Abstract
The curative treatment of oligometastases with radiotherapy remains an area of active investigation. We hypothesise that treating oligometastases with SBRT can prolong life and potentially cure patients, while in patients with multiple lung metastases SBRT can improve quality of life. Fifty patients with lung metastases were treated on this study. Individuals with five or fewer total lesions were treated with curative intent. Individuals with > five metastases were treated palliatively. Most patients (62%) received 5 Gy/fraction for a total of 50 Gy. The number of targets treated per patient ranged from one to five (mean 2.6). Tumor sizes ranged from 0.3–7.7 cm in maximal diameter (median 2.1 cm). Mean follow-up was 18.7 months. Local control of treated lesions was obtained in 42 of 49 evaluable patients (83%). Of the 125 total lesions treated, eight progressed after treatment (94% crude local control). The median overall survival time from time of treatment completion of the curatively treated patients was 23.4 months. The progression-free survival of the same group of patients was 25% and 16% at 12 and 24 months, respectively. Grade 1 toxicity occurred in 35% of all the patients, 6.1% had grade 2 toxicity, and 2% had grade 3 toxicity. Excellent local tumor control rates with low toxicity are seen with SBRT. Median survival time and progression-free survival both appear better than that achieved with standard care alone. Long-term progression-free survival can be seen in a subset of patients when all tumors are targeted
For most cancers, the primary cancer itself can be controlled locally Citation[1]. The usual approach to treatment of the primary tumor features combined modality therapy, emphasizing a local therapy, typically surgery or radiation. Deaths most commonly result from tumor that has spread. Therapy for metastastic tumors, unlike that for primary tumors, typically features only systemic chemotherapy or palliative radiation. The latter is given at “palliative” doses that are not expected to be sterilizing, but which reduce symptoms and delay tumor growth.
Metastatic disease to the lung is one of the most common life threatening complications of cancer. Metastases to the lung are very common and can be seen with most cancer types. The lethal outcome is hypoxia with ultimate asphyxiation. The impact of lung metastases is substantial, with most patients surviving only about a year and rare survivors at three years Citation[2]. Thus treatments that might reduce the severity of, or that delay the onset of, hypoxia without themselves altering pulmonary function are of great interest. Indeed there is evidence of long-term survival for surgical metastectomy for sarcoma and breast cancer, though the approach is not fully utilized Citation[2].
Chemotherapy remains the standard of care and can prolong survival time, but long-term survivors with metastatic disease are extremely rare. Progression-free survivors after chemotherapy are not generally expected; however effective chemotherapy, which produces a complete or near complete response, might successfully down-stage micrometastatic disease, leaving patients oligometastastic. Oligometastasis refers to a situation wherein metastases might not be disseminated, butrather present only in a few sites. In this case, it is possible that chemotherapy down-staging can leave a patient curable by local therapy alone. Surgery might be considered for easily resectable lesions. Other minimally invasive or non-invasive therapies might also be considered for control of macroscopic residual disease.
Stereotactic Body Radiation Therapy (SBRT) is a method for delivering focused radiation fields targeting almost exclusively the tumor nidus while excluding tissues not grossly involved with tumor. We hypothesized that aggressive management of pulmonary metastases, particularly in the presence of effective chemotherapy, would reduce pulmonary complications of cancer and prolong survival.
In this study we investigated the feasibility and potential utility of SBRT (using Novalis® Shaped Beam Surgery™) combined with respiratory gating in patients with lung metastases. High total dose radiation therapy with hypofractionation was used with the aim of achieving permanent local control.
Materials and methods
Patient characteristics
Between February 2001 and December 2005, 50 patients were treated on this phase II study of hypo-frationated SBRT for lung metastases. The study was approved by the University of Rochester Medical Center institutional review board. Patients were stratified into two categories. Those with metastatic disease confined to the thorax and/or with total metastases limited to five total lesions (termed curative), and those with more extensive disease wherein lung metastasis were considered the most life limiting component of their disease (termed palliative). Sequential and concurrent chemotherapy was encouraged. While patients were allowed to enroll with or without a chemotherapy response, most patients who enrolled had failed adjuvant chemotherapy (if applicable) and at least one course of systemic chemotherapy. Concurrent anthrocyclines were excluded. Patients with poor baseline pulmonary function or extensive emphysema were not excluded, but there were more stringent dose volume histography (DVH) constraints imposed.
Patients with local control and minimal side effects after a previous course of SBRT were allowed to undergo repeat cycles of SBRT for new lesions. In this study only the index lesions are reported according to protocol, though treatment of additional lesions may have been needed to obtain disease free status.
