Abstract
Gene therapy with adenoviral vectors is a promising new approach for the treatment of refractory advanced breast cancer. Strategies to restrict adenoviral-mediated therapeutic gene expression are important to avoid harming normal cells. Fatty acid synthase (FAS) is overexpressed in several human cancers. FAS is highly expressed in infiltrating breast cancer tissue, and always associated with malignant phenotypes and poor prognosis. In this study, expression of the FAS was evaluated in three breast cancer cell lines. A 680 bp-FAS promoter was cloned and its transcriptional activity was analyzed in breast cancer cell lines. We made a recombinant adenovirus construct carrying herpes simplex virus thymidine kinase (HSV-TK) driven by human FAS promoter (Ad-FAS-TK) and analyzed its target cytotoxicity in vitro and in vivo against human breast cancer cells combined with prodrug ganciclovir (GCV). The results show that the expression of FAS varies in the three breast cancer cell lines examined (respectively, SK-Br3>MCF-7>MDA-MB-231), but FAS promoter can initiate relative high transcriptional activities in all three kinds of cancer cells while little in normal fibroblast cells. Furthermore, FAS promoter can drive the therapeutic gene in a wider range of human breast cancers than cerbB2 promoter and exhibit a stronger activity than midkine (MK) promoter. Combination of Ad-FAS-TK and GCV treatment exhibited strong-targeted cytotoxic effect on breast cancer cells but showed little activity in normal fibroblast cells. The tumorigenic capability of breast cancer cells treated with Ad-FAS-TK/GCV was completely inhibited in vitro and in vivo assays. In conclusion, adenoviral-mediated suicide gene therapy controlled by tumor associated-FAS promoter can induce specific cytotoxic effect on human breast cancer cells in vitro and in vivo. So it is a promising target for the development of gene therapy against breast cancers.
Breast cancer is the second-leading cause of cancer death and morbidity for women in the world. It is estimated that 1 200 000 new cases appear annually, representing 18% of all female cancers and causing the death of 50 000 patients in the globe. Although advances in early detection and treatment have led to a decline in mortality, the survival rate for patients with advanced-stage breast cancer is still very low Citation[1]. Consequently, there is still a great need to identify and validate new molecular targets for anti-breast cancer therapy.
Tumor specific promoter controlled adenovirus carrying therapeutic gene therapy strategies have been postulated as a promising avenue for cancer therapy, for it can selectively target cancer cells in a tumor specific manner with little effect on normal cells Citation[2]. As gene therapy strategies have been limited by the inefficient gene delivery systems, adenovirus-mediated suicide gene therapy with HSV-TK (herpes simplex virus thymidine kinase)/GCV (ganciclovir) is an available approach for cancer treatment. The efficacy of HSV-TK/GCV can be augmented by the bystander effect which overcomes the limitation of gene transduction Citation[3]. Selection of an ideal tumor specific promoter is crucial in this strategy. For breast cancer gene therapy, a few promoters have already been used to drive the expression of therapeutic genes such as MUC1 promoter Citation[4], cerbB2 promoter Citation[5], MK (midkine) promoter Citation[6], human lactalbumin Citation[7] and heparanase promoter Citation[8]. But the transcriptional activities of the promoters in different breast cancer cells vary greatly. They can target cells expressing the antigens efficiently while show little activity in negative-expressed cells. The study aimed to identify a tumor-associated promoter for gene therapy which could efficiently target different breast cancer cells.
Fatty acid synthase (FAS) is the enzyme responsible for cellular synthesis of palmitate, the precursor of long-chain non-essential fatty acids Citation[9]. A growing body of evidence indicates that FAS is overexpressed in a wide range of human cancers, such as breast Citation[10], Citation[11], ovary, prostate, colon, liver, lung and melanoma Citation[12–17], but it is rarely expressed in most normal adult tissues. FAS is linked to tumor virulence and is necessary for the malignant transformation of tumor. The up-regulation of FAS in tumors is an early and nearly universal epigenetic change and involved in the development, maintenance and enhancement of the malignant phenotype Citation[18], Citation[19]. The differential expression of FAS between normal tissues and cancer cells has led to the notion that FAS is a target for anticancer drug development. Furthermore, it has been demonstrated that FAS constitutively over-expresses with hyperactivity in a biologically aggressive subset of human breast carcinomas compared with normal human breast epithelial tissue Citation[20]. In studies of node-negative early breast cancer patients, those with high levels of FAS expression experienced both shortened disease-free and overall survival Citation[21]. Thus, FAS has been suggested as a potential therapeutic target for breast cancer. We assume the FAS promoter could be used to drive HSV-TK suicide gene in an Ad vector to selectively target breast cancer cells, with little effect on normal cells.
