning as described. The treatment planning process was much shortened when developing serial tomotherapy plans; however, future hardware and software developments will probably shorten the currently crippling slow helical tomotherapy planning process.
To our surprise, treatment delivery times were actually longer when using the helical tomotherapy unit, whereas on average by less than 10 min. This is without doubt related with the use of the smallest available pencil beam dimension (11 mm) for helical tomotherapy planning and the associated tight pitches. In three cases of SBRT planning for primary NSCLC and thus deliveries of the highest doses in the present series (3×20 Gy), we had to choose the larger 25 mm pencil beam in order to shorten the treatment delivery times into a reasonable time window. While the smaller pencil beams would have provided marginally better treatment plans, the clinically accepted plans fulfilled all stipulated criteria while cutting delivery time almost in half. Since excessively long treatment delivery times might cause patients to start moving due to increasing discomfort and loss of compliance, those rather marginal dosimetric advantages might have been lost in actual delivery. We are currently conducting a comparison of gains and tradeoffs when using different sized pencil beams and pitch settings, data of which is not yet available.
The stipulation of developing both a primary helical tomotherapy plan as well as a backup serial tomotherapy plan may not be continued as all prescribed fractions in this small series could be delivered on scheduled treatment days, whereas partially with time delays or with short, but recoverable interruptions. We had established this policy when initiating clinical operation of the helical tomotherapy unit and to date have pursued the resource intensive process of creating clinical doublets of plans.
Outcome assessments in the present small series of patients are limited to between one and three follow-up visits and associated CT and/or PET imaging. While very preliminary, those early clinical and imaging outcomes match observation made in early follow-up following serial tomotherapy delivery, with a lack of associated underlying organ toxicity and indications of tumor response. Since the overall treatment concept was not changed, similarly favorable outcomes for serial and helical tomotherapy treated patients are expected.
M. Fuss would like to thank Nomos Corp. and TomoTherapy Inc. for funding the research. He is a member of the Clinical Protocol Development committee at TomoTherapy Inc., Madison, WI and a member of the Technical Advisory Committee at Nomos Corp., North American Scientific, Cranberry Township, PA. N. Papanikolaou would also like to thank TomoTherapy Inc. for funding his research
References
- Salter BJ, Hevezi JM, Sadeghi A, Fuss M, Herman TS. An oblique arc capable patient positioning system for sequential tomotherapy. Med Phys 2001; 28: 2475–88
- Mackie TR, Balog J, Ruchala K, Shepard D, Aldridge S, Fitchard E, et al. Tomotherapy. Semin Radiat Oncol 1999; 9: 108–17
- Welsh JS, Patel RR, Ritter MA, Harari PM, Mackie TR, Mehta MP. Helical tomotherapy: An innovative technology and approach to radiation therapy. Technol Cancer Res Treat 2002; 1: 311–6
- Fuss M, Salter BJ, Rassiah P, Cheek D, Cavanaugh SX, Herman TS. Repositioning accuracy of a commercially available double-vacuum whole body immobilization system for stereotactic body radiation therapy. Technol Cancer Res Treat 2004; 3: 59–67
- Nevinny-Stickel M, Sweeney RA, Bale RJ, Posch A, Auberger T, Lukas P. Reproducibility of patient positioning for fractionated extracranial stereotactic radiotherapy using a double-vacuum technique. Strahlenther Onkol 2004; 180: 117–22
- Lax I, Blomgren H, Naslund I, Svanstrom R. Stereotactic radiotherapy of malignancies in the abdomen. Methodological aspects. Acta Oncol 1994; 33: 677–83
- Herfarth KK, Debus J, Lohr F, Bahner ML, Fritz P, Hoss A, et al. Extracranial stereotactic radiation therapy: Set-up accuracy of patients treated for liver metastases. Int J Radiat Oncol Biol Phys 2000; 46: 329–35
- Wulf J, Hadinger U, Oppitz U, Thiele W, Ness-Dourdoumas R, Flentje M. Stereotactic radiotherapy of targets in the lung and liver. Strahlenther Onkol 2001; 177: 645–55
- Welsh JS, Bradley K, Ruchala KJ, Mackie TR, Manon R, Patel R, et al. Megavoltage computed tomography imaging: A potential tool to guide and improve the delivery of thoracic radiation therapy. Clin Lung Cancer 2004; 5: 303–6
- Forrest LJ, Mackie TR, Ruchala K, Turek M, Kapatoes J, Jaradat H, et al. The utility of megavoltage computed tomography images from a helical tomotherapy system for setup verification purposes. Int J Radiat Oncol Biol Phys 2004; 60: 1639–44
- Boda-Heggemann J, Walter C, Mai S, Dobler B, Dinter D, Wenz F, et al. Frameless stereotactic radiosurgery of a solitary liver metastasis using active breathing control and stereotactic ultrasound. Strahlenther Onkol 2006; 182: 216–21
- Fuss M, Salter BJ, Cavanaugh SX, Fuss C, Sadeghi A, Fuller CD, et al. Daily ultrasound-based image-guided targeting for radiotherapy of upper abdominal malignancies. Int J Radiat Oncol Biol Phys 2004; 59: 1245–56
- Fuss M, Thomas CR, Jr. Stereotactic body radiation therapy: An ablative treatment option for primary and secondary liver tumors. Ann Surg Oncol 2004; 11: 130–8
- Fuss M, Salter BJ, Herman TS, Thomas CR, Jr. External beam radiation therapy for hepatocellular carcinoma: Potential of intensity-modulated and image-guided radiation therapy. Gastroenterology 2004; 127: S206–17