Abstract
Introduction. In SRT/SRS, dedicated treatment planning systems are used for the calculation of the dose distribution. The majority of these systems utilize the standard TMR/OAR formalism for dose calculation as well as they usually neglect any perturbation due to head heterogeneities. The aim of this study is to examine the errors due to head heterogeneities for both absolute and relative dose distributions in stereotactic radiotherapy. Materials and methods. Dosimetric measurements in phantoms have been made for linac stereotactic irradiation. CT-based phantoms have been used for Monte Carlo simulations for both linac-based stereotactic system and Gamma Knife unit. Absolute and relative dose distributions have been compared between homogeneous and heterogeneous media. DVH and TCP results are presented for all cases. Results. The maximum absolute dose difference at the isocenter was 2.2% and 6.9% for the linac and Gamma Knife respectively. The impact of heterogeneity in the target DVH was minor for the linac technique whereas considerable difference was observed for the Gamma Knife treatment. This was reflected also to the radiobiological evaluation, where the maximum TCP difference for the linac system was 2.7% and for the Gamma Knife was 4%. Discussion and conclusions. The errors rising from the existence of head heterogeneities are not negligible especially for the Gamma Knife which uses lower energy beams. The errors of the absolute dose calculation could be easily eliminated by implementing a simple heterogeneity correction algorithm at the TPS. Nevertheless, the errors for not taking into account the lateral electron transport would require a more sophisticated approach and even direct Monte Carlo calculation.
Stereotactic Radiotherapy/Radiosurgery (SRT/SRS) is currently the treatment of choice for small inoperable brain tumors and Arterioveneous Malformations (AVMs) Citation[1], Citation[2]. There are two major SRT/SRS treatment modalities: the Leksell Gamma Knife® (LGK) and the Linear Accelerator (Linac) based systems. The Leksell Gamma Knife® uses 201 Cobalt-60 sources array distributed in a shape of a headband around the patient's head Citation[3], Citation[4]. There are four collimator helmets that can be used, providing collimation of 4, 8, 14, and 18 mm in diameter at focus distance. With Linac based SRT, usually tertiary cylindrical collimators are attached under the secondary jaws of the linac's head, having diameters that range from 5 mm to 40 mm when projected to the isocenter Citation[4–6]. The treatment beam arrangement usually consists of multiple non-coplanar convergent arcs that are used to deliver the desired dose distribution to the patient. Both systems present great target localization and treatment delivery accuracy, which is essential for focusing the high dose region to the target volume while minimizing the late-term complications and radiation induced necrosis Citation[2], Citation[4], Citation[5].
In SRT/SRS, dedicated treatment planning systems (SRT-TPS) are used for the calculation of the dose distribution. In the case of Gamma Knife®, the Gamma Plan® treatment planning system is used for dose calculation while in the case of the various linac-based SRT units different home-made or commercial SRT-TPSs are used Citation[4], Citation[5]. The vast majority of the these systems utilize the standard TMR/OAR formalism for dose calculation as well as they consider the patient's head of unit density and any perturbation due to head heterogeneities (such as skull bone and air cavities) is usually neglected Citation[4], Citation[7], Citation[8]. Taking into account that the SRT/SRS is basically a three-dimensional (3D) problem, the above approach exhibits several sources of errors. It is well-known that the conventional empirical and semi-empirical dose algorithms are approximate and limited in accuracy since they do not adequately take into account the lateral electron transport of radiation Citation[9], Citation[10]. As a result, they produce inaccurate dose distributions, particularly in the presence of inhomogeneities. The magnitude of the errors in the calculated doses increases as the field size decreases Citation[9], Citation[11]. Furthermore, the small stereotactic beams present lack of transient and lateral electronic equilibrium, which makes the acquisition of basic dosimetric data difficult especially since the volume of measuring devices (e.g. ion-chambers), is large comparing to the field size Citation[5], Citation[9], Citation[12]. This further reduces the accuracy of the conventional dose algorithms which use measured single beam data as input.
