Abstract
The iso-effective irradiation of continuous low-dose-rate (CLDR) irradiation was compared with that of various schedules of pulsed dose rate (PDR) irradiation for cells of two established human lines, T-47D and NHIK 3025. Complete single-dose response curves were obtained for determination of parameters α and β by fitting of the linear quadratic formula. Sublethal damage repair constants µ and T1/2 were determined by split-dose recovery experiments. On basis of the acquired parameters of each cell type the relative effectiveness of the two regimens of irradiation (CLDR and PDR) was calculated by use of Fowler's radiobiological model for iso-effect irradiation for repeated fractions of dose delivered at medium dose rates. For both cell types the predicted and observed relative effectiveness was compared at low and high iso-effect levels. The results indicate that the effect of PDR irradiation predicted by Fowlers model is equal to that of CLDR irradiation for both small and large doses with T-47D cells. With NHIK 3025 cells PDR irradiation induces a larger effect than predicted by the model for small doses, while it induces the predicted effect for high doses. The underlying cause of this difference is unclear, but cell-cycle parameters, like G2-accumulation is tested and found to be the same for the two cell lines.
Traditionally, brachyteraphy has been clinically attractive in treating tumors that are accessible for radioactive implants. Such treatments can be achieved using a variety of dose rates (DR); ranging from low dose rate (LDR) (DR < 3 Gy/h) to high dose rate (HDR) (DR > 12 Gy/h). Radiobiologically, LDR is regarded as advantageous as compared to HDR, since late effects may be less pronounced following LDR. On the other hand, HDR-therapy provides improved radiation protection of the staff, and when optimized, an improved dose distribution compared to continuous low dose rate therapy (CLDR). Pulsed dose rate (PDR) brachytherapy has emerged as a technique that takes advantage of both LDR- and HDR-modalities. Biologically it is expected to be equal to LDR-therapy. Technically PDR can more or less be performed using the existing HDR-therapy equipment.
In PDR a stronger radioactive source (about 1 Curie) is used compared to traditional LDR irradiations. The dose is delivered in a series of short exposures, each of 10–30 min, every hour or with intervals up to 4 h Citation[1], Citation[5]. The total dose and the total treatment time are the same as for LDR therapy. Only one “stepping source” is used. Computer control enables moving the source to any defined position and to control dwell time for each applicator that is implanted into or close to the tumor. In this manner tailored dose distributions can be achieved.
Based on Thames Model of Incomplete Repair (IR-model, Citation[15], Citation[19]) Fowler et al. Citation[5] have developed a radiobiological model (Appendix A1), that predicts the relative effectiveness (RE) for repeated fractions of radiation at medium dose rate. Relative effectiveness (i.e. effect of repeated fractions relative to CLDR) multiplied by the total dose equals the biological effective dose (BED), which is directly proportional to loge cell kill (Appendix A1). The α and β parameters, describing the shape of a cell survival curve, are determined from survival data fitted to the LQ-equation, whilst µ, the repair rate constant of sublethal cell damage, is determined from split-dose results fitted to Thames’ IR-model. The use of survival data achieved by PDR radiotherapy is an opportunity to investigate the validity of Fowlers model, since PDR, in many respects, can be considered as repeated fractions at low dose rate.
It is, however, possible that genetical differences between cells can play an important role when it comes to the question of whether the radiation response of cells is comparable for LDR- and PDR-irradiation. Perhaps some cell types may respond similarly to the two treatment modalities while others may respond differently. From a clinical point of view it is of importance to sort out factors that can distinguish between patients having tumor cells of the first or the second kind in this respect.
Normally the cellular radiosensitivity decreases with decreasing dose rate. A phenomenon where the radiosensitivity is increasing with decreasing dose rate over a certain range of dose-rates is termed inverse dose rate effect. The cells are allowed to progress into more radiosensitive phases of the cell cycle where they suffer more damage.
In a recent paper Mitchell et al. Citation[13] have stated that the inverse dose rate effect is not due to accumulation of premitotic cells or to cell resensitization or to any other cell cycle perturbations, but that it is merely phenomenologically and mechanistically related to the hyper-radiosensitivity seen after low doses.
In the present study we have compared the response following PDR-irradiation with that following CLDR irradiation for cells of two different human cancer lines. The results are discussed in the light of predicted radiobiological effects following irradiation based on Fowlers model. Also addressed in this paper, is whether this model is valid for cell lines that express an inverse dose rate effect at dose rates commonly used in PDR. Finally, we have investigated the effect of an accumulation of cells in a sensitive G2-phase of the cell cycle after PDR irradiation. It is believed Citation[1], Citation[5], Citation[10] that the accumulation can modify the predicted radiobiological response for the nominal dose rates used in PDR irradiations.