Materials and methods
Cells and cell culture
HEK-293, QBI-293A, NIH3T3 normal fibroblast cells, SK-Br3, MCF-7 and MDA-MB-231 breast cancer cells were obtained from the department of Medical Genetics and Developmental Biology, FMMU, China. They were routinely grown in Dulbecco's modified Eagle's medium (DMEM, Invitrogen) containing 10% heat inactivated fetal bovine serum (FBS, Hyclone), 1% L-glutamine, 50 U/ml penicillin and 50 µg/ml streptomycin in a humidified incubator at 37°C in an atmosphere of 5% CO2.
Western blotting analysis
The purified goat anti-human FAS polyclonal antibody and mouse anti-human β-actin monoclonal antibody were purchased from Santa Cruz Biotech. Western blotting kit was purchased from Roche Biotech. SK-Br3, MCF-7, MDA-MB-231 breast cancer cells and NIH3T3 fibroblast cells were scraped and lysed. Equivalent amounts of the supernatant containing the solubilized proteins were separated by SDS/PAGE on 6% gels (for FAS) or12% gels (for β-actin) and transferred to PVDF (polyvinylidine difluoride) membranes (Bio-Rad). PVDF membranes were blocked for 1 h in blocking buffer (2% non-fat milk + 0.05% Tween-20 in TBS) at room temperature (RT). Membranes were then incubated overnight at 4°C with anti-FAS (diluted 1000-fold) and anti-β-actin (diluted 500-fold) antibodies dissolved in blocking buffer. Membranes were washed with TBS containing 0.05% Tween-20 at RT. They were then incubated for 1 h with horseradish peroxidase (HRP)-conjugated anti-goat IgG or anti-mouse IgG secondary antibody (sigma) diluted 1000-fold in blocking buffer at RT. After washing three times, the membranes were exposed to HRP substrates for 5 min. Exposures to autoradiography X-ray films were between 10 s and 30 min. X-ray films were scanned.
Immunofluorescence analysis
SK-Br3, MCF-7 and MDA-MB-231 breast cancer cells grown on the tissue culture chamber slide were rinsed with PBS and then fixed in 4% paraformaldehyde for 20 min. The cells were washed three times with PBS, and blocked with a blocking buffer (1%BSA, 0.3% Triton X-100 in PBS) for 30 min, then incubated with goat anti-human FAS polyclonal antibody diluted 1:100 in blocking buffer for 1 h at 37°C. After extensive washes, the cells were incubated for 45 min with cy3-conjugated anti-goat IgG secondary antibody (sigma) diluted 1:50 in blocking buffer at 37°C. After washing, the preparations were kept in the mounting medium. As controls, cells were stained with secondary antibody alone. Control experiments should not display significant fluorescence. They were then examined using a fluorescence microscope.
Luciferase reporter plasmid construction
The pGL3 Luciferase Reporter Vector System was purchased from Promega. The human genome DNA was extracted from the cultured HEK-293 cells. The 680-bp fragment of human FAS promoter was acquired by two-step PCR amplification (TaKaRa Ex Taq, TaKaRa Biotech) from human genome DNA using the following primers: sense, 5′ TAGGTACCATCACCCTATCGCCTAGCAAC3′; anti-sense, 5′ GTCTCGAG GCTGCCGTCTCTCTGGCTC 3′. Denoted in italic and bold are the Kpn I and Xho I restriction sites. The PCR conditions were as follows: 95°C 5 min; 94°C 40 s, 52°C 40 s, 72°C 40 s×5 cycles; 94°C 40 s, 62°C 40 s, 72°C 40 s ×25 cycles; 72°C 7 min. The amplified FAS promoter was sequenced and cloned into pGL3-enhance (Multiple Cloning Region allows the insertion of exogenous promoter) to make pGL3-FAS construct. We also release the cerbB2 promoter (258bp) and MK promoter (600bp) from pGEM-Teasy-cerbB2 and pGL2-MK which were kindly presented by Dr Masatoshi Tagawa (Division of Pathology, Chiba Cancer Center Research Institute, Japan) and cloned the two promoters into pGL3-enhance to construct pGL3-cerbB2 and pGL3-MK respectively.
Luciferase assay
Sixteen to 20 h before transfection, NIH3T3, SK-Br3, MCF-7 and MDA-MB-231 cells were seeded (5×105 per well) in 6-well plates to be 70–80% confluent at the time of transfection. pGL3-FAS, pGL3-cerbB2 and pGL3-MK were transient transfected into NIH3T3, SK-Br3, MCF-7 and MDA-MB-231 cells using LipofectAMINE 2000 (Invitrogen) respectively. pGL3-control (driven by SV40 promoter) and pGL3-TK (no promoter) were used as positive and negative control respectively. pSV-β-gal was co-transfected with the test plasmids in each experiment as an internal control. Forty eight hour later, cells were lysed and the luciferase activity was determined with Luciferase Reporter Assay System (Promega). The relative luciferase activity of reporter plasmid was revised by pSV-β-gal which was measured by the absorption at A420. The transcriptional activity of each plasmid was expressed as a percentage of the SV40 promoter (pGL3-control) -mediated activity. All of the values were expressed as the mean of three independent experiments.