Errors rising from single static field irradiation might be large when the problems of electronic disequilibrium and tissue inhomogeneities are not taken into account. However, there is a general assumption that the multiple field arrangement is compensating the perturbation of the relative dose distribution due to heterogeneities. In conventional and conformal radiotherapy there are a number of clinical measurements and simulations supporting this assumption Citation[9], Citation[10], Citation[13]. Since stereotactic radiotherapy uses a large number of convergent beams, it is believed that the relative dose distribution of two similar plans, one taking into account the inhomogeneities of the head (air cavities and scull) and one considering the head as homogeneous medium, might be of negligible difference. However, as far as we know, there is no a complete clinical study supporting this argument.
The aim of this study is to examine the errors due to head heterogeneities for both absolute and relative dose distributions in stereotactic radiotherapy. For this purpose we have employed the most common used stereotactic irradiation techniques, Gamma Knife and linac-based systems, applied to phantoms and to different clinical cases. All calculations have been made with Monte Carlo, for homogeneous and heterogeneous media, in order to achieve better approximation to reality Citation[14]. Dose-volume histograms (DVHs) and tumor control probability (TCP) have been used as dosimetric and radiobiological methods respectively for the evaluation of the results.
Materials and methods
A. Monte Carlo model for the linac-based SRT unit
The BEAM code was used for the simulations of the linear accelerator Citation[15]. The version of the BEAM code used is the BEAMnrc, which uses the EGSnrc version of EGS Citation[16], Citation[17]. The Elekta SL 75-5 linear accelerator (a) was modeled in three steps. The component modules that defined the geometry of the Linac are: i) target, ii) primary collimator, iii) flattening filter, iv) monitor chamber and v) secondary (collimating) jaws. In the first step, parts i) – iv) were modeled and a resulting phase space was calculated before the secondary jaws. This step was implemented in order to save time for future simulations when the opening of the secondary jaws is altered in order to change the field dimensions. The resulting phase space from the first step was used as input in the second step where the simulation of the secondary jaws took place and then again a phase space file was created immediately after the jaws so it could be used further in the simulations. In this second step the 10 cm×10 cm field was originally modeled. The 10 cm×10 cm field was used to verify the model of the accelerator against measured data in a water phantom. Depth dose data provide benchmark of the nominal energy of the accelerator while off axis ratios provided the benchmarks for the full width at half maximum (FWHM) of the Gaussian distribution of the primary lateral electron fluence incident on the target. In order to verify the accelerator's model, nominal energies ranging from 5.6 MeV to 6.4 MeV were simulated and the nominal energy of 6.1 MeV was chosen when compared to the dose measurements.
Figure 1. (a) The linear accelerator's outline used for Monte Carlo simulation. The position of each scoring plane used is shown. The 3rd phase space is used for dose calculation both in phantoms and in the real patient cases. (b) The Gamma Knife outline used for Monte Carlo simulation. The position of each scoring plane used is shown. The 2nd phase space is used for dose calculation both in phantoms and in the real patient cases.
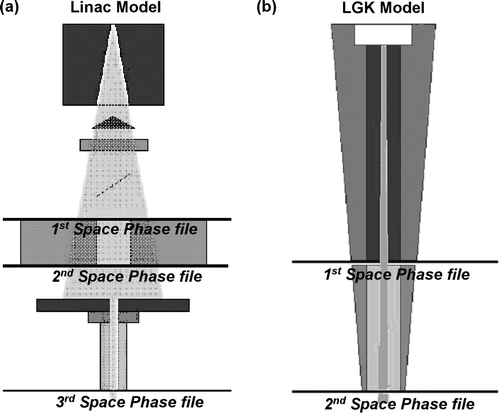
In the third step the stereotactic collimators were modeled along with the assembly that attaches them on the linear accelerator head (a). The phase space file of 8 cm×8 cm field was used as input in this simulation and the resulting phase space file after the stereotactic collimators was stored for the dose computations.