Methods and materials
Cell culture
Two cell lines were used; NHIK 3025 human cervical carcinoma and T-47D human breast carcinoma. The NHIK 3025 cells are non-functional with respect to p53 and pRB Citation[21], Citation[22] due to integration of HPV 18, whereas the T-47D cells are pRB-functional, but do not express functional p53 Citation[18].
The NHIK 3025 cells were grown in MEM medium with Hanks’ salts, supplemented with 15% newborn calf serum, 2% penicillin/streptomycin, 1% L-glutamine and 1% non-essential amino acids. The T-47D cells were grown in RPMI 1640, 10% foetal calf serum, 1% L-glutamine, 1% penicillin/streptomycin and 0.2% insulin. The cells were cultured in monolayers attached to 75 cm2 Nunclon tissue culture flasks with 15 ml growth medium under a gas mixture of air and 5% CO2. The cells were incubated at 37°C. A more detailed description of the technical cell procedures is described earlier by Furre et al. Citation[6].
Irradiation
HDR split dose irradiation was performed using a linear accelerator operating at 4 MV (Clinac 600 C, Varian, USA) in order to establish the repair constant, µ. The dose rate to the cells was 1 Gy/min. The time interval between the split-dose fractions varied between 0 (single dose) and 5 h. For the T-47D cells split-doses of 2×5 Gy and for the NHIK 3025 cells 2×4.5 Gy, due to higher radio sensitivity, were used.
CLDR and PDR irradiations were carried out using a 60-Cobalt source (Mobaltron, TEM) with a retrofitted filter system that reduced the dose rate to 0.33–1.0 Gy/h. During irradiation the cell flasks were kept submerged in a water bath by lead blocks. A heater and a circulation pump were provided to maintain a stable temperature of 37°C.
A computer with a Lab-PC card and a self-constructed interface was used to control the dose delivered by the 60-Cobalt source, generating pulses with appropriate durations and frequencies. The dose was measured prior to each experiment using a minimum of 18 thermoluminescent dosimeters (Harshaw) placed evenly over the radiation field.
The CLDR irradiations were performed at mainly two different dose rates, 0.33–0.37 Gy/h and 0.86–0.94 Gy/h. All pulsed dose-rate irradiation sequences as well as the continuous irradiation dose-rates are listed in for both cell types.
Table I. List of irradiation schedules studied for NHIK 3025 and T-47D cells. CLDR was given with 2 dose rates while PDR was given with 2 different dose rates during pulses combined with pulse separations of 1 h or 4 h respectively, given in a total of 44 or 12 pulses.
Note that PDR irradiations were performed with such sequencing that the total dose delivered per hour over-all time was the same as that delivered per hour with CLDR irradiations. A total of 4 PDR pulse regimens were used; two based on the delivery of one pulse each hour with pulse durations of viz. 6.2 min and 13.8 min and two based on delivery of one pulse each 4 h with pulse durations of viz. 24.7 min and 55.2 min ().
Cell survival
Cell survival was determined by counting the number of macroscopically visible colonies following a standard procedure for seeding of single cells Citation[16]. Seeding of control cells made it possible to calculate the plating efficiency, i.e. how many viable cells that were able to form colonies in the asynchronous cell population. Prior to the irradiations the cells were investigated by multiplicity counting to correct for the number of colonies that are not formed by single cells. Immediately following irradiation, cells were removed from flasks with trypsin, diluted and seeded as single cells into tissue culture dishes for survival assay. After 2–3 weeks incubation, colonies were stained with methylene blue color and counted. Colonies having more than 40 cells were scored. The data are presented as survival curves where the log of the surviving fraction is plotted against the radiation dose Citation[11].
Data analysis
Survival data for cells following acute irradiations were fitted to the linear-quadratic (LQ) model Citation[2], Citation[8]. The survival data for cells receiving split dose irradiations were fitted to the Incomplete Repair Model (Equation 1) Citation[15], Citation[19].1 Here d1 and d2 are the two split doses delivered at a time interval t, µ is the repair constant for the sublethal damage (SLD), α and β are parameters determined by the LQ-formula.
Statistical comparisons between the results of CLDR exposures and the PDR exposures were performed by comparing the results of single-dose head-to-head of CLDR curves to the corresponding PDR results. A Student t-test was performed to investigate whether surviving fractions were statistically different; a level of significance of 0.05 was used.