Construction of recombinant adenovirus vector
Replication-deficient adenoviral vector system AdEasyTM was purchased from Stratagene Biotech. The 680-bp fragment of human FAS promoter was cloned into pshuttle (no-promoter adenoviral shuttle plasmid) to make pshuttle-FAS construct. pUMVC1-TK plasmid was kindly provided by Dr Lina Prasmickaite (Department of Molecular Biosciences University of Oslo, Norway). We amplified HSV-TK gene containing rabbit β-gobin polyadenylation signal from pUMVC1-TK using the following primers: sense, 5′ ATAAGCTTGATAGCTTCACCATGG C 3′; anti-sense, 5′ GGATCCGAGCGCAGCGAGTCAGTGAG 3′. Denoted in italic and bold are the Hind III and BamHI restriction sites. The 1.2 kb fragment was subcloned downstream of pshuttle-FAS to make pshuttle-FAS-TK recombinant adenoviral shuttle construct (total length 8.5 kb) containing the complete expression cassette (FAS promoter, the HSV-TK cDNA and rabbit β-gobin polyadenylation signal).
Amplification and identification of recombinant adenovirus
Recombinant adenoviral shuttle construct pshuttle-FAS-TK was linearized with PmeI digestion and subsequently cotransfected with adenovirus bone plasmid pAdeasy-1 into Escherichia coli BJ5183 competent cells by electroporation. The correct recombinant plasmid pAd-FAS-TK was identified with the digestion of PacI. Twenty four hours before transfection, QBI-293A cells were seeded at the density of 1×106 in a 60 mm plate. More than 4 ug recombinants were linearized with PacI digestion and transfected into QBI-293A cells using LipofectAMINE 2000 to generate recombinant adenoviruses. The viruses were screened by amplify the HSV-TK gene from the viral DNA to choose the correct plaques after two or three additional runs of plaque isolations. Amplified adenovirus was purified by CsCl gradient centrifugation. Viral titer was determined by plaque assay using QBI-293A cells.
Cell viability assay
Sixteen to 20 h before infection, SK-Br3, MCF-7, MDA-MB-231 and NIH3T3 cells were plated in 96-well plates in triplicate at the density of 3 000 per well. After overnight culture, the mediums were removed and cells were infected with recombinant adenovirus at multiplicities of infection (MOI) (0, 1, 10, 50, 100) for 18 h. The infecting mediums were then replaced with fresh DMEM containing 10% FBS and graded concentrations GCV (0, 1, 10, 50, 100 µg/ml, Roche) for 3 days. MTT (3-(4, 5-dimethylthiazole-2-yl)-2, 5 diphenyltetrazolium bromide, sigma) assays were used to evaluate the survival cell percentage. Control cells without agents were cultured using the same conditions. Data presented summarize the mean of three independent experiments made in triplicate.
Tumor cell clone formation assays
For the tumorigenesis analysis in vitro, tumor cells were plated in 24-well plates in triplicate at the density of 5×104 per well. After overnight culture, the mediums were removed and cells were infected with recombinant adenovirus at the MOI of 50 for 18 h. The infecting mediums were then replaced with fresh DMEM containing 10% FBS and 10 µg/ml GCV. Twenty four hours later, tumor cells were trypsinized, counted, diluted in fresh medium, and seeded in 6-well plates at the density of 1×103cells per well. Tumor cells were incubated for 14 days to allow for colony formation and then be fixed and stained with methylene blue in 50% ethanol. The number of colonies that contained at least 50 cells was counted on triplicate dishes. Results are given as the mean±SE. Comparisons between variables were tested by ANOVA test. p < 0.05 was considered statistically significant.
Animal study
To ascertain the anti-tumor effect of recombinant adenovirus Ad-FAS-TK/GCV in vivo, nude mice were purchased from the Animal Center of Fourth Military Medical University. 5×106 SK-Br3 cells were incubated with Ad-FAS-TK (1×109 pfu/0.1ml per mouse) or Ad-EGFP (enhanced green fluorescent protein) for 1 h (the same amount as a mock control). Twenty four tumor-free nude mice were randomized into three groups, and were inoculated subcutaneous with SK-Br3 breast cancer cells pre-treated with Ad-FAS-TK, Ad-EGFP and PBS (0.1 ml per mouse as a negative control) respectively. Subsequently, 50 mg/kg of GCV was then administered into the peritoneal cavity daily over 5 consecutive days. Growth of the tumors was observed 4 weeks after inoculation. Serial changes in tumor volume were estimated twice a week from the start of the recombinant adenovirus treatment. Tumor volume was calculated according to the formula [1/2×length×width2].