Stereotactic Body Radiation Therapy technique
Patient immobilization for initial simulation and treatment was accomplished using the ExacTrac® patient positing platform (BrainLAB® Inc.). This platform consists of a vacuum cushion for initial positioning, and infrared reflecting body fiducial markers monitored by two ceiling mounted infrared cameras. Respiratory gating was accomplished using relaxed expiratory breath hold techniques. The therapist monitors, real time, the infrared system and turns off the beam if any axial dimension exceeds 1 mm. The beam is not turned on unless all axial dimensions are under 0.5 mm. Using this technique we have shown the standard deviation of motion between treatment is under 1.8 mm anterior-posterior and lateral, and under 2.3 mm superior-inferior Citation[3]. Quality assurance is further confirmed by a minimum of four computerized tomographic (CT) scans per patient.
Treatment planning was performed using a 3D treatment planning system. CT supplemented by magnetic resonance imaging (MRI) and positron emission tomography (PET) data was used to ensure the accurate definition of gross tumor volume (GTV). The use of arcs and non-coplanar beams was encouraged. A DVH was calculated of any irradiated vital organs including the lung, liver, spinal cord, stomach, cardiac ventricles, esophagus, and kidneys. The allowed maximum DVH for the liver, lung, and spinal cord was specified. There was no specific size limit placed on tumor diameter. Instead, the DVH was used to control the size and number of tumors that could be safely treated. The combined left and right lung DVH was used to calculate the percentage of total lung volume (excluding the GTV) receiving ≥ 10 Gy (V10) and ≥ 20 Gy (V20).
The target volume was the GTV with no expansion for the clinical target volume (CTV). The GTV was defined by CT, MRI, or image fusion of both. The target volume was determined by a radiation oncologist certified for submission of patients onto the protocol. The volume includes imaging abnormalities believed to encompass the metastasis(es) but not microscopic extension. While it is known that tumors can infiltrate about the imaged abnormality, infiltration was not included in the target volume. The 80% isodose volume (defined as the planning target volume (PTV)) was designed to include the GTV with 7 mm of lateral and anterior-posterior margin, and 10 mm of superior inferior margin. There was no effort made to assure homogeneous dose to the isocenter or throughout the CTV, and the prescription dose was the isocenter dose (100% isodose point).
The target dose acceptability was determined based on the DVH of normal (uninvolved) lung and surrounding organs. Normal lung volume was defined as the portion of the lungs not radiographically involved by the GTV. Briefly, patients were required to have 1000 ml of tumor-free lung. For patients with chronic obstructive pulmonary disease or existing chronic lung disease, 70% of the lung or 800 ml (whichever was larger), was kept under 1.7 Gy per fraction for a total dose of less than 17 Gy. For patients with otherwise healthy lungs, 60% of the lung was kept under 2.0 Gy per fraction for a total dose of less than 20 Gy.
Follow-up
Patients were evaluated by the treating physician weekly during treatment including physical exam and appropriate blood work. Additional follow-up visits were planned at one month following completion of treatment and every three months after that for 24 months. Imaging at each visit included at minimum 3D evaluation of the chest (such as CT±PET) and other studies as clinically indicated (such as bone scan). Most surviving patients maintained a rigorous three-month visit schedule after official protocol completion, and all patients have continued close follow-up schedules.
Evaluation of response
The primary objective was to determine if radiotherapy for lung metastases combined with standard therapy is feasible and shows promise in a phase II study. The primary endpoint was local control. Secondary endpoints included regional control, and distant metastasis frequency. Response Evaluation Criteria in Solid Tumors (RECIST) was also evaluated Citation[4]. Local control differs from a complete response in that tumor shrinkage is not required, but there must never be any re-growth. A tumor is not locally controlled if there is ever any progression of that individual treated lesion. A regional failure is defined as a failure within the thorax but outside the 80% isodose volume, and a distant failure is one outside the thorax. The study was powered to demonstrate a local control rate of ≥ 50%. Pulmonary and other toxicity was scored using CTCAE v 3.0 Citation[5]. A study objective was to keep grade 3 toxicity as low as possible, and at maximum 5%.
Examination of response was made serially over time using the follow-up 3D imaging of the chest. The treated lesions were assessed by the diagnostic radiologists and three radiation oncologists on each scan. The time of disease progression included all local, regional, or distant failures. Actuarial overall survival, progression-free survival, and local control rate were defined in the usual way using the Kaplan-Meier method. There were no non-cancer related deaths.