Mathematical model
Based on Dale‘s complex equation Citation[4] for repeated fractions at low dose rate, Fowler Citation[5] published a formalism that predicts the biological effective dose (BED) after PDR irradiation relative to a CLDR regime, while keeping the overall dose and time equal. The radiobiological parameters of this formalism, the α/β ratio and the rate constant for repair of sublethal damage µ, were calculated from acute irradiation experiments and split dose irradiations for the two cell lines (). The calculated parameters were applied to the Fowlers equation, and relative effectiveness (RE) was plotted as a function of the dose rate. RE was found as BED divided by the total dose, D. The standard errors, SE, for each of values α, β and µ as decided in single- and split-dose experiments, were incorporated into the RE equation (Equation A1) by the relation:2 and hence the ratio between relative effectiveness RE for CLDR versus PDR by the relation:
3
Table II. The radiobiological parameters α and β Citation[6] and µ and T1/2 (from the present data) as calculated from single- and split dose irradiations of T47D and NHIK 3025 cells. The experimental data were fit to the IR-model with one or two unknown variables (2. 1) A graphical solution was calculated by deciding the time to reach half the split dose ratio (SDR).
Flow cytometry
Flow cytometric DNA-measurements were performed to investigate the progression of cells through the cell cycle as a function of dose and PDR pulse patterns. That is; the cell- cycle distribution, represented by G1, S and G2 (no mitoses), were determined on the basis of the DNA-content in the isolated cell nuclei. Four samples were analysed, representing 0, 5, 10 and 15 Gy corresponding to 0, 15, 30 and 45 h of irradiation. The flow cytometry procedure was the same as presented by Vindløv Citation[20].
Results
In A and B respectively, cell survival curves are shown for T-47D and NHIK 3025 cells after CLDR (0.37 or 0.94 Gy/h for T-47D cells and 0.33 or 0.86 Gy/h for NHIK 3025 cells) as well as all the investigated PDR combinations described in . Total doses were varied from 0 to 19 Gy resulting in a cell kill up to three logs for the T-47D cells and six logs for the NHIK 3025 cells. A straight line was fitted to each data set in A while the initial and final parts of the data sets in B were fitted with separate straight lines.
Figure 1. (A) Cell survival curves after CLDR- and PDR irradiations of T-47D cells). When symbols lack error bars it is because the bar does not exceed the symbol. (B) Cell survival curves after CLDR- and PDR irradiations of and NHIK 3025 cells. When symbols lack error bars it is because the bar does not exceed the symbol.
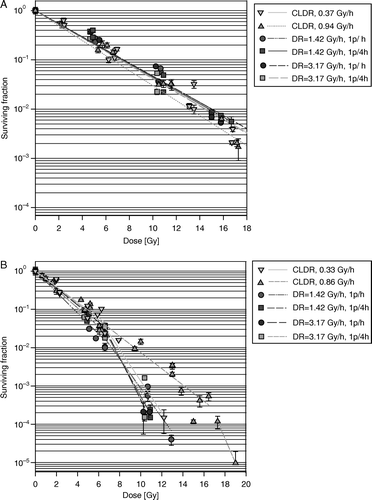
The results from the split-dose experiments are presented in and . The split-dose ratio is the ratio between the surviving fraction as measured following irradiation with two doses split with some time for repair following the first fraction and a single radiation with a dose equal to the sum of the two split doses. The split-dose ratio (SDR), show a rapid initial increase with increasing time between the two exposures, for time intervals shorter than 2 h. For longer times between dose fractions the curves reach a plateau. The plotted curves represent fits of the Thames IR-model, formula (1), with values for α and β taken from the study of Furre et al. (1999) Citation[6]. The radiobiological parameters α and β Citation[6] and µ and T1/2 (from the present data) as calculated are presented in .
Figure 2. Split dose ratio as a function of the repair interval between two doses (5 Gy + 5 Gy) for T-47D cells. Dose rate is 1 Gy/min. The plotted curves represent fits of the Thames IR-model, formula (1), with values for α and β taken from the study of Furre et al. (1999) Citation[6]. When symbols lack error bars it is because the bar does not exceed the symbol.
![Figure 2. Split dose ratio as a function of the repair interval between two doses (5 Gy + 5 Gy) for T-47D cells. Dose rate is 1 Gy/min. The plotted curves represent fits of the Thames IR-model, formula (1), with values for α and β taken from the study of Furre et al. (1999) Citation[6]. When symbols lack error bars it is because the bar does not exceed the symbol.](/cms/asset/fdba93e9-9507-4325-92b9-7eaef4f01690/ionc_a_197799_f0002_b.gif)
Figure 3. Split dose ratio as a function of the repair interval between two doses (5 Gy + 5 Gy) for NHIK 3025 cells. Dose rate is 1 Gy/min. The plotted curves represent fits of the Thames IR-model, formula (1), with values for α and β taken from the study of Furre et al. (1999) Citation[6]. When symbols lack error bars it is because the bar does not exceed the symbol.