Results
The expression of FAS protein in human breast cancer cells
We investigated three breast cancer cell lines for FAS protein expression with western blotting and indirect immunofluorescent method. Western blotting analysis showed that FAS was not expressed in normal fibroblast NIH3T3 cells, but was expressed in all of three breast cancer cell lines with different levels, i.e. highest in SK-Br3 cells, lowest in MDA-MB-231 cells and intermediate in MCF-7 cells (). The result was consistent with the expression levels of her2/neu oncogene in the three examined cell lines. This suggested that expression of FAS was associated with the expression of her2/neu oncogene in breast cancer cells. In regards to western blotting, we confirmed that FAS was dramatically accumulated in her2/neu (+ + +) breast cell lines but less in her2/neu (+/±) breast cancer cells. Similar results were obtained from indirect immunofluorescent analysis (). We also observed that FAS was localized primarily in the cytosol of cultured SK-Br3, MCF-7, MDA-MB-231 cells under fluorescence microscopy.
Figure 1. The expression of FAS protein in three breast cancer cell lines and normal fibroblast cells by western blotting analysis. Equivalent amounts of the supernatants were loaded to express FAS and β-actin. 6% SDS-PAGE was used in FAS protein analysis due to its large molecular. 12% SDS-PAGE was used to detect β-actin.
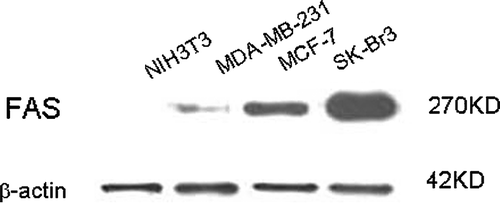
Figure 2. Micrographs of localization of FAS in breast cancer cells. Cells grown in chamber slides were fixed and stained with anti-FAS antibody by the indirect immunofluorescent method. A. SK-Br3 cells; B. MCF-7 cells; C. MDA-MB-231 cells; D. negative control. FAS localizes primarily in the cytosol of cultured SK-Br3, MCF-7 and MDA-MB-231 breast cancer cells. ×400
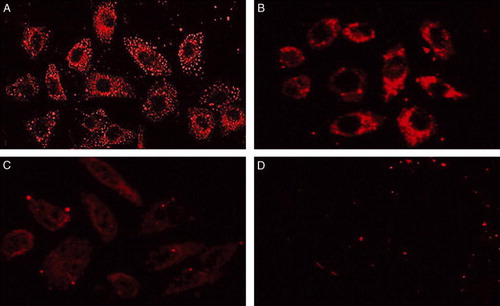
Transcriptional activity of FAS promoter compared with cerbB2 and MK promoter
A 680-bp fragment of human FAS gene promoter was amplified from human genome DNA by two-step PCR. To evaluate the tumor-specific transcriptional activity of FAS promoter, we made the pGL3-FAS luciferase reporter construct. We also made pGL3-cerbB2 and pGL3-MK constructs for comparison. The luciferase analysis results showed that the human FAS promoter exhibited high transcriptional activities in SK-Br3, MCF-7 and MDA-MB-231 breast cancer cells. Among these cell lines, FAS promoter showed the highest transcriptional activity in SK-Br3 cells that over-expressed her2/neu oncogene as has been proved by previous report. The transcriptional activities of the FAS promoter were significantly higher than those of SV40 strong promoter (pGL3-control as a positive control) in the three breast cancer cells. CerbB2 promoter showed the highest transcriptional activity in SK-Br3 cells and relatively lower activities in MCF-7 and MDA-MB-231 cells. The transcriptional activity of FAS promoter was comparable to the activity of cerbB2 promoter in SK-Br3 cells, but higher than it in MCF-7 and MDA-MB-231 breast cancer cells. MK promoter showed similar transcriptional activities in the three breast cancer lines, which was also significantly lower than those of FAS promoter. Unlike the SV40, the three tumor specific promoters (FAS, cerbB2 and MK) showed little transcriptional activities in NIH3T3 fibroblast cells. Therefore, our data revealed that the human FAS promoter can initiate high tumor-specific transcriptional activity in breast cancer cell lines and it can also drive high transcriptional activity in a wider range of breast cancer cells than cerbB2 promoter and show a stronger activity than MK promoter ().
Figure 3. Relative luciferase activities of pGL3-FAS, pGL3-cerbB2 and pGL3-MK on breast cancer cells and normal fibroblast cell. pGL3-control (SV40 promoter) and pGL3-TK (no promoter) were used as positive and negative control respectively. Indicated cells were co-transfected with pSV-β-gal. The luciferase activity was revised by ß-gal activity. The transcriptional activity of each plasmid was expressed by standardizing the SV40 promoter-mediated activity (tested with pGL3-control) as 100%. The transcriptional activities of the FAS promoter were higher than SV40 and MK promoter in all breast cancer cell lines (SK-Br3 highest), and superior to the cerbB2 promoter in the MCF-7 and 231 lines. Unlike the SV40, FAS promoter showed little transcriptional activity in NIHT3 fibroblast cell.
