Abstract
Background. We have implemented an intensity-modulated radiotherapy (IMRT) protocol for simultaneous irradiation of bladder and lymph nodes. In this report, doses to normal tissue from IMRT and our previous conformal sequential boost technique are compared. Material and methods. Sixteen patients with urinary bladder cancer were treated using a six-field dynamic IMRT beam arrangement delivering 60 Gy to the bladder and 48 Gy to the pelvic lymph nodes. Dose-volume histogram (DVH) parameters for relevant normal tissues (bowel, bowel cavity, rectum and femoral heads) for the IMRT plans were compared with corresponding DVHs from our previous conformal sequential boost technique. Calculations of the generalized Equivalent Uniform Dose (gEUD) were performed for the bowel, with a reference volume of 200 cm3 and a volume effect parameter k = 4, as well as for the rectum, using k = 12. Acute gastrointestinal (GI) and genitourinary (GU) RTOG toxicity was recorded. Results. Statistical significant normal tissue sparing was obtained by IMRT. For the bowel, a significant reduction was obtained at all dose levels between 20 and 50 Gy (p < 0.05), e.g. from 180 to 121 cm3 at 50 Gy, while the gEUD was reduced from 58 to 53 Gy (p < 0.05). Similar patterns were seen for the bowel cavity. For the rectum, IMRT reduced the maximum dose as well as the volumes receiving more than 50 and 60 Gy (p < 0.05), e.g. from 72 to 48 cm3 at 50 Gy. The rectum gEUD was reduced from 55 to 53 Gy (p < 0.05). For the femoral heads, IMRT reduced the maximum dose as well as the volumes above all dose levels. The rate of acute peak Grade 2 GI RTOG complications was 38% after IMRT. Conclusion. IMRT to the urinary bladder and elective lymph nodes result in considerable normal tissue sparing compared to conformal sequential boost technique. This has paved the way for further studies combining IMRT with image-guided radiotherapy (IGRT) in bladder cancer.
The main challenge in radiotherapy (RT) is to deliver a tailored high radiation dose to the tumour while minimizing irradiation to surrounding healthy tissue. Recent technological development has considerably improved our possibilities to individualize RT dose distributions to match the 3-D tumour shape Citation[1]. A major technological solution within this field, the intensity-modulated RT (IMRT), applies beams with varying intensity across the fields. IMRT has shown great potential to spare normal tissue and to ensure tumour coverage and thus enable tumour dose escalation, also in bladder cancer Citation[2–5]
Treatment outcome using traditional conformal RT of bladder cancer is modest Citation[6]. In a study from Aarhus University Hospital, the 5 year overall survival and local control was 21 and 37%, respectively. Furthermore, considerable acute and long term side-effects were observed Citation[7], Citation[8]. Efforts to improve treatment plans have therefore been considered, with special focus on normal tissue sparing, in particular for the bowel and rectum. Because of considerable internal movement of the bladder, large safety margins around the clinical target volume are required. Consequently, this results in large volumes of normal tissues included in the high dose region. Detailed studies on bladder filling and interfractional movement of the bladder have enabled us to implement an anisotropic margin Citation[9], Citation[10]. To safely reduce the margins, individualized margins based on image-guided strategies have been explored Citation[11].
As a starting point for our image-guided IMRT activities, we have implemented an IMRT protocol for simultaneous treatment of the bladder and the lymph nodes. The present study evaluates the normal tissue sparing effect of IMRT in comparison to a conventional sequential boost 3-field box technique which was previously the standard for RT of bladder cancer in our department.
Materials and methods
Patient material
Between June 2007 and February 2008, 16 consecutive patients were treated with IMRT for bladder cancer at Department of Oncology, Aarhus University Hospital. Diagnostic workup, staging and examination for metastatic disease including CT of the abdomen and CT or conventional x-ray of the thorax were performed in all patients. The patients had T2-T4a N0M0 disease and were considered inoperable due to severe co-morbidity. The mean age was 70 years (range 51-79) and the majority of patients were men. Patient and tumour characteristics are shown in .