![Figure 3. Split dose ratio as a function of the repair interval between two doses (5 Gy + 5 Gy) for NHIK 3025 cells. Dose rate is 1 Gy/min. The plotted curves represent fits of the Thames IR-model, formula (1), with values for α and β taken from the study of Furre et al. (1999) Citation[6]. When symbols lack error bars it is because the bar does not exceed the symbol.](/cms/asset/4f5229d6-4c3c-425b-858b-a6b1c5e305f1/ionc_a_197799_f0003_b.gif)
Based on the calculated parameters in , the model of Fowler (Equation A1) predicted an increase in relative effectiveness of a maximum of 6% (T-47D) and 5% (NHIK 3025) respectively for PDR irradiations given every fourth hour and for a dose rate of 3.2 Gy/h. The calculated biological effect was only influenced to a minor extent by the variation of µ-, α- and β-values that were considered. For the T-47D cells no significant difference was found when predicted PDR (relative effectiveness) were compared to the experimental results after PDR irradiation.
In the different experiments the cell batches sometimes received different doses as can be observed from the CLDR-figures. In order to compare the effect of different radiation regimes we therefore chose to use iso-effect levels as the comparing standard. and present the observed ratios between iso-effective radiation for CLDR- and PDR regimes at three different surviving fractions (closed symbols) for the two cell lines. There is one panel for each PDR-regimen. Deviation from the line where the ratio = 1 means that 1 Gy physical dose of CLDR and 1 Gy physical dose of the relevant PDR regiment are not iso effective.
Figure 4. Ratio between iso-effective doses for CLDR- (0.37 Gy/h) versus PDR irradiations as a function of the iso-effect level for T-47D cells. Closed /open symbols represent observed/predicted responses respectively. Referred to , A = PDR1, B = PDR2, C = PDR3 and D = PDR4. The iso- effect levels represent a surviving fraction of 0.2, 0.04 and 0.01, corresponding to the effect of 5, 10 and 15 Gy respectively.
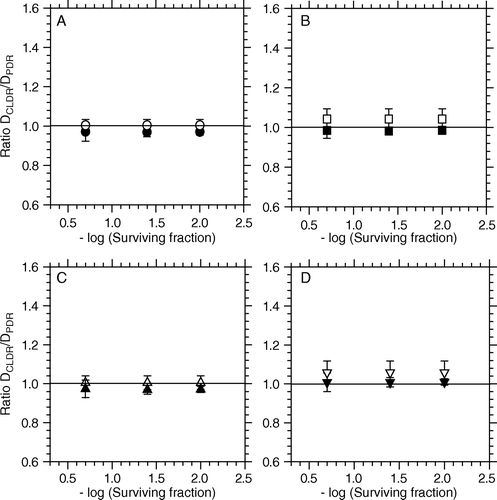
Figure 5. Ratio between iso-effective doses for CLDR- (0.33 Gy/h) and PDR irradiations as a function of the iso-effect level for NHIK 3025 cells. Closed /open symbols represent observed/predicted responses respectively. Referred to , A = PDR1, B = PDR2, C = PDR3 and D = PDR4. The iso-effect levels represent a surviving fraction of 0.06 and 0.001, corresponding to the effect of 5 and 10 Gy respectively.
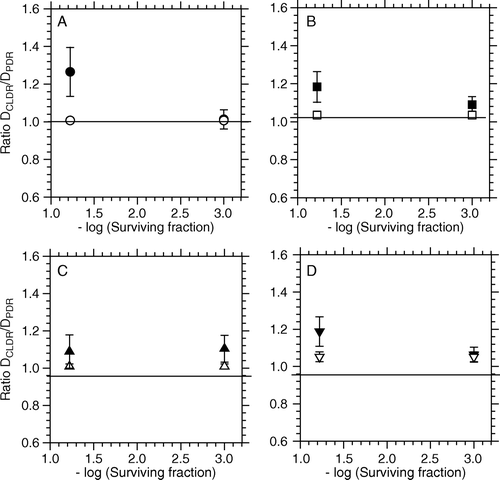
For comparison, these data are plotted together with the calculated levels of relative effectiveness as based on Fowlers predictive model (open symbols). According to the model Citation[5] the predicted values can be considered as CLDR/PDR dose ratios, in which the relative effectiveness (RE) is unity for a dose rate in the pulse equal to that in CLDR (one continuous PDR pulse). shows iso-effective dose estimates at the three different dose levels chosen for CLDR as well as all combinations of PDR studied. In the right column of the table is shown the p-values of a two-sided t-test, testing the hypothesis DCLDR=DPDR for each PDR-regimen.