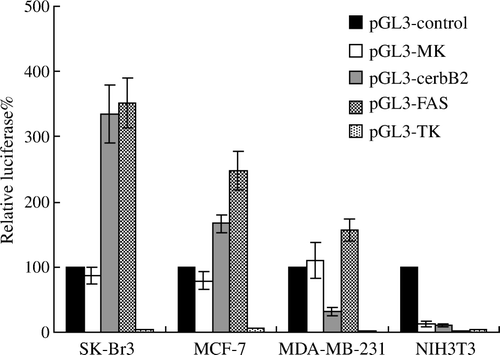
Cytotoxic effects of recombinant adenoviral HSV-TK suicide gene driven by FAS promoter combined with GCV on breast cancer cells
We tried to develop a strategy for the target gene therapy of breast cancer based on the use of a recombinant adenovirus carrying suicide gene driven by tumor-specific promoter FAS (Ad-FAS-TK). We constructed the recombinant adenoviral vector pAd-FAS-TK. Linearized pAd-FAS-TK was transfected into QBI-293A packing cells, and about 4×107 pfu/ml recombinant adenovirus was generated after 7–10 days. With replication in QBI-293A cells and CsCl gradient centrifugation, we harvested ∼1012 pfu/ml recombinant adenovirus for use. Next we examined the cytotoxic effects of Ad-FAS-TK/GCV treatment on breast cancer cells. In this study, the number of viable cells was estimated with MTT assay. For all breast cancer cell lines examined, Ad-FAS-TK/GCV showed evident cell-killing effect. Normal fibroblast cells treated with Ad-FAS-TK seemed resistant to GCV; the survival rate remained the same as before ( and ).
Figure 4. Sensitivity of breast cancer cells and normal fibroblast cells treated with Ad-FAS-TK to various concentrations of GCV. Cell viability was determined using MTT assay. In general, the cytotoxic effect was enhanced with the increase of GCV concentration. A. As control, cells were treated with GCV without infection with adenovirus. B. Cells were infected with adenovirus at the MOI of 10. When the concentration of GCV exceeded 50 µg/ml, the cytotoxic effects reach a plateau. C. As the MOI of adenovirus reached 50, the cytotoxic effects were significant even treated with low concentration of GCV (1 µg/ml).
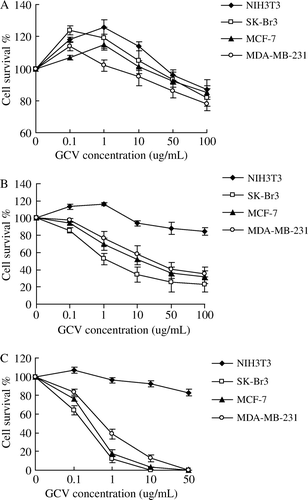
Figure 5. Selective cytotoxicity in vitro of Ad-FAS-TK/GCV towards breast cancer cells using MTT assay. NIH3T3 fibroblast cells were resistant to Ad-FAS-TK/GCV treatments. In A, cells were infected with different MOI of adenovirus followed with low concentration (1 µg/ml) of GCV while higher in B (10 µg/ml). The cytotoxic effect is dose-dependant with the MOI of the recombinant adenovirus.
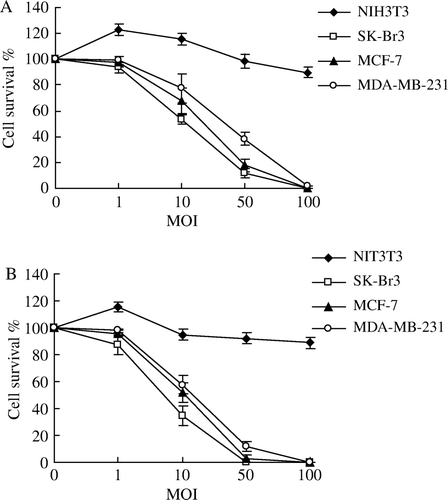
The sensitivities to GCV were all increased in the three breast cancer cells infected with relative high titer (MOI 50) of Ad-FAS-TK in a dose-dependant manner. After 3 days, almost all cells treated with MOI 50 were killed by 50 µg/ml GCV (C). When cells were treated with lower titer of virus (MOI 10), the sensitivity would reach a plateau even with the increase of GCV dose (B). Furthermore, Ad-FAS-TK-infected SK-Br3 cells were most sensitive among the three examined cell lines, and they can be completely eliminated with less GCV at the same MOI. We evaluated the correlation between MOI and killing effect. Even treated with a low concentration of GCV, the cytotoxic effects of Ad-FAS-TK were enhanced by the increase of MOI in examined breast cancer cells (). In addition, tumor cells colony formation assay was used to determine the capability of tumorigenesis of breast cancer cells after being treated with Ad-FAS-TK/GCV (). The data obtained from this assay correlated well with the results of MTT assay. The efficacy of inhibiting tumor formation by Ad-FAS-TK was most in SK-Br3 breast cancer cells as tumor colony formation was completely prevented. It is noteworthy that Ad-FAS-TK showed the same strong activities to prevent tumor colony formation in MCF-7 cells and MDA-MB-231 cells, but had little effect on normal fibroblast cells NIH3T3. In aggregate, these data demonstrated that Ad-FAS-TK achieved potent, selective cell _killing effects in all three breast cancer cell lines.