The resulting phase space file had approximately 3×107 particles. The variance reduction techniques that were used are: Brehmsstralung splitting of 20 and Russian roulette ON and also the ECUT and PCUT were set to 1.0 MeV and 0.01 MeV respectively inside the component modules of the primary collimator, secondary collimator and inside the stereotactic collimator.
B. Monte Carlo model for the Gamma Knife unit
The Gamma Knife® was modeled in a similar manner with the linac (b). The modeling in this case consisted of two steps. The first step was the simulation of the source body and the central body with the stationary collimator, and in the second step the helmet and the final collimator was simulated. The phase space created in the first step was used as input for the second case in order to reduce the time of the simulation of all the collimators. A second phase space was scored for each collimator after the final collimator.
The resulting phase space file had approximately 3×107 particles. Variance reduction techniques used were Brehmsstralung splitting of 20 and Russian roulette ON and also the ECUT and PCUT were set to 1.0 MeV and 0.01 MeV respectively inside the component modules of the central body and helmet. The whole simulation has been verified against benchmark dosimetric data provided by ELEKTA.
C. Phantoms
Two phantoms, with dimensions 10×10×10 cm3, have been used for the assessment of the dose perturbation due to high density materials in the case of the linac-based stereotactic irradiation. The one phantom was homogeneous, consisted only of PMMA slabs, and at the other one slab of 5 mm Al was introduced at the end of buildup region i.e. 1.5 cm depth, simulating bone (). Both phantoms had the ability to accommodate an ion-chamber (PTW, type M2332, with effective volume of 0.1 cc) for on-axis measurements and film (Kodak, EDR) for off-axis measurements. The on-axis measurements with ion-chamber where started 1 cm bellow interface and the standard deviation at each measurement (3 measurements per point) was less than 0.5%. The off-axis measurements with film dosimetry have been done at one depth (1 cm bellow interface), the films were then digitized (VIDAR 3600) with analysis of 0.2 mm and the standard deviation was ±1.5%. The two phantoms have been also digitized in order to be used by the DOSXYZ code for Monte Carlo simulation of the dose distribution. The corresponding Monte Carlo calculated depth dose curves and profiles have been produced using 1×1×1mm3 voxel size with statistical uncertainty of 1% for all cases.
Figure 2. Linac-based stereotactic irradiation in phantoms: (a) Depth dose curves measured and calculated in homogeneous (PMMA) and heterogeneous (PMMA-Al) phantoms. The inhomogeneity thickness was 5 mm and it was placed 1.5 cm below surface. The measurements were done with ion-chamber (first position 1 cm below inhomogeneity, standard deviation <0.7%) and calculations were done with Monte Carlo. (b) Off-Axis Ratios curves measured in homogeneous (PMMA) and heterogeneous (PMMA-Al) phantoms. The inhomogeneity thickness was 5 mm and it was placed 1.5 cm below surface. The measurements were done with radiographic films (standard deviation <0.5%) placed 1 cm below inhomogeneity.
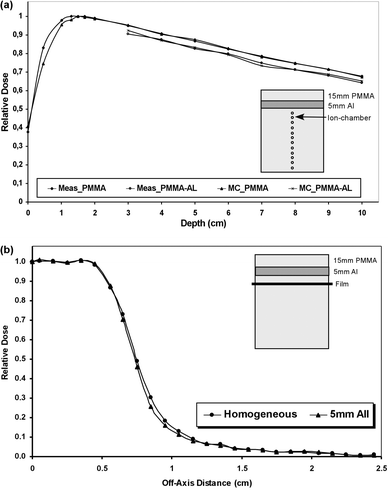
For the LGK irradiation only digital phantoms were used since there was no possibility for measurements. The one phantom was homogeneous (water) and at the other, heterogeneity of 1cm bone was introduced just bellow the buildup region i.e. 0.5 cm depth (). The two phantoms of dimensions 10×10×10 cm3, with analysis of 1×1×1mm3 voxel size, where introduced to DOSXYZ code. Only one source has been used at the Monte Carlo simulations and the statistical uncertainty was less than 1%.