Results
Patient characteristics and treatment planning
Between February 2001 and December 2005, 50 patients (49 evaluable, one patient did not return for follow-up) with 125 lung lesions were treated. Thirty were treated curatively and 19 palliatively. Patient ages ranged from 37 to 86 years (median 60 years), and the number of index targets treated per patient ranged from 1–5 (mean 2.6). Tumor sizes ranged from 0.3–7.7 cm in maximal diameter (median 2.1 cm) on CT. The GTV volume ranged from 0.1–125 ml (median 4.7 ml). Mean follow-up was 18.7 months (range 3.7–60.9). Most patients had undergone multiple therapies for their metastatic disease and the median time from first diagnosis of metastatic disease to referral and radiation was 14.8 months. For more details see .
Table I. Patient and treatment characteristics.
The total dose delivered to the tumor isocenter was typically 50 to 55 Gy, usually at 5 Gy per dose, but varied from 2.5 and 6.5 Gy per dose. The fractionation was determined by restrictions imposed by the normal tissue DVH as discussed in the Materials and methods section. The preferred fractionation and dose to the tumor isocenter was 50 Gy at 5 Gy/fraction (31 of 49 patients).
Thirty-seven of the 49 patients had received previous chemotherapy and most had also received chemotherapy or other cytotoxic therapy following focal irradiation. Though concurrent chemotherapy was permitted, the treating oncologists commonly held a cycle during the irradiation.
Response
The progression-free survival of the curatively treated patients at 12 and 24 months was 25% and 16%, respectively (). Based on the Kaplan-Meier method, the median time to progression was 5.8 months for curatively treated patients and 3.8 months for those treated palliatively. Thus the time to first progression was not significantly altered in those individuals with five or fewer lesions compared to patients with more lesions. The median overall survival time of the palliatively treated patients (n = 19) was decreased by 11 months compared to the curatively treated subjects (Curative: median 23.4 months [95% CI 14.5 to 31.0 months] vs. Palliative: median 12.4 months [95% CI 7.6 to 22.8 months], p < 0.05) (). Long-term survivors, free of disease, were seen in the group of patients treated with curative intent. The overall survival of the curatively treated patients (n = 30) at 12, 24, and 36 months was 71%, 38%, and 25%, respectively (). Patients on both arms were allowed to have repeated cycles of therapy as long as previously treated sites had not progressed. Thus some patients with multiple metastases were ultimately treated comprehensively.
Figure 1. Actuarial progression-free survival for patients treated with curative and palliative intent is shown. Progression-free survival for patients treated with curative intent was 25% and 16% at 12 and 24 months, respectively. The number of curative patients at risk was 15 and 7 at 12 and 24 months, respectively. Progression among subjects in either curative or palliative categories was uncommon if they achieved 15 months of well patient visits.
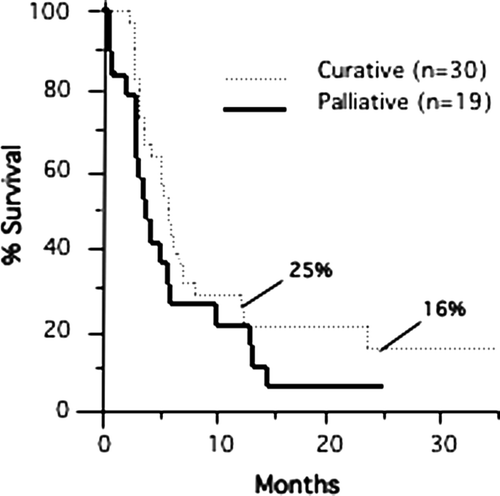
Figure 2. Actuarial overall survival for patients treated with curative and palliative intent is shown. Survival was 71%, 38%, and 25% at 12, 24, and 36 months, respectively, for patients treated with curative intent. The number of curative patients at risk was 17, 7, and 3 at 12, 24, and 36 months, respectively.
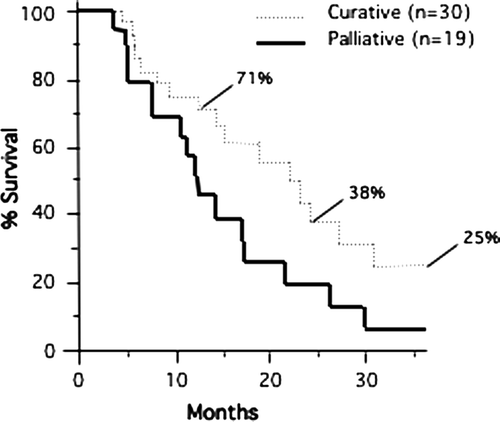
We examined for long-term progression-free survival in the whole cohort of patients so as to reduce the potential for selection bias of more favorable patients. The progression-free survival of all patients is shown by site of primary disease in . Though patient numbers are small, long-term progression-free survival is seen in a proportion of subjects independent of primary disease site. For example all six patients with progression following treatment for primary lung cancer have died, while the two living patients (23 and 33 months after treatment) remain free of progression.