Figure 6. Clonogenic capability of SK-Br3, MCF-7 and MDA-MB-231 cells after 14 days incubation period in vitro. Cells were treated with the recombinant adenovirus (MOI: 50) or/and GCV (10µg/ml). The inhibitory effect of Ad-FAS-TK/GCV on breast cancer cells and normal fibroblast cells is evidently different (p < 0.01, ANOVA test).
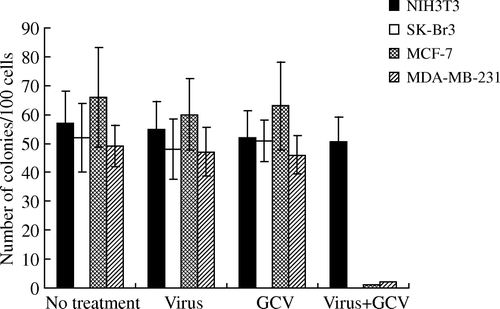
Anti-tumor effects of Ad-FAS-TK/GCV in vivo
We further examined Ad-FAS-TK mediated anti-tumor effects in vivo. SK-Br3 cells were treated with Ad-FAS-TK, Ad-EGFP or PBS and then were inoculated into nude mice. Mice injected with Ad-FAS-TK-treated SK-Br3 cells did not develop any tumor (4 weeks), whereas mice injected with Ad-EGFP-treated or PBS-treated SK-Br3 cells all developed tumors (). This result suggested that Ad-FAS-TK mediated gene therapy combined with GCV exhibit an evident anti-tumor effect in vivo.
Discussion
A number of recent studies have demonstrated surprisingly high levels of fatty acid synthase (FAS) expression in a wide variety of human malignancies including breast cancer and their precursor lesions. Treatment of cancer cells in vitro with cerulenin, a covalent FAS inhibitor, leads to cell apoptosis Citation[22]. However, cerulenin's effects are limited in vivo due to its chemical instability. Meanwhile adenovirus vectors-mediated gene therapy is becoming a promising approach for cancer treatment. Adenovirus vectors are the most highly efficient vehicles currently available for gene transfer to mammalian cells. Their ability to enter both proliferating and non-dividing cells allows in vivo gene delivery, but the wide spectrum of cell types infected by adenovirus necessitates a requirement for targeting, particularly if the transfected gene is detrimental when expressed in inappropriate tissues. Over the past decade, investigators have examined tissue/tumor specific enhancer/promoters as a means to transcriptionally target genes delivered by adenovirus vectors. An ideal promoter for cancer gene therapy should have two characteristics: relatively stronger transcriptional activity and wide target spectrums for cancer cells. In this study, we demonstrated a strategy based on the use of adenovirus carrying HSV-TK suicide gene controlled by tumor-associated FAS promoter for breast cancer gene therapy.
The cDNA sequence of FAS has an open reading frame of 7512 bp that encodes 2504 amino acids (M 272,516) Citation[23]. There are three transcription initiation sites (Ti I, Ti II, and Ti III) and two promoters (promoter I and promoter II) identified for FAS gene. The Ti I site is mapped to the beginning of the untranslated exon I and preceded by promoter I which is rich of TATA box and CAAT boxes. The Ti II and Ti III sites are located within first intron (I) and downstream of promoter II. FAS promoter I exhibits a strong transcriptional activity comparable with CMV promoter. However, promoter II is weaker and it can only reach 4–6% of promoter I activity Citation[24]. We cloned a sequence fragment of about 680-bp upstream of the Ti I site but within promoter I, which contains CAAT and TATA boxes for the experiments.