Figure 3. LGK irradiation in phantoms: (a) Monte Carlo calculated depth dose curves for homogeneous (water) and heterogeneous (water-bone) phantoms. The inhomogeneity thickness was 1 cm and it was placed 0.5 cm below surface. (b) Monte Carlo calculated off-axis ratios curves for the same phantoms. The statistical uncertainty for both simulations was <1%.
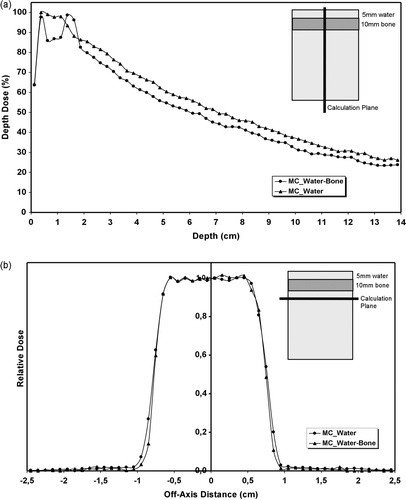
One complete CT data set was used as patient phantom. The CTCREATE code was used in order to convert the DICOM images to a format that could be used by DOSXYZ when possible Citation[18]. In the cases where CTCREATE failed to complete the task the DICOM-RT Toolbox was used Citation[19]. For both linac-based and LGK systems, five different spherical clinical target volumes (CTVs) were defined in different positions inside the head. The isocenter positions were chosen in order to cover the majority of clinical cases accounted in stereotactic radiation. Thus, one was located centrally in the brain, one at the top of the head, one close to the skull bone (0.5 cm from the bone), one approximately 3 cm from the skull bone and one close to an air cavity ().
For all phantom simulations, the parameters for DOSXYZnrc were: AE = ECUT = 0.700 MeV, AP = PCUT = 0.010 MeV, photon splitting number was 1 and there was no recycling of the particles. The range rejection technique was turned off. 3×108 histories were simulated in DOSXYZnrc in order to get a statistical uncertainty below 1%.
D. Irradiation scheme and dose calculation
For the linac-based stereotactic treatment, each case was irradiated with five arcs at table positions 30, 60, 90, 300, and 330 degrees and arc length of 100°. The collimator diameter for all cases was 20 mm at isocenter distance. The dose prescription consisted of a reference dose of 25 Gy at the 75% reference isodose.
For the LGK irradiation, all 201 sources were used and the collimator diameter was 18 mm at focus distance for all cases. The dose prescription consisted of a reference dose of 24 Gy at the 75% reference isodose.
The DOSXYZnrc code, part of the OMEGA project, was used for the simulation of the transport of energy in the phantoms Citation[18]. The phase space files created under the stereotactic collimator (for the linac) or under the helmet (for the LGK) were used as input. All the DOSXYZnrc and BEAMnrc simulations were performed using a Linux cluster of four Pentium IV 1.8 GHz PCs. For the DOSXYZnrc simulations the number of histories run was 3×108 taking about 4–5 hours for each plan. For the BEAMnrc simulations the time required to produce a phase space files after the collimating jaws (Linac) was approximately 15 hours and for the phase space files after each collimator about 8 to 10 hours. The time required for the LGK simulations was of the same order. For all simulations, the statistical uncertainty was less than 1%.
E. Radiobiological evaluation
The radiobiological statistical model used in this work in order to evaluate the different dose distributions, was the linear Poisson model which mathematically is given by the expression:1 where P(D) is the probability of obtaining tumor control assuming that the whole CTV is irradiated uniformly with a dose D. D50 is the dose which gives a response probability of 50% and γ is the maximum normalized value of the dose-response gradient. The parameters D50 and γ characterize the shape of the target dose-response relation. The suitability of the linear Poisson model for describing stereotactic irradiation has been discussed in the literature Citation[20–22].