Figure 3. Actuarial progression-free survival by primary tumor types (breast, colorectal, lung, and others) among all patients is shown. Long-term progression-free status was most obvious for breast and lung cancer.
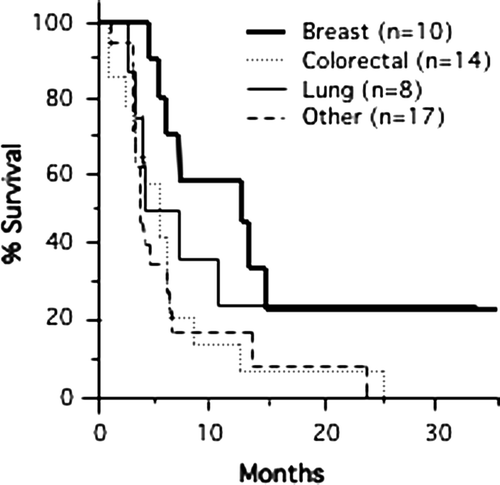
The actuarial 3-year local control rate of all 49 patients treated was 82.5±6.2% based on analysis with the Kaplan-Meier method (). The 3-year local control rate for all tumors (n = 125) was 91.0±3.2% (±1 se) (). Of the 125 lesions treated, 36 lesions (29%) disappeared completely after treatment (complete response). Thirty-two lesions (26%) decreased in maximal diameter by ≥ 30% (partial response) after treatment, and 49 lesions (39%) were stable after treatment (stable disease). Only eight of all 125 lesions treated (6%) progressed at any time after treatment. Therefore, the crude local control rate was 117 of 125 lesions (94%). Based on our original design, we considered a 50% local control rate evidence of feasibility. Thus the study met the feasibility milestone.
Figure 4. Actuarial local control rate for lesions (n = 125) and for patients (n = 49) is shown. Local failures were all seen during the first 15 months after treatment. At 15 months the local control rate for all lesions and patients was 91% and 83%, respectively. There were 20 patients at risk at 15 months. Crude local control was achieved in 117 of 125 lesions (94%). One patient had two local failures leading to local control in 42 of 49 patients.
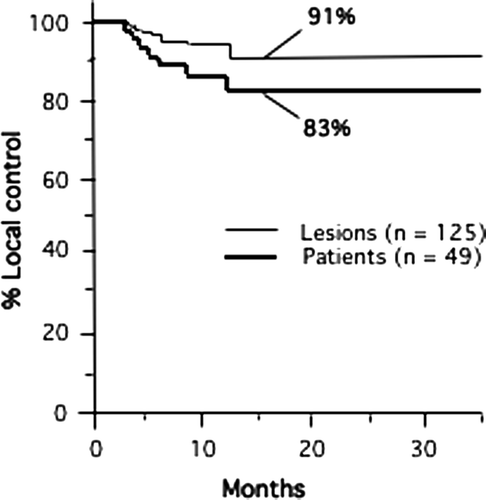
Pattern of failure
Regional failures were defined as thoracic failures outside the 80% isodose volume. Regional failure±distant failures in curatively treated patients occurred in 12 of 30 (40%), and distant failures alone occurred in seven of 30 patients (23%) treated with curative intent. There were five patients with local failures, while seven subjects (23%) are currently disease-free (median 33 months) in this patient group.
Eight of the 125 tumors had local failure. The maximum diameter of these eight lesions ranged from 1.5–3.0 cm. At the time of progression, they ranged from 1.9–4.5 cm. One patient had two lesions that progressed. Five of these seven patients who suffered local failure were treated with curatively intent. Of the eight lesions, three were pulmonary metastases from colon malignancy. The time from end of treatment to local failure of the lesions ranged from 3.1–12.2 months (). Regional and distant failures became less common with longer follow-up. New failures were rare after 15 or more months of progression-free survival.
Table II. Characteristics of the lesions that progressed (n = 8).
Toxicity
CTCAE v 3.0 grade 1 pulmonary toxicity (asymptomatic pulmonary changes) occurred in 17 (35%) of the patients, three patients (6.1%) had grade 2 complaints, and one individual had grade 3 toxicity (). The grade 3 toxicity was a non-malignant pleural effusion successfully managed with pleurocentesis and sclerosis. This patient's V10 and V20 were 21% and 4%, respectively. The mean V20 of the entire cohort of patients was 12.6%, with a range of 1–34%. Among these patients, toxicity was not clearly associated with V20. Most grade 2 toxicity was in the form of coughing, which was self-limited.