In this study, the difference of FAS expression between normal fibroblast cells and breast cancer cells provides the possibility for its targeted gene therapy. Menendez et al. Citation[25] has revealed that there exist a molecular bi-directional cross-talk between FAS and Her2/neu in cancer cells. Her2/neu over-expression stimulates FAS promoter and ultimately results in an increased endogenous fatty synthesis. On the other hand, inhibition of FAS can repress Her2/neu gene expression. Our results suggest that a high level of FAS expression is related to Her2/neu oncogene over-expression in human breast cancer cell lines. The expression of the FAS protein was dramatically up-regulated in SK-Br3 cells compared with in MCF-7 and MDA-MB-231breast cancer cells. Our luciferase activity assay showed that FAS promoter could initiate strong transcriptional activities in all three breast cancer cells, but little in normal fibroblast cells. The transcriptional activities of the FAS promoter were even higher than those of SV40 strong promoter in the three breast cancer cells. Compared with the transcriptional activity of FAS promoter in SK-Br3 cells, those in MCF-7 and MDA-MB-231 cells were relative lower. We compared the transcriptional activities of FAS promoter with cerbB2 and MK promoter in the three breast cancer cells. Her2/neu (cerbB2) is overexpressed in about 30% breast cancer patients Citation[26]. A clinical study of suicide gene therapy in breast cancer using cerbB2 promoter region has been reported Citation[27]. However, cerbB2 is only expressed (detected) in a small proportion of cancer patients, therefore its clinical application is limited. MK is a heparin-binding growth/differentiation factor which is highly expressed in many tumor tissue/cells including breast cancers. But whether it is expressed in normal breast tissues is still unclear Citation[28], Citation[29]. Our data showed FAS promoter could drive a therapeutic gene in a wider range of breast cancers than cerbB2 promoter and exhibit a stronger activity than MK promoter. Thus, FAS promoter was more suitable for breast cancer targeted gene therapy than cerbB2 and MK promoters. Then we made a construct for recombinant adenoviral suicide gene controlled by FAS promoter. Cytotoxicity assay in vitro showed that the recombinant adenovirus combined with GCV could induce evident target killing effects on breast cancer cells. Combined with a higher titer of recombinant adenovirus, even low concentration of GCV could induce complete elimination of breast cancer cells that may be associated with the bystander effect of HSV-TK suicide gene Citation[30]. Moreover, similar results were derived from in vitro and in vivo tumorigenesis assays. It is noteworthy that Ad-FAS-TK can exhibit anti-tumor effects on MCF-7 cells and MDA-MB-231 cells as well as SK-Br3 cells. This suggests Ad-FAS-TK has a wide target spectrum of breast cancer cells, so its gene therapy could be extensively used for breast cancer patients even with different histological types.
In summary, our results indicated that the recombinant adenoviral HSV-TK suicide gene driven by tumor-associated FAS promoter combined with GCV is a novel potential treatment for breast cancer gene therapy.
Acknowledgements
This work was supported by the China National Science Fund 30471944 (to Liu Wen-Chao).
References
- McPherson K, Steel CM, Dixon JM. ABC of breast diseases. Breast cancer-epidemiology, risk factors, and genetics. BMJ 2000; 321: 624–8
- Hart IR. Tissue specific promoters in targeting systemically delivered gene therapy. Semin Oncol 1996; 23: 154–8
- Matono S, Tanaka T, Sueyoshi S, Yamana H, Fujita H, Shirouzu K. Bystander effect in suicide gene therapy is directly proportional to the degree of gap junctional intercellular communication in esophageal cancer. Int J Oncol 2003; 23: 1309–15
- Kurihara T, Brough DE, Kovesdi I, Kufe DW. Selectivity of a replication-competent adenovirus for human breast carcinoma cells expressing the MUC1 antigen. J Clin Invest 2000; 106: 763–71
- Pandha HS, Martin LA, Rigg A, Hurst HC, Stamp GW, Sikora K, et al. Genetic prodrug activation therapy for breast cancer: A phase I clinical trial of erbB-2-directed suicide gene expression. J Clin Oncol 1999; 17: 2180–9
- Yu L, Yamamoto N, Kadomatsu K, Muramatsu S, Matsubara S, Sakiyama S, et al. Midkine promoter can mediate transcriptional activation of a fused suicide gene in a broader range of human breast cancer compared with c-erbB-2 promoter. Oncology 2004; 66: 143–9