For a heterogeneous dose distribution, which is most likely the case for stereotactic radiotherapy, tumor control probability (TCP) using the linear Poisson model is given by the expression:2 where Δvi (=ΔVi/Vref) is the fractional irradiated subvolume of the target compared to the reference volume for which the values of D50 and γ have been calculated. TCP(Di) is the probability of target response when the target has the reference volume and is irradiated to dose Di as described by equation (1) and M is the total number of voxels or subvolumes in the target receiving a certain dose. The reference volume is related to the characteristics of the clinical material from which the parameters D50 and γ were calculated. In this study, the radiobiological parameters used were D50=22.9 Gy, γ=1.25 and Vref=3.0cm3, which are relevant to stereotactic single fraction brain irradiation Citation[21]. The radiation sensitivity was assumed to be homogeneous throughout the target volume of each case.
Finally, since different dose distributions were compared, the biologically effective uniform dose, , was used also as evaluation index Citation[23], Citation[24].
is the uniform dose that causes exactly the same obliteration probability as the real dose distribution delivered to the individual patient and is given by the expression:
3
Results
For the 6 MV single stereotactic beam incident on the phantom, the perturbation of the on-axis and off-axis dose distribution due to Al heterogeneity is shown in . Monte Carlo calculations and measurements show a very good agreement (less than 1%). The dose at central axis beyond the heterogeneity is reduced, compared to the dose at homogeneous medium, and remains reduced at all depths (a). The mean difference is 3.6% (1 s.d. 0.4%). The dose reduction is attributed to higher attenuation of the photon in the presence of the Al slab. High density heterogeneity has little effect on transverse dose distribution. The dose profile appears to have a slightly reduced penumbra width (distance between 20–80% dose points) when heterogeneity is involved while the field width is reduced by 0.9mm (b).
The results of the LGK single beam incident on the phantom are presented in . The central axis dose is reduced inside the bone slab compared with the homogeneous dose values, then there is a clear buildup region exactly after the heterogeneity due to increased scattered electrons from the high density material and finally the dose is reduced, compared with the dose at homogeneous medium, due to increased attenuation, and remains reduced at all depths (a). The mean dose difference at the exponential region between homogeneous and heterogeneous media is 4.8% (1 s.d. 0.6%). Concerning the transverse dose distribution, the presence of the high density heterogeneity has almost no influence on the beam width but a large decrease of the penumbra width is observed (3.5 mm difference) compared with dose at homogeneous media (b). This effect is attributed to beam hardening, since the low energy component was absorbed by the bone slab, so the remaining photons are more energetic and thus more forward scattered.
The results of the irradiation of the different patient phantoms for the linac-based stereotactic technique are presented in . The absolute dose at isocenter (Disoc), the mean dose (Dmean) at the CTV, the minimum dose (Dmin) at the CTV, the TCP and the , for every case are shown as well as their percentage difference between homogeneous and heterogeneous medium. The maximum difference of the Disoc is 2.2% when the isocenter is located at the top of the head while the maximum difference of the Dmean is 2.0% when the isocenter is located at the middle of the head. The Dmin presents a maximum difference of 2.8% when the isocenter is located 0.5 cm from the scull bone. The maximum difference of the TCP is 2.7% and of
is 1.9% when the isocenter is located at the middle of the head. The relative 3D dose distributions in the form of DVHs, for two of five cases, are shown in . As expected, the differences between homogeneous and heterogeneous dose distributions are quite small for both CTV and normal tissues.
Figure 5. Dose Volume Histograms (DVHs) for the linac-based stereotactic irradiation for the clinical target volume (CTV) and the normal tissues. As normal tissue volume was considered the whole brain. The upper diagram is the DVH produced when the isocenter is located at the middle of the head while the lower diagram is the DVH produced when the isocenter is located 3 cm from the scull bone.
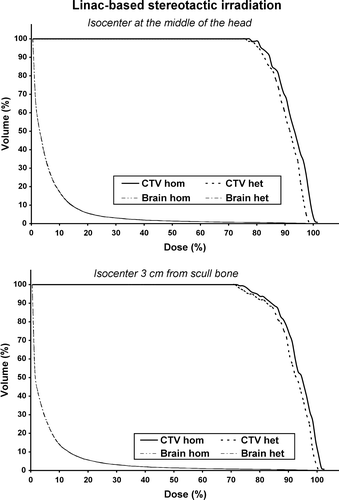
Table I. Linac-based stereotactic irradiation.