Table III. Toxicitya.
Discussion
The natural progression of cancer is generally assumed to start with a primary tumor; subsequent initial metastases can be limited to lymph nodes, but distant metastases are presumed to be multiple even at the earliest stages. Treatments are defined accordingly. Primary tumors are removed, radiation fields often include the nodal drainage regions, and metastases are treated with systemic therapy. Local treatment of metastases is used only for symptomatic tumor palliation and is delivered at lower doses than that required for sterilization. It is now possible however to offer local control through aggressive local therapies for metastases, opening the door for testing curative options for treatment of metastases, and providing an opportunity for testing the hypothesis of oligometastasis. In this study, we evaluated the potential for focal high dose hypofractionated radiation to locally control tumors that have metastasized to the thorax. Our results demonstrate a high (94%) local control for treated lesions, with a low level of significant toxicity (grade 3, 2%). Thus the approach appears robust. Similar results have been seen by a number of other institutions that have approached focal radiation by a variety of methods Citation[6–17]. Our study however was the most aggressive with regard to the number of targets chosen.
A total of 12 SBRT lung series, including 389 patients and 457 targets (excluding this study), were reviewed (). Patient numbers in these series ranged from 10–61. All series exclusively studied lesions in the thorax. The numbers of lesions treated on the studies ranged from 10–71. The mean number of targets treated per patient ranged from 1–1.5. Crude local control rates ranged from 79–95%. Median follow-up ranged from 8–36 months. Overall, acute toxicity grade 1–2 ranged from 0–100%. Acute toxicity grade 3–5 occurred in 0–8%. In our study, 49 patients with 125 lung lesions were treated, and the number of index targets treated per patient ranged from 1–5 (mean 2.6). Crude local control was 94% (117/125). Mean follow-up was 18.7 months (range 3.7–60.9). Overall, acute toxicity grade 1 occurred in 35% and grade 2 occurred in 6.1% of the 49 patients; acute toxicity grade 3 occurred in 2% (one patient).
Table IV. Published treatment concepts and results of stereotactic radiotherapy of targets in thorax.
These results suggest that very high permanent control rates can be consistently obtained by aggressive focal radiation even for subjects with multiple lesions. Ultimately, the utility of hypofractionated SBRT will be maximized by determining the needed dose and dose distribution, optimal tumor margin and imaging techniques, and necessary immobilization requirements.
Dose and fractionation
The optimal dose and fractionation can be defined as the lowest dose schedule that controls the tumor and at the same time maximally limits collateral normal tissue damage. In the Table IV, based on information in the various publications, we adjusted the dose specifications to a similar standard to allow direct comparison. Doses ranged from 15 to 94 Gy at fractionation schedules of 1 to 15 fractions. The fractionated doses were given from once per week to five days per week. There is no clear correlation between total dose, number of fractions, or fractionation schedule. In fact, crude local control was high in all studies. Thus dose might have been sufficient in all studies, with variability of control depending mostly on targeting and biological variability. Our choice of doses was based on mathematical calculations from literature searches Citation[18]. The dose was chosen to be the Tumor Control Dose 85% (TCD85). Others have suggested that higher doses are required than the dose we chose, and higher doses have been chosen for some national clinical trials Citation[19]. We had an unexpected number of local failures among patients treated with lung metastases from colorectal cancers. Thus some dose escalation is likely needed for this pathological subtype. The experience with radiosugery for metastastic brain tumors suggests that even lower doses chosen by previous investigators have high efficacy Citation[20]. Based on all the studies taken together, it is fair to say that homogeneity of dose to the target tissue is not a priority. Choosing the lowest dose that permanently controls tumor should be a very high priority for treatment of metastases, since the number of lesions that can be safely treated will depend on the integral dose received by the whole lung.
Fractionation is also a potentially important issue. Fractionation takes advantage of tumor reoxygenation and redistribution in the cell cycle. Single fraction radiation dose requirements are expected to be dominantly dependant on the hypoxic cell fraction, while with even just 2 fractions the hypoxia effects are hugely reduced Citation[21]. Theoretical estimates done by Brenner et al. Citation[22] suggest that after about 7 fractions the benefit from further fractionation is minimal. Completing a course of treatment during an interval that prevents repopulation is also important. We chose therefore to deliver treatments daily rather than three times per week or weekly, and we used 10 fractions to assure maximum reoxygenation benefits and reduce fractional dose size to normal lung. The results outperformed our initial expectations with regard to both local control and toxicity. Optimal fractionation, however, logically involves the minimum number of doses that obtain the desired effect. Thus shorter and lower dose schedules might be better for the majority of tumors, saving higher doses or more protracted schedules for tumors known to be more hypoxic or more resistant to radiation response. The latter will require advances in imaging and science, not yet available, to a priori measure radiosensitivity. Research in those fields is indicated.