Table III. Iso-effective dose estimates at the different dose levels chosen for CLDR as well as all combinations of the PDR parameters: Time between pulses, pulse dose rate and total dose, as studied in the PDR-regimes. P-values were calculated with the two-sided t-test deciding the probability of the observed and -calculated dose ratios in and not being statistical different. A p-value ≥0.05 was used to indicate that the dose ratios were not statistically different (at a probability of 95%).
For the T-47D cells the iso-effect levels used represent cell surviving fractions of 0.2, 0.04 and 0.01, corresponding to the chosen total doses of 5, 10 and 15 Gy respectively (see A). For these cells the model predicts CLDR/PDR isoeffective dose ratios that should be constant with variable surviving fraction (i.e. it predicts dose independent iso-effective dose-ratios). The data indicate that these predictions hold very well for these cells. There was no significant difference between iso-effective dose ratios (i.e. DCLDR/DPDR) whether the PDR pulses were given once every hour or once every fourth hour for dose-rates during pulses up to 3.2 Gy/h (, p ≥ 0.24).
For NHIK 3025 cells the iso-effect levels of 0.06 and 0.001 respectively represent the cell kill following 5 and 10 Gy doses. From the observed dose ratio (DCLDR/DPDR) is greater for a 5 Gy dose than for a 10 Gy dose, which is different from the prediction made by Fowlers model. Also, the difference in observed iso-effective dose-ratios following CLDR as compared to PDR, was greater for NHIK 3025 cells than for T-47D cells. The difference between iso-effective PDR and CLDR doses was found to be approximately 20% (, p ≥ 0.034) at a surviving fraction corresponding to a CLDR dose of 5 Gy, and approximately 12% at a surviving fraction corresponding to a CLDR dose of 10 Gy (p ≥ 0.10).
Flow cytometric DNA-measurements ( and ) revealed that cells of both lines accumulated in the G2 phase with increasing dose. For T-47D cells the fractions of cells in G1 and G2 phases were respectively about 20% and 70% after a PDR treatment time of 45 h, corresponding to a total dose of 15 Gy (D). For these cells the data can not be taken as an absolute proof that the G2-arrest is complete, since neither the G1 nor the S fraction has reached a stable level close to zero after 45 h PDR irradiation. However, these cells have a median cell-cycle time of 37 h Citation[18] (compared to 20 h for NHIK 3025 cells) and therefore the rate of G2-accumulation is close to what would be expected if the G2-arrest was complete. The survival curves for the CLDR- and PDR irradiations indicated no inverse dose rate effect for the T-47D cells for the dose and dose rates investigated, in line with our recent report Citation[7].
Figure 6. Flow cytometric measurements of cell nuclei illustrating the fraction of cells in G1, S and G2 phase for T-47D cells as a function of the total irradiation time when irradiated by 4 different PDR schedules. A) 1.42 Gy/h and 1 pulse/h, B) 1.42 Gy/h and 1 pulse/4h, C) 3.17 Gy/h and 1 pulse/h, D) 3.17 Gy/h and 1 pulse/4h.
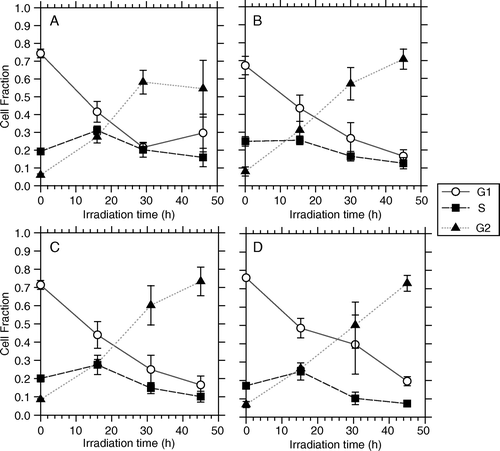
Figure 7. Flow cytometric measurements of cell nuclei illustrating the fraction of cells in G1, S and G2 phase for NHIK 3025 cells as a function of the total irradiation time when irradiated by 4 different PDR schedules. A) 1.42 Gy/h and 1 pulse/h, B) 1.42 Gy/h and 1 pulse/4h, C) 3.17 Gy/h and 1 pulse/h, D) 3.17 Gy/h and 1 pulse/4h.