At the same parameters are presented for every case concerning the LGK irradiation of the different patient phantoms. As the photon energy is lower comparing to the linac system, the differences between homogeneous and heterogeneous medium, for both relative and absolute dose distributions, are larger. At the relative 3D dose distributions, for the two of five cases, are shown in the form of DVHs. Concerning the CTV, there is an obvious shrinkage of the higher isodoses resulting to an underdosage. The presence of heterogeneity does not have any important effect on the DVH of the normal tissues. Concerning the absolute dose values, the maximum difference of the Disoc is 6.9% when the isocenter is located 0.5 cm from the scull bone while the maximum difference of the Dmean is 5.1% when the isocenter is located at top of the head. The Dmin presents a maximum difference of 5.1% when the isocenter is located the top of the head. The maximum difference of the TCP is 4.0% and of is 5.0% when the isocenter is located at the top of the head.
Figure 6. Dose Volume Histograms (DVHs) for the Gamma Knife stereotactic irradiation for the clinical target volume (CTV) and the normal tissues. As normal tissue volume was considered the whole brain. The upper diagram is the DVH produced when the isocenter is located at the middle of the head while the lower diagram is the DVH produced when the isocenter is located 3 cm from the scull bone.
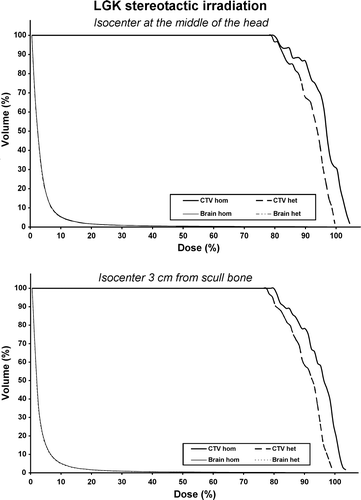
Table II. Gamma Knife stereotactic irradiation.
Discussion
Errors rising from the presence of head heterogeneities for both absolute and relative dose distributions in stereotactic treatment were studied in this work. The most common stereotactic irradiation techniques i.e. Gamma Knife and linac-based systems, were employed for that purpose and stereotactic irradiation was applied to phantoms and to different clinical cases. The 3D dose distributions, produced by Monte Carlo simulations for homogeneous and heterogeneous media, have been compared by physical, DVHs, as well as by radiobiological, TCP and means.
Even though there are several studies on the impact of high or low density heterogeneities for stereotactic beams of energies 6–10 MV, there are no studies, as far as we know, addressing this subject for Co-60 stereotactic beams such the ones employed for Gamma Knife treatments. This is mainly due to the fact that the Gamma Knife users most frequently utilize MRI and angiographic images so no complete density information is used by the GammaPlan.
Our central axis dose measurements and Monte Carlo simulations, with a single linac stereotactic beam, indicate that ignoring the high density heterogeneity such as scull bone is resulting to an overestimation of the dose at isocenter of the order of 3%. Those results agree with the work of Rustgi et al. where the estimated error was 2–5% depending on heterogeneity thickness and field size Citation[11]. It has been proven Citation[9] that as the photon energy decreases the dose perturbation due to high density material in increased due to increased absorption of the incident beam. This is also shown to the Gamma Knife results on central axis dose calculations where the error is of the order of 5%.
The off-axis measurements and Monte Carlo calculations show a considerable shrinkage of the penumbra region. For the linac-based irradiation, the penumbra at the inhomogeneous phantom was reduced by approximately 1 mm compared to the homogeneous medium and this result agrees with previous published studies Citation[11]. The penumbra decrease for the Co-60 beam in the inhomogeneous medium was of the order of 3.5 mm.