Imaging
Advances in imaging and molecular diagnosis now allow the detection of subcentimeter metastases and assessment of tumor metabolic activity. Improved imaging has resulted in a natural stage migration. More “tiny” tumors are now detected, leading to an increased number of patients who are diagnosed with very early stage cancers. Likewise, easier detection of very small metastases now leads to increased diagnosis of advanced stage disease among individuals who would formerly have been considered of earlier stage. The impact of this improved imaging has dangers. For example, there is a danger of under-treatment of patients with very early metastasis (previously considered stage I). This subject will be addressed in more detail later.
Tumor targeting is extremely dependent on accurate imaging. In general, radiation oncology has focused on treating tumors and an assumed microscopic invasion margin. These margins are sometimes considered to include whole anatomic regions and/or many centimeters beyond a visible target on 3D imaging. The results seen in our studies and those of others listed in suggest that margins need not be substantial. Autopsy studies suggest that the infiltrating tumor margin around the gross nidus on CT is approximately 5 mm Citation[23] for lung metastasis. Surgical series have debated the need for nidusectomy versus lobulectomy or pneumonectomy. Recurrences after limited surgery commonly occur at the bronchial stump rather than in the circumferential margin. We have observed a few very focal recurrences just outside the 80% isodose volume. It is unclear how many are new metastases and how many might have been “marginal misses”. These lesions were not necessarily at the bronchial margin. We however do not suggest that margins be increased, but rather that imaging technologies will improve and help us properly size the treatment volume. Most studies have featured a specifically defined or implicitly applied 10 mm margin about the GTV to account for combined imaging and positioning error. Given the high rates of local control, this is clearly sufficient. It is not yet known if margins can be reduced.
Immobilization and gating
Normal physiological motion is a disadvantage of extracranial radiosurgery and hypofractionation techniques compared to intracranial radiosurgery. Achieving adequate and quality assured immobilization is a challenge. Current techniques include modeling of respiration with gating of the radiation beam, voluntary breath hold techniques, and physical pressure devices. A relatively complete review by Yin et al. Citation[24] on this subject has recently been published. All these various techniques and simple quiet respiration with no specific immobilization were used by various groups. Our approach for immobilization has been published Citation[3] and takes advantage of the natural elasticity of the lung to achieve reproducible relaxed end-exhalation breath hold. This technique was combined with biofeedback from infrared markers placed on the chest (ExacTrac®) and calibrated to the treatment planning CT scan. Each patient had at least four quality assurance CT scans to measure the maximum positioning error. It was rare for the tumor to require additional margin in order to assure that the 80% isodose volume completely covered the GTV on all treatments. Conveniently, tumors commonly shrink during the 10 treatment doses, leading to naturally increasing margins. As noted in the methods, our 80% isodose was typically 7 to 10 mm from the edge of the GTV. The falloff dose about the 80% isodose is extremely steep leading to little dose exposure of apparently uninvolved lung. Thus no V20 exceeded 34%, and the median was 10%. Typically V20 under 37% for pulmonary malignancies is judged safe by the literature and in national clinical trials in the United States Citation[25].
There are advantages and disadvantages to each of the many technologies used in the various studies. These differences are mostly theoretical since there was little obvious difference in tumor control rates among different studies. Toxicity between studies was not consistently evaluated using the CTCAE v 3.0 methods. Nevertheless, here too the various methods used over the years by various institutions are not yet demonstrably different. It is likely that differences between the methods will not be seen until more institutions attempt to treat a large number of targets per patient. Indeed margins and dose distributions need to be minimized: this will require pulmonary control techniques that are quality assured. It is not yet certain how that assurance should be ascertained, and this is a problem that must be dealt with as clinical trials are designed in the future. It is likely that some of the technologies will prove better than others.
Oligometastases and role of chemotherapy in downstaging of patients to an oligometastatic state
Improved imaging now allows us to detect tumor metastases at a size previously impossible. Patients that formerly were considered early stage therefore are now commonly upstaged, leading to changes in their primary therapy. This change is generally considered an advantage, but may in some cases result in under-treatment. Specifically, in patients upstaged from I to IV, primary therapy plus adjuvant chemotherapy might have controlled some formerly unseen metastases. Absence of the primary therapy or less aggressive palliative chemotherapy might have prevented the desired outcome.