Table I. Patient characteristics
Dose planning CT and definitions of targets and organs at risk
Patients were immobilized in supine position using a heel and knee fixation system. The CT scans were performed using a spiral scanner (Siemens Somatom Plus 4) with 3 mm slice thickness (6 mm/s) and reconstructed with 512×512 pixels and 3 mm inter-slice distance. Before CT planning and treatment the patient was instructed to empty the bladder. Delineation was performed in collaboration between a radiation oncologist and a diagnostic radiologist by use of the Eclipse treatment planning system (Varian Oncology Systems, Palo Alto, CA, USA). Two planning target volumes (PTV) were contoured: PTV1 included the bladder with margins and PTV2 included both the bladder and lymph nodes with margins. The bladder included bladderwall as well as its content. The lymph nodes were contoured from the level of the promontory, with the internal iliac included to the obturatorial fossa and the external iliac to the level of the femoral heads. Para-rectal and pre-sacral lymph nodes below the sacro-iliac joint were excluded. An-isotropic wide margins were used around the bladder to account for the considerable geometrical uncertainties present in bladder cancer (superior 25 mm, inferior 15 mm, lateral 20 mm, anterior 25mm and posterior 20mm) whereas a uniform 10 mm margin was added between the lymph nodes and the PTV2 to account for delineation and geometrical uncertainities. Electronic portal images were acquired at setup of the first treatment, and repeated whenever required according to a standard protocol. Daily image-guidance was not performed.
Organs at Risk (ORs) (bowel, bowel cavity, rectum and femoral heads) were contoured. The bowel volume included all segments of contrast and non-contrast enhanced small intestine and colon as seen on the planning scan (superior border 12 mm above the PTV2). For the rectum, the recto-sigmoid flexure was applied as the superior/cranial limit and the anal verge as the inferior/caudal limit. Both the bowel and rectum were outlined as wall with contents. The femoral heads were outlined from the most cranial part to the inferior part of minor trochanter on both sides.
Field arrangements and optimization criteria
An IMRT plan with simultaneous integrated boost delivering 48 Gy to PTV2 and 60 Gy to PTV1 in 30 fractions (2 Gy daily fractions, 5 days a week) was used for all patients. A six-field beam arrangement with standard gantry angles of 45°, 82°, 168°, 192°, 278°, 315° was applied for all patients using 15 MV photon beam. A collimator angle of 5° was used for all beam to reduce tongue-and-groove effects. Patients were treated on Varian Clinacs equipped with the Millennium MLC-120 multi leaf collimator (MLC). Intensity-modulation was obtained using the sliding window technique.
Treatment planning and optimization were performed in ARIA (Varian Oncology Systems, Palo Alto, CA, USA) using locally defined clinical protocols including structure templates, field arrangement, prescription and optimization criteria. Four virtual volumes were defined for optimization purposes: oPTV60, oPTV48, oBowel and oRectum. The oPTV60 was a copy of PTV1 reduced with 5 mm from the skin surface. The oPTV48 was a copy of PTV2 reduced with 5 mm from the skin surface and oPTV60 subtracted so that only one dose level was prescribed to the volume. The oBowel was defined as the content of the bowel more than 5 mm from PTV1 and PTV2 in the cranio-caudal extension of the PTV1 and PTV2. The oRectum was the part of rectum with at distance of more than 8 mm from PTV2. PTV constraints were hard whereas the constraints on the rectum, oRectum and oBowel were soft.
The mean dose to PTV1 was normalised to 60 Gy. The optimisation strategy was to increase the priorities to oPTV60 and oPTV48 until at least 99% of PTV1 were covered by 95% of 60 Gy and at least 99% of PTV2 were covered by 95% of 48 Gy while keeping the global maximum less than 107% of 60 Gy. Next, the soft constraints to oBowel and oRectum were modified until the 50 Gy isodose line never circumscribed the whole circumference of the rectum at any CT-slice and V35 Gy < 20% for the bowel without violating the target coverage. A smoothing factor of 60 was used for all fields.
For the purpose of this study, patients were re-planned with the traditional sequential boost 3-field box technique using two opposing lateral fields and one anterior field (gantry angles 90°, 270°, 0°) using 15 MV photon beams. Fields were individually shaped with the MLCs. Margins and constraints to targets and ORs were the same as for the original treatment plan. The prescribed dose to the PTV2 was 46 Gy in 23 fractions followed by a sequential boost to the PTV1 of 14 Gy in 7 fractions (2 Gy daily fractions, 5 days a week).
Analysis of dose-volume histograms
DVH parameters (in 10 Gy steps) were derived for bowel, bowel cavity, rectum and femoral heads as well as median dose, dose to specified percent volume of PTVs (e.g. near minimum D98%, near maximum D2%) and standard deviation of the dose to the PTVs. Since PTV2 encompasse PTV1 in the IMRT treatment plan, we subtracted the PTV1 volume in the analysis of PTV2 related parameters. IMRT DVH parameters were compared with corresponding DVH parameters resulting from the summed plans of our previous conformal sequential boost technique.
Calculations of the bowel generalized Equivalent Uniform Dose (gEUD) were performed to supplement the comparisons of the DVHs for the IMRT and CRT technique. Bowel gEUDs were also calculated for CRT plans with a prescribed dose to the PTV1 of 60 Gy and 64 Gy, respectively. For the bowel, we followed the method by Hysing et al., extending the Niemierko EUD equation by introducing an absolute reference volume, Vref, and by replacing the volume fraction with the absolute volume at the ith differential DVH dose bin Citation[12], Citation[13].