The absolute dose overestimation when inhomogeneities are not taken into account is 1–3% and 1–6% for the linac-based stereotactic irradiation and for the Gamma Knife irradiation respectively. As the isocenter moves in depth, the differences in absolute dose are decreasing since we are moving away from the heterogeneity and its influence is decreasing. Ayyangar et al. predicted an overestimation of 2.6% of the absolute isocenter dose for the 6 MV when the dose calculation is done with conventional algorithm without heterogeneity correction Citation[7].
The dose perturbation on the single stereotactic beam due to high density heterogeneity has an important impact on the 3D dose distribution when multiple convergent beams are employed. For the 6 MV photon beam there is shrinkage of the higher isodose levels () resulting to a small underdosage of the target. This is reflected also to the TCP and values which range between 1% and 3%. At the work of Ayyangar et al. for the clinical cases, this effect was not observed resulting to the conclusion that the relative dose distribution is not changing. This is not the case for our results since the differences in the DVHs are systematic for all cases. The shrinkage of the higher isodose values is more pronounced at the Gamma Knife irradiation () resulting in a larger target volume underdosage. The corresponding differences in TCP and
range between 1% and 4%.
The observed differences in absolute and relative dose distributions when the isocenter is located close to an air cavity are negligible. One would expect that the presence of low density heterogeneity in the proximity of the target volume would result to an underdosage of the CTV due to lack of scatter electrons and an overdosage of the surrounding normal tissues due to enhanced electron and photon range through air. Especially for the Gamma Knife, there are studies showing that the CTV underdosage due to air cavities is between 7 and 10% Citation[25], Citation[26]. Those effects are not observed at our results. This probably due to small size of the air cavity in our clinical cases as well as due to low energy beams used. Nevertheless, this point needs further investigation especially if stereotactic irradiation is to be used for tumors lying at the base of the scull or at head and neck region.
Finally, one should take into account that all dose calculations for both homogeneous and heterogeneous media have been done with Monte Carlo simulation which is more accurate than any of the conventional and sophisticated algorithms used in treatment planning systems today. Thus, the errors due to heterogeneities in head stereotaxy are expected to be larger in the every day clinical practice when a conventional treatment planning is used. Taking into account that in stereotactic radiotherapy more accuracy is required than in conformal radiotherapy due to the fact that most frequently is a single fraction treatment, the observed dosimetric difference can be considered important. According to the results of this study it is worth the trouble to incorporate heterogeneity correction algorithms in stereotactic TPSs as well as to change the usual clinical practice of the Gamma Knife treatment in order to include CT image data.
Conclusion
The errors rising from the existence of head heterogeneities are not negligible especially for the Gamma Knife which uses lower energy beams. The errors of the absolute dose calculation could be easily eliminated by implementing a simple heterogeneity correction algorithm at the TPS. Nevertheless, the errors for not taking into account the lateral electron transport would require a more sophisticated approach and even direct Monte Carlo calculation.
References
- Auchter RM, Lamond JP, Alexander E, Buatti JM, Chappell R, Friedman WA, et al. A multiinstitutional outcome and prognostic factor analysis of radiosurgery for resectable single brain metastasis. Int J Radiat Oncol Biol Phys 1996; 35: 27–35
- Delannes M, Daly NJ, Bonnet J, Sabatier J, Tremoulet M. Fractionated radiotherapy of small inoperable lesions of the brain using a non-invasive stereotactic frame. Int J Radiat Oncol Biol Phys 1991; 21: 749–55
- Leksell L. Stereotaxic and radiosurgery. An operative system. Springfield, Illinois: Thomas; 1971.
- Webb S. The physics of three-dimensional radiation therapy. Medical Science Series, Institute of Physics, London 1993
- AAPM report No. 54. Stereotactic Radiotherapy. 1995.