- Li X, Zhang J, Gao H, Vieth E, Bae KH, Zhang YP, et al. Transcriptional targeting modalities in breast cancer gene therapy using adenovirus vectors controlled by α-lactalbumin promoter. Mol Cancer Ther 2005; 4: 1850–9
- Breidenbach M, Rein DT, Schondorf T, Khan KN, Herrmann I, Schmidt T et al. A new targeting approach for breast cancer gene therapy using the Heparanase promoter. Cancer Lett 2005;
- Semenkovich CF, Coleman T, Fiedorek FT, Jr. Human fatty acid synthase mRNA: Tissue distribution, genetic mapping, and kinetics of decay after glucose deprivation. J Lipid Res 1995; 36: 1507–21
- Alo PL, Visca P, Marci A, Mangoni A, Botti C, Di Tondo U. Expression of fatty acid synthase (FAS) as a predictor of recurrence in stage I breast carcinoma patients. Cancer 1996; 77: 474–82
- Milgraum LZ, Witers LA, Pasternack GR, Kuhajda FP. Enzymes of the fatty acid synthesis pathway are highly expressed in in situ breast carcinoma. Clin Cancer Res 1997; 3: 2115–20
- Gansler TS, Hardman W, Hunt DA, Schaffel S, Hennigar RA. Increased expression of fatty acid synthase (OA-519) in ovarian neoplasms predicts shorter survival. Hum Pathol 1997; 28: 686–92
- Swinnen JV, Roskams T, Joniau S, Van Poppel H, Oyen R, Baert L, et al. Overexpression of fatty acid synthase is an early and common event in the development of prostate cancer. Int J Cancer 2002; 98: 19–22
- Rashid A, Pizer ES, Moga M, Milgraum LZ, Zahurak M, Pasternack GR, et al. Elevated expression of fatty acid synthase and fatty acid synthetic activity in colorectal neoplasia. Am J Pathol 1997; 150: 201–8
- Yahagi N, Shimano H, Hasegawa K, Ohashi K, Matsuzaka T, Najima Y, et al. Co-ordinate activation of lipogenic enzymes in hepatocellular carcinoma. Eur J Cancer 2005; 41: 1316–22
- Piyathilake CJ, Frost AR, Manne U, Bell WC, Weiss H, Heimburger DC, et al. The expression of fatty acid synthase (FASE) is an early event in the development and progression of squamous cell carcinoma of the lung. Hum Pathol 2000; 31: 1068–73
- Innocenzi D, Alo PL, Balzani A, Sebastiani V, Silipo V, La Torre G, et al. Fatty acid synthase expression in melanoma. J Cutan Pathol 2003; 30: 23–8
- Menendez JA, Decker JP, Lupu R. In support of fatty acid synthase (FAS) as a metabolic oncogene: Extracellular acidosis acts in an epigenetic fashion activating FAS gene expression in cancer cells. J Cell Biochem 2005; 94: 1–4
- O'Connell MJ, McInerney JO. Adaptive evolution of the human fatty acid synthase gene: Support for the cancer selection and fat utilization hypotheses?. Gene 2005; 360: 151–9
- Menendez JA, Lupu R. Fatty acid synthase-catalyzed de novo fatty acid biosynthesis: From anabolic-energy-storage pathway in normal tissues to jack-of-all-trades in cancer cells. Arch Immunol Ther Exp (Warsz) 2004; 52: 414–26
- Alo PL, Visca P, Trombetta G, Mangoni A, Lenti L, Monaco S, et al. Fatty acid synthase (FAS) predictive strength in poorly differentiated early breast carcinomas. Tumori 1999; 85: 35–40
- Kuhajda FP, Pizer ES, Li JN, Mani NS, Frehywot GL, Townsend CA, et al. Synthesis and antitumor activity of an inhibitor of fatty acid synthase. Proc Natl Acad Sci USA. 2000; 97: 3450–4
- Jayakumar A, Tai MH, Huang WY, al-Feel W, Hsu M, Abu-Elheiga L, et al. Human fatty acid synthase: Properties and molecular cloning. Proc Natl Acad Sci USA. 1995; 92: 8695–9
- Hsu MH, Chirala SS, Wakil SJ. Human Fatty acid Synthase Gene: Evidence for the presence of two promoters and their functional interaction. J Biol Chem 1996; 271: 13584–92
- Menendez JA, Vellon L, Lupu R. Targeting fatty acid synthase-driven lipid rafts: A novel strategy to overcome trastuzumab resistance in breast cancer cells. Med Hypotheses 2005; 64: 997–1001
- Hynes NE, Stern DF. The biology of erbB-2/neu/HER-2 and its role in cancer. Biochem Biophys Acta 1994; 1198: 165–84
- Pandha HS, Martin LA, Rigg A, Hurst HC, Stamp GW, Sikora K, et al. Genetic prodrug activation therapy for breast cancer: A phase I clinical trial of erbB-2-directed suicide gene expression. J Clin Oncol 1999; 17: 2180–9
- Miyashiro I, Kaname T, Shin E, Wakasugi E, Monden T, Takatsuka Y, et al. Midkine expression in human breast cancers: Expression of truncated form. Breast Cancer Res Treat 1997; 43: 1–6
- Garver RI, Jr, Radford DM, Donis-Keller H, Wick MR, Milner PG, et al. Midkine and pleiotrophin expression in normal and malignant breast tissue. Cancer 1994; 74: 1584–90
- Nicholas TW, Read SB, Burrows FJ, Kruse CA. Suicide gene therapy with Herpes simplex virus thymidine kinase and ganciclovir is enhanced with connexins to improve gap junctions and bystander effects. Histol Histopathol 2003; 18: 495–507