A volume dependency parameter k = 4 and a Vref = 200 cm3 was used for the bowel Citation[14]. For the rectum, the standard gEUD equation was used (with fractional volumes) and with k = 12 Citation[15]. The choice of parameters (k-value, Vref) for the different ORs in the extended Niemierko formula (gEUD) was derived from available literature to make comparison with similar studies feasible.
To describe the homogeneity of the dose distribution of the plans, we calculated the Homogeneity Index (HI) Citation[16].
Finally, to compare the conformity of the various plan alternatives, the Dice Similarity Coefficient (DSC) was calculated Citation[17]. Treated volume (TV) was the volume enclosed within the 95% isodose level.
Scoring of toxicity
Acute toxicity was evaluated retrospectively from patient charts using the Radiation Therapy Oncology Group (RTOG) scoring system Citation[18], to grade peak lower gastrointestinal (GI) and genitourinary (GU) morbidity before, during and at the last day of treatment. Patients were scheduled for regular assessments of toxicity during the course of therapy. Anal symptoms were scored using the modified scoring system of Koper et al. Citation[19] together with the GI score. First cystoscopy follow-up was scheduled 3 months after RT.
Results
Normal tissue DVHs
Using IMRT we obtained considerable normal tissue sparing (). For the bowel, a statistical significant reduction was obtained at all dose levels between 20 and 50 Gy (p < 0.05), e.g. from 180 to 121 cm3 at 50 Gy. Similar patterns were observed for the bowel cavity, e.g. a reduction from 465 cm3 to 319 cm3 at 50 Gy (p < 0.05). For the rectum, IMRT reduced the maximum dose as well as the volumes receiving more than 50 and 60 Gy (p < 0.05), e.g. from 72 to 48 cm3 at 50 Gy. For the femoral heads, IMRT reduced the maximum dose as well as the volumes above all dose levels. illustrates an iso-dose distribution comparing CRT and IMRT in one patient. This demonstrates the ability of IMRT to deliver a high dose to the PTV1 and PTV2 while sparing the surrounding healthy tissue, in particular the bowel.
Figure 1. Example of a treatment plan for RT of bladder cancer including the lymph nodes with IMRT (left) and the summed sequential CRT (right) in the upper (upper) and lower (lower) pelvis. Target volumes shown in red, intestine in pink, bladder in yellow and rectum in blue. Dose colour wash is from 25 Gy (dark blue) to 50 Gy (red).
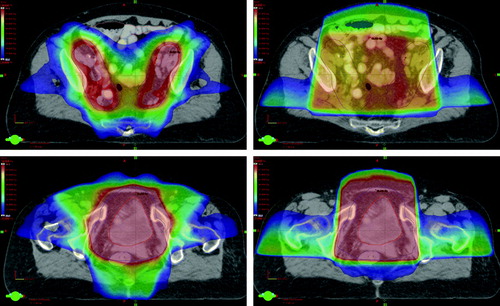
Table II. Mean volume of organs (ORs) at risk receiving step-vice increased radiation doses.
Normal tissue EUDs
Use of IMRT instead of the sequential boost CRT technique reduced the average bowel gEUD from 58 to 53 Gy (p < 0.05). Calculation on the CRT plan treating the bladder (PTV1) alone resulted in an average bowel gEUD of 52 and 55 Gy for a prescription dose of 60 and 64 Gy respectively. For the rectum, the average gEUD was reduced from 55 to 53 Gy using IMRT instead of CRT (p < 0.05).
We found no correlation between the reductions in bowel gEUD and individual planning parameters such as bladder volume, the volume overlap between bowel and PTVs or the ratio between high- and low-dose PTV.
Target coverage
IMRT and CRT were similar with respect to the average dose homogeneity, standard deviation of the dose, median, D2% and D98% doses for the PTV1. However, IMRT showed improved DSC compared to CRT (from 0.62 to 0.88, p < 0.05). In PTV2, the prescription dose differed (e.g. IMRT 48 Gy, CRT 46 Gy), and still the use of IMRT reduced the average median dose from 52.5 to 49.6 Gy (p < 0.05). The average homogeneity index was improved from 29% to 22% (p < 0.05), the average D2% from 60.8 to 57.5 Gy (p < 0.05) and the average standard deviation of the dose decreased from 4.6 to 2.8 Gy (p < 0.05). No difference in D98% was observed for PTV2 between IMRT and CRT treatment plans.