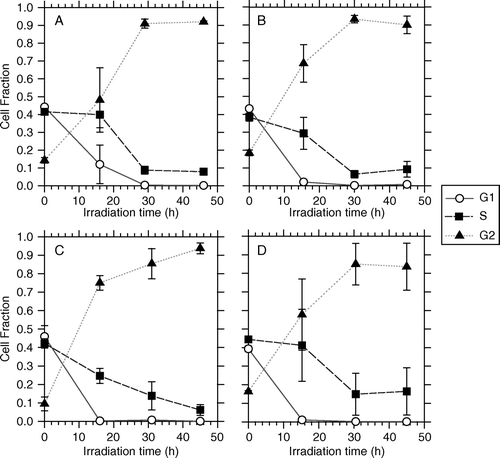
For NHIK 3025 cells the data clearly indicate that the G2-arrest is complete since the G2 fraction increases up to 90% during a treatment period of 45 h corresponding to a total radiation dose of 15 Gy () resulting in a complete lack of cells escaping to mitosis during the treatment period and no recruitment into the subsequent G1. Since cells originally in G1 continue into S-phase, G1 is left empty 45 h after irradiation. The survival curves for the CLDR- and the PDR experiments (B) indicate that the inverse dose rate effect occurs after a treatment time of approximately 20 h, corresponding to a radiation dose of 6.6 Gy for these cells Citation[6].
Discussion
The theoretical model of Fowler suggests that PDR with a pulse of 10–15 min every hour would yield equal biological effect as a CLDR irradiation with dose rate between 0.5 and 0.6 Gy/h. In the present study, we chose a mean PDR dose rate below this range (0.33 Gy/h) in order to extend treatment time and to include an eventual participation of the inverse dose rate effect that is expressed by the NHIK 3025 cells Citation[6].
Our results for the T-47D cells (A) demonstrated no difference in radiobiological effect between CLDR- and PDR irradiation using a dose rate in the pulse of 3.17 Gy/h, a mean dose rate of 0.33 Gy/h, and doses per pulse up to 1.25 Gy given every 4 h. This observation was valid for all doses investigated in these experiments, and thus, T-47D cells respond as predicted by Fowlers theoretical model.
As indicated by B NHIK 3025 cells seem to respond equally to CLDR- and PDR irradiation when the mean dose rates were similar and at the 0.3 Gy/h level (i.e. CLDR: 0.37 Gy/h and PDR: 0.33 Gy/h). Thus, B clearly shows that the inverse dose rate effect that was previously demonstrated for cells of this line using CLDR irradiation (Furre et al. 1999) is also valid following PDR irradiation. In line with this finding we see from that G2-accumulation is as efficient with PDR-irradiation as it was previously shown to be with CLDR-irradiation (Furre et al. 1999). We therefore believe that the down-bending of the dose response curves after PDR irradiation for doses above 6 Gy (B) reflects the higher response of G2-cells compared to cells in other phases of the cell cycle Citation[14].
Still, the iso-effect plots in suggest that there is a difference in response to CLDR and PDR for NHIK 3025 cells at the level of 5 Gy total dose. At this dose level the cells express a higher relative effectiveness when radiation is given by PDR as compared to CLDR. No such difference between the two treatment modalities were seen following 10 Gy. In fact, following 5 Gy total dose the presumably least effective of the PDR regimes (1.4 Gy/h, 1 pulse every hour) results in the highest biological response compared to the other used PDR regimes. This enhanced PDR effect can not be explained by for example an increased inverse dose rate effect during PDR- than during CLDR irradiation for the NHIK 3025 cells, since such an effect does not occur at total doses beneath 6.6 Gy (corresponding to 20 h treatment time, see B)). Besides, from , little or no G2-accumulation has taken place after 4 h of treatment, corresponding to 5 Gy total dose.
We have considered the possibility that NHIK 3025 cells could be hypersensitive to small single doses of 0.33 Gy, but that induced radio resistance (IRR) appears after about 15 dose fractions, corresponding to 5 Gy total dose Citation[9]. Repeated small doses of irradiation, represented by PDR irradiations, would then yield a greater biological effect than a CLDR or PDR irradiation using increased pulse-doses. However, unpublished data in our lab shows that NHIK 3025 cells have no hypersensitivity at low doses, while T-47D cells show marked hypersensitivity (Edin, unpublished data). Thus, this is not the explanation since one would in that case rather have expected increased PDR compared to CLDR-effect in T-47D cells and not in NHIK 3025 cells.