- Kooy HM, Nedzi LA, Loeffler JS, Alexander E 3rd, Cheng CW, Mannarino EG, et al. Treatment planning for stereotactic radiosurgery of intra-cranial lesions. Int J Radiat Oncol Biol Phys 1991; 21: 683–93
- Ayyangar KM, Jiang SB. Do we need Monte Carlo treatment planning for linac based radiosurgery? A case study. Med Dosim 1998; 23: 161–8
- Maitz AH, Wu A. Treatment planning of stereotactic convergent gamma-ray irradiation using Co-60 sources. Med Dosim 1998; 23: 169–75
- AAPM Report 85. Tissue inhomogeneity corrections for megavoltage photon beams. Radiation Therapy Committee Task Group No. 65. 2004.
- Ahnesjo A, Aspradakis MM. Dose calculations for external photon beams in radiotherapy. Phys Med Biol 1999; 44: R99–R155
- Rustgi SN, Rustgi AK, Jiang SB, Ayyangar KM. Dose perturbation caused by high-density inhomogeneities in small beams in stereotactic radiosurgery. Phys Med Biol 1998; 43: 3509–18
- Verhaegen F, Das IJ, Palmans H. Monte Carlo dosimetry study of a 6 MV stereotactic radiosurgery unit. Phys Med Biol 1998; 43: 2755–68
- Engelsman M, Damen EM, Koken PW, van't Veld AA, van Ingen KM, Mijnheer BJ. Impact of simple tissue inhomogeneity correction algorithms on conformal radiotherapy of lung tumours. Radiother Oncol 2001; 60: 299–309
- Leavitt DD, Starkschall, G et al, Proceedings of the 12th International Conference on the use of Computers in Radiation Therapy. Medical Physics Publishing; 1997. p 16–18.
- Rogers DW, Faddegon BA, Ding GX, Ma CM, We J, Mackie TR. BEAM: A Monte Carlo code to simulate radiotherapy treatment units. Med Phys 1995; 22: 503–24
- Karawkow IW, Rogers DWO. The EGSnrc code system: Monte Carlo Simulation of Electron and Photon Transport. NRCC Report PIRS-701. 2002.
- Rogers DWO, Karawkow IW, Seuntjens JP, Walters BRB. NRC User Codes for EGSnrc”, NRCC Report PIRS-702. 2002.
- Ma C, Rogers DWO, Walters B. DOSXYZnrc users Manual. NRC Report PIRS-509b(revF). 2001.
- Spezi E, Lewis DG, Smith CW. A DICOM-RT-based toolbox for the evaluation and verification of radiotherapy plans. Phys Med Biol 2002; 47: 4223–32
- Karlsson B, Lax I, Soderman M. Can the probability for obliteration after radiosurgery of arteriovenous malformations be accurately predicted?. Int J Radiat Oncol Biol Phys 1999; 43: 313–9
- Mavroidis P, Theodorou K, Lefkopoulos D, Nataf F, Schlienger M, Karlsson B, et al. Prediction of AVM obliteration after stereotactic radiotherapy using radiobiological modelling. Phys Med Biol 2002; 47: 2471–94
- Mavroidis P, Lind BK, Theodorou K, Laurell G, Fernberg J-O, Lefkopoulos D, et al. “Statistical methods for clinical verification of dose-response parameters related to esophageal stricture and AVM obliteration from radiotherapy”. Phys Med Biol 2004; 49: 3797–816
- Mavroidis P, Lind BK, Brahme A. Biologically effective uniform dose () for specification, report and comparison of dose response relations and treatment plans. Phys Med Biol 2001; 46: 2607–30
- Mavroidis P, Lind BK, Brahme A. , an effective uniform dose linked to the probability of response. Phys Med Biol 2002; 47: L5–9
- Moskovin V, Timmerman R, DesRosiers C, Randall M, DesRosiers P, Dittmer P, et al. Monte Carlo silumation of the Leksell Gamma Knife®: II. Effects of heterogeneous versous homogeneous media for stereotactic radiosurgery. Phys Med Biol 2004; 49: 3879–95
- MO Al-Dweri F, Rojas EL Lallena MA. Effects of bone- and air-tissue inhomogeneities on the dose distributions of the Leksell Gamma Knife calculated with PENELOPE. Phys Med Biol 2005;50:5665–78.