Focal radiation is an obvious opportunity for consolidation of patients with minimal bulk metastases. Effective chemotherapy might down-stage metastatic disease to oligometastases. Evidence for this phenomenon is seen among women in this study with breast cancer who had pulmonary disease. Appropriate chemotherapy was offered to all, and most had experienced good chemotherapy responses initially. Many continued on some cytotoxic therapy for a period of time after SBRT. Recurrences during the months before SBRT were largely in the sites of initial metastatic disease, which was then targeted by our protocol. Women with breast cancer treated with curative intent enjoyed a 36% 40-month progression-free survival. While the number of subjects is small and data is preliminary, an advance of this magnitude in the care of women with metastastic breast cancer is monumental. The notion that some women with breast cancer and pulmonary metastases have long-term disease-free survival is not new. Surgical metastectomy has very similar results Citation[2] to those shown in our study. While long-term survivors are common among women with just bone disease, our patients all had thoracic tumors. Interestingly, progression, when it occurs, appears to occur early. The median progression-free survival rates were 5.8 and 3.8 months for individuals treated comprehensively or palliatively, respectively. These numbers are very similar to those expected from chemotherapy. Interestingly however, late recurrences become scarce after approximately 15 months of well patient visits. We do not believe that the patients enrolled on this study had incidentally favorable disease; indeed most had already failured chemotherapy and many had been suffering with metastatic disease for over a year before enrollment on the study. Thus we interpret the presence of disease free patients with long follow-up as evidence for oligometastasis. Proposed national studies are in development to test this question.
Tolerance and complications
In this study we commonly treated patients with multiple lesions and commonly included midline structures and the hilum. Patients were allowed to go on to additional courses of focal radiation but toxicity was continuously scored. While care was given to reduce dose to the esophagus to less than 3 Gy per dose, very little adjustment was made for lesions adherent to the left ventricle, hilum, chest wall, or vascular structures. Many patients (37/49) had received previous chemotherapy and many received chemotherapy following focal irradiation. Despite this however, toxicity was very mild. Low levels of toxicity have been seen in most studies reported in the literature. Indeed it must be stated that focal toxicity is very high, leading to a “radiation lobectomy” which is radiographically evident but clinically well tolerated. Focal radiation therefore is well tolerated and can be easily inserted into the care plan for individuals requiring chemotherapy for metastases. The short course of this radiation is consistent with currently standard radiation schedules for metastatic disease.
Future plans
Excellent local tumor control rates with low toxicity are seen with SBRT. Median survival time and progression-free survival both appear better than that achieved with standard care alone. Long-term progression-free survival can be seen in a subset of patients, supporting the oligometastasis hypothesis. While long-term survivors might be due to patient selection, the analyses include individuals with large numbers of lesions and unfavorable disease primaries. We are currently moving forward with national feasibility/phase II studies. Randomized studies to evaluate the role of SBRT may not require excessive numbers of patients if the apparent high survival gains are seen in the phase II studies.
References
- Suit H, Skates S, Taghian A, Okunieff P, Efird JT. Clinical implications of heterogeneity of tumor response to radiation therapy. Radiother Oncol 1992; 25: 251–60
- DeVita VT, Hellman S, Rosenberg SA. Cancer: Principles and Practice of Oncology5th ed. Lippincott, Philadelphia 1997; 2523–2606
- O'Dell WG, Schell MC, Reynolds D, Okunieff P. Dose broadening due to target position variability during fractionated breath-held radiation therapy. Am Assoc Phys Med 2002; 29: 1430–7
- Therasse P, Arbuck SG, Eisenhauer EA, Wanders J, Kaplan RS, Rubinstein L, et al. New guidelines to evaluate the response to treatment in solid tumors. J Natl Cancer Inst 2000; 92: 205–16
- Common Terminology Criteria for Adverse Events version 3.0 (CTCAE v 3.0), Cancer Therapy Evaluation Program 2003. Available at: http://ctep.cancer.gov/reporting/ctc.html.