The time of appearance of the inverse dose rate effect following the start of irradiation (after about 20 h, B), coincide with the time of maximum G2 accumulation (). Moreover, the estimated median cell-cycle time, Tc, also coincides with this 20 h delay, suggesting that the inverse dose rate effect (for the dose rates investigated), is purely a function of time. This observation was confirmed in CLDR experiments performed by Furre et al. Citation[6] suggesting that the inverse dose rate effect may be present even at higher dose rates (0.89 Gy/h). However, the mechanism of inversed dose rate effect may be more complicated since this phenomenon has even been observed without G2-arrest Citation[22]. It is possible that some of this apparent contradiction might be resolved by considering that acute low-dose hypersensitivity is related to cells irradiated in G2 failing to undergo an early checkpoint arrest as shown by Marples et al. Citation[12].
In order to seek an explanation to this finding we therefore have to consider differences between the cell types used in this study. Reports showing parallel data have been published. Chen et al. Citation[3] discovered a similar equivalence in response between CLDR and PDR irradiations for T-47D cells as we have presented here. There were no statistical difference between a CLDR regime at 0.6 Gy/h and a PDR regime with a pulse dose of 2.4 Gy/h every 4th hour and at a maximum dose rate of 9.48 Gy/h. Chen also irradiated HTB 35 (human cervix carcinoma) and MCF7 cells (human mammae carcinoma) with the same PDR protocols. For a pulse every 2nd or 3rd hour (DR 9.48 Gy/h) the two latter cell lines showed an enhanced response to the PDR irradiation compared to T-47D cells.
Keilholz et al. Citation[10] irradiated Chinese hamster fibroblast cells (HY B14 FAF 28) in a brachytherapy model. According to Keilholz the α/β-ratio of these cells is approximately 11.7 Gy, comparable to our NHIK 3025 cells. The cell doubling time of HYB14FAF28 cells is 12–14 h while it is about 20 h for NHIK 3025 cells. For pulses delivered every hour Keilholz found no difference in cell survival for a pulse dose of 1 Gy whether the dose rate in the pulse was 1.15, 2 or 3 Gy/h. The average dose rate was 1 Gy/h. Neither was there any statistical difference between the biological effect after CLDR irradiation of 0.5 Gy/h and PDR using a pulse dose of 1 Gy given every hour or every second hour. Increasing the pulse dose to 2.5 Gy yielded no statistical difference from CLDR (0.5 Gy/h), but a further increase in pulse dose to 5 Gy resulted in an enhanced biological effect.
Pomp et al. Citation[17] irradiated cells derived from a human bladder carcinoma (RT 112) and a human neuroblastoma (HX 142) with various PDR regimes. They compared the observed survival to the predicted survival derived by Fowlers PDR model. No statistical differences were found for the RT 112 cells, whereas the HX 142 cells showed a much stronger effect for pulsed HDR regimes than predicted. One possible explanation for this result was that the HX 142 cells have biexponential repair kinetics.
In summary, PDR induces a greater effect than predicted for some, but not all cell lines. For T-47D cells various reports agree that iso-effective DPDR/DCLDR – ratios do not vary with varying PDR-regimes. The reason is not known, but genetical differences between different cell types may well play a role.
In the present study we have seen a difference in relative response to PDR compared to CLDR in NHIK 3025 cells and not in T-47D cells. Which genetical or other difference between the cell types that is the cause of the difference in response to PDR is not presently known, but is a matter that should be looked into in the future.
Acknowledgements
The skilful technical assistance of Charlotte Borka is gratefully acknowledged. The present study was supported by The Norwegian Cancer Society.
References
- Brenner DJ, Hall EJ. Conditions for the equivalence of continuous to pulsed low dose rate brachytherapy. Int J Radiation Oncol Biol Phys 1991; 20: 181–90
- Chadwick KH, Leenhouts HP. A molecular theory of cell survival. Phys Med Biol 1973; 18: 7887
- Chen C-Z, Huang Y, Hall E, Brenner DJ. Pulsed brachytherapy as a substitute for continuous low dose rate: An in vitro study with human carcinoma cells. Int J Radiat Oncol Biol Phys 1997; 37: 137–43
- Dale RG, Huczkowski J, Trott KR. Possible dose rate dependence of recovery kinetics as deduced from allmenn preliminary analysis of the effects of fractionated irradiations at varying dose rates. Brit J Radiol 1988; 61: 153–7
- Fowler J, Mount M. Pulsed brachytherapy: The conditions for no significant loss of therapeutic ratio compared with traditional low dose rate brachytherapy. Int J Radiat Oncol Biol Phys 1992; 23: 661–9
- Furre T, Koritzinsky M, Olsen DR, Pettersen EO. Inverse dose-rate effect due to pre-mitotic accumulation during continuous low dose rate irradiation of cervix carcinoma cells. Int J Radiat Biol 1999; 75: 699–707
- Furre T, Eggen I, Koritzinsky M, Åmellem Ø, Pettersen EO. Lack of inverse dose rate effect and binding of the retinoblastoma gene product in the nucleus of human cancer T-47D cells arrested in the G2 by ionizing radiation. Int J Radiat Biol 2003; 79: 413–22
- Hall EJ. Radiobiology for the radiobiologist4th ed. JB Lippingcott Co. 1994
- Joiner MC, Marples B. Does induced repair determine the lethal effects of very low radiation doses? In: Radiation Science of Molecules Mice and Men. Denekamp J, Hirst DG. Brit J Radiol 1992;(Suppl 24):74–8.
- Keilholz L, Seegenschmiedt MH, Lotter M, Martus P, Schulz-Wendtland R, Sauer R. Effect of dose per pulse, pulse duration, and total exposure time of pulsed dose rate brachytherapy: Results of an in vitro model. J Brachyther Int 1998; 14: 59–69
- Lea DE. Actions of radiations on living cells. University Press, Cambridge 1942
- Marples B, Wouters BG, Joiner MC. An association between the radiation-induced arrest of G2-phase cells and low-dose hyper-sensitivity: A plausible underlying mechanism?. Radiat Res 2003; 160: 38–45
- Mitchell CR, Folkard M, Joiner MC. Effects of exposure to low-dose-rate 60Co gamma rays on human tumor cells in vitro. Radiat Res 2002; 158: 311–8
- Mitchell JB, Bedford JS, Bailey SM. Dose-rate effects on the cell cycle and survival of S3 HeLa and V79 cells. Radiat Res 1979; 79: 520–36
- Oliver R. A comparison of the effects of acute and protracted gamma-radiation on growth of seedlings of Vicia faba. Part II. Theoretical calculations. Int J Radiat Biol 1964; 8: 475–88
- Pettersen EO, Wang H. Radiation-modifying effect of oxygen in synchronized cells pre-treated with acute or prolonged hypoxia. Int J Radiat Biol 1996; 70: 319–26
- Pomp J, Woudstra EC, Kampinga HH. Pulsed-dose-rate and low-dose-rate brachytherapy: Comparison of sparing effects in cells of a radiosensitive and a radioresistant cell line. Radiat Res 1999; 151: 449–53
- Stokke T, Erikstein BK, Smedshammer L, Boye E, Steen HB. The retinoblastoma gene product is bound in the nucleus in early G1 phase. Exptl Cell Res 1993; 204: 147–55
- Thames HD, Withers HR, Peters LJ. Tissue repair capacity and repair kinetics deduced from multifractionated or continuous irradiation regimens with incomplete repair. Br J Cancer 1984; 49(Suppl VI)263–9
- Vindeløv LL, Christensen IJ, Nissen NI. A detergent-trypsin method for the preparation of nuclei for flow cytometric DNA analysis. Cytometry 1983; 3: 323–7
- Åmellem Ø, Stokke T, Sandvik JA, Pettersen EO. The retinoblastoma gene product is reversibly dephosphorylated and bound in the nucleus in S and G2 phases during hypoxic stress. Exptl Cell Res 1996; 227: 106–15
- Åmellem Ø, Sandvik JA, Stokke T, Pettersen EO. The retinoblastoma protein-associated cell cycle arrest in S-phase under moderate hypoxia is disrupted in cells expressing HPV18 E7 oncoprotein. Br J Cancer 1998; 77: 862–72
Appendix A
The IR-model by Thames Citation[19] is used as a basis for a model that predicts the relative effectiveness (RE) for repeated fractions at low dose rate. RE multiplied by the total dose equals the biological effective dose (BED), which is directly proportional to loge cell kill. One long, continuous dose pulse is comparative to CLDR, which is the gold standard of the Equation A1. Applying the LQ-formalism, α and β are radiobiological parameters describing the shape of the survival curve for an irradiated cell culture. The repair constant µ, describing the repair rate of sublethal damage (SLD), can be calculated performing split dose irradiations of the same cell culture:A1 R = dose rate, T = duration of each pulse, N = number of pulses
K = exp(−µX), where X is the period without radiation between pulses