- Blomgren H, Lax I, Göranson H, Kræpelien T, Nilsson B, et al. Radiosurgery for tumors in the body. Clinical experience using a new method. J Radiosurg 1998; 1: 63–74
- Uematsu M, Shioda A, Tahara K, Fukui T, Fuyumi Y, Tsumatori G, et al. Focal, high dose, and fractionated modified stereotactic radiation therapy for lung carcinoma patients. Cancer 1998; 82: 1062–70
- Wulf J, Hädinger U, Oppitz U, Thiele W, Ness-Dourdoumas R, Flentje M. Stereotactic radiotherapy of targets in lung and liver. Strahlenther Onkol 2001; 177: 645–55
- Nakagawa K, Aoki Y, Tago M, Terahara A, Ohtomo K. Megavoltage CT-assisted stereotactic radiosurgery for thoracic tumors: Original research in the treatment of thoracic neoplasms. Int J Radiat Oncol Biol Phys 2000; 48: 449–57
- Uematsu M, Shioda A, Suda A, Fukui T, Ozeki Y, Hama Y, et al. Computed tomography-guided frameless stereotactic radiotherapy for stage I non-small-cell lung cancer: A 5-year experience. Int J Radiat Oncol Biol Phys 2001; 51: 666–70
- Nagata Y, Negoro Y, Aoki T, Mizowaki T, Takayama K, Kokubo M, et al. Clinical outcomes of 3D conformal hypofractionated single high-dose radiotherapy for one or two lung tumors using a stereotactic body frame. Int J Radiat Oncol Biol Phys 2002; 52: 1041–6
- Hara R, Itami J, Kondo T, Aruga T, Abe Y, Ito M, et al. Stereotactic single high dose irradiation of lung tumors under respiratory gating. Radiother Oncol 2002; 63: 159–63
- Onimaru R, Shirato H, Shimizu S, Kitamura K, Xu B, Fukumoto S, et al. Tolerance of organs at risk in small-volume, hypofractionated, image-guided radiotherapy for primary and metastatic lung cancers. Int J Radiat Oncol Biol Phys 2003; 56: 126–35
- Hof H, Herfarth KK, Münter M, Hoess A, Motsch J, Wannenmacher M, et al. Stereotactic single-dose radiotherapy of stage I non-small-cell lung cancer (NSCLC). Int J Radiat Oncol Biol Phys 2003; 56: 335–41
- Lee S, Choi EK, Park HJ, Ahn SD, Kim JH, Kim KJ, et al. Stereotactic body frame based fractionated radiosurgery on consecutive days for primary or metastatic tumors in the lung. Lung Cancer 2003; 40: 309–15
- Timmerman RD, Papiez L, McGarry R, Likes L, DesRosiers C, Frost S, et al. Extracranial stereotactic radioablation: Result of a phase I study in medically inoperable stage I non-small cell lung cancer. Chest 2003; 124: 1946–55
- Wulf J, Haedinger U, Oppitz U, Thiele W, Mueller G, Flentje M. Stereotactic radiotherapy for primary lung cancer and pulmonary metastases: A noninvasive treatment approach in medically inoperable patients. Int J Radiat Oncol Biol Phys 2004; 60: 186–96
- Okunieff P, Morgan D, Suit H. Radiation dose-response of human tumors. Int J Radiat Oncol Biol Phys 1995; 32: 1227–37
- Timmerman RD, Choy H, Galvin JM, Michalski J, Fowler J, Johnstone D, et al. A phase II trial of Stereotactic Body Radiation Therapy (SBRT) in the treatment of patients with medically inoperable stage I/II non-small cell lung cancer, RTOG 0236,2004. RTOG protocol available online (PDF) www.rtog.org/members/protocols/0236/0236
- Varlotto JM, Flickinger JC, Niranjan A, Bhatnager A, Kondziolka D, Lundsford L. The impact of whole-brain radiation therapy on the long-term control and morbidity of patients surviving more than one year after gamma knife radiosurgery for brain metastases. Int J Radiat Oncol Biol Phys 2005; 62: 1125–32
- Suit HD, Howes AE, Hunter N. Dependence of response of a C3H mammary carcinoma to fractionated irradiation on fractionation number and intertreatment interval. Radiat Res 1977; 72: 440–54
- Hall EJ, Brenner DJ. The radiobiology of radiosurgery: Rationale for different treatment regimes for AVMs and malignancies. Int J Radiat Oncol Biol Phys 1993; 25: 381–5
- Li WL, Yu JM, Liu GH, Zhong WX, Li WW, Zhang BJ. A comparative study on radiology and pathology target volume in non-small lung cancer. Zhonghua Zhong Liu Za Zhi 2003; 25: 566–8
- Yin F-F, Das S, Kirkpatrick J, Oldham M, Wang Z, Zhou S. Physics and imaging for targeting of oligometastases. Semin Radiat Oncol 2006; 16: 85–101
- Bradley J, Graham K, Winter J, Purdy J, Komaki R, Roa W, et al. Acute and late toxicity results of RTOG 9311: A dose escalation study using 3D conformal radiation therapy in patients with inoperable non-small cell lung cancer. Proc Am Soc Thera Rad Oncol (ASTRO). Int J Radiat Oncol Biol Phys 2003; 57: 137