Acute effects
Acute Grade 2 GI effects were reported by 6 of 16 patients (38%), while Grade 1 was the highest GI adverse effect in 6 patients (38%). No Grade 3-4 GI toxicity occurred. None of the patients had GI symptoms before start of RT. The symptoms generally consisted of increased frequency of bowel movement and change in stool consistency (diarrhea). Four patients (25%) did not experience any GI adverse effects during treatment. Acute Grade 2 GU toxicity were reported by 8 (50%), while Grade 1 was the highest GU toxicity reported by 7. One patient experienced Grade 3 GU toxicity because of the need for a urinary catheter. 50% of the patients with a peak Grade 2 or higher toxicity score had the same toxicity score before start of RT. The GU symptoms generally consisted of increased urinary frequency and dysuria.
Discussion
In this report we have presented our planning procedures as well as our early clinical experiences from a study of integrated bladder and lymph node IMRT. The main finding was that use of IMRT reduced the doses and gEUD to important ORs such as the bowel and rectum. At the same time, target conformity and homogeneity were improved. The early clinical outcome in terms of acute toxicity was also promising.
In this study the regional lymph nodes were included in the treated target volume, due to high risk of lymph node metastasis. In patients treated by radical cystectomy, metastases to the lymph nodes were observed in 30%, increasing with tumour stage Citation[20]. However, inclusion of the lymph nodes is not standard treatment in all countries and has never been tested in a randomized setting. Limited evidence exists on the performance of integrated IMRT for bladder and lymph nodes in terms of DVH dosimetrics and gEUD of the ORs. Most studies evaluating normal tissue sparing of IMRT versus CRT in the pelvis have been performed on prostate cancer. In a study by Muren et al., IMRT reduced the volume of intestine receiving more than 40 Gy from 139 to 89 cm3 and 50 Gy from 81 to 19 cm3, no Grade 3 toxicity and only 28% of patient's experienced Grade 2 GI toxicity Citation[21]. Similar results were observed in a study from Ashman et al., were 13 of 27 prostate patients received IMRT to the regional lymph nodes (prescription dose 45 Gy). The volume of intestinal dose over 45 Gy was reduced from 23 to 9% and only one patient experienced acute Grade 2 GI toxicity Citation[22].
The present study demonstrates a statistical significant reduction in gEUD for the bowel (from 58 to 53 Gy) and rectum (from 55 to 53 Gy). In a comparable study by Hysing et al. on prostate cancer where the target also included the regional lymph nodes, the gEUD using IMRT was 40 Gy and 46 Gy for the bowel and rectum, respectively Citation[13]. The difference in gEUD between our study and the study by Hysing et al. reflects the different volumes of normal tissue included in the PTV for prostate and bladder RT, respectively. We also showed that a dose of 60 Gy prescribed to the PTV1 using CRT resulted in a bowel gEUD of 52 Gy, which compares well with that achieved by an IMRT plan covering both the PTV1 and PTV2 (gEUD 53 Gy). In terms of gEUD to the bowel, the lymph nodes can therefore be included as a secondary target at the expense of a 1 Gy increase in gEUD. It should, however, be mentioned that the gEUD calculations presented do not take the fractionation effects of simultaneous integrated boost (SIB) IMRT into account Citation[23]. The actual normal tissue sparing of SIB IMRT when also including fractionation effects could therefore be even larger.
The acute toxicity presented in our study is limited by the small number of patient's and the short follow-up. Consequently, comparison with previous studies could be misleading. However, the results are encouraging since the acute GI toxicity is at least comparable to toxicity observed in a study on conformal RT of bladder cancer were only the bladder was the target Citation[24]. IMRT may allow the inclusion of the lymph nodes without increasing the acute toxicity as suggested in a study on treatment of prostate cancer Citation[25].
In the present study, treatment planning as well as calculation of dose volume parameters to ORs was based on a single snap-shot dose planning CT scan. However, it is well known that the ORs, and especially the bowel will change position between treatment fractions Citation[26]. In the study by Hysing et al., the IMRT sparing of the bowel and rectum persisted when the intra-fractional movements were analyzed on repeated CT scans Citation[13]. Because of limited accuracy and definition of the targets and ORs, comparison with other studies is difficult, and the correlation between gEUD and clinical outcome may be problematic. Therefore, clinical studies with careful follow-up on tumour control, survival and toxicity outcomes using standardized scoring system along with data on the treatment plans are warranted.
The use of daily image guidance with on-board target correction may allow us to reduce the margins. A study by Redpath et al. using an image-guided RT (IGRT) strategy with setup correction of translational errors, demonstrated that this technique allowed a reduction of the safety margin around the bladder Citation[11]. IGRT not only allow correction of setup-errors based on bone or soft tissue, but could also be used in an online/offline adaptive strategy where treatment is re-planned or adjusted according to the immediate position and conformity of the targets and ORs. With daily isocenter correction, it was possible to half the volume of OAR included by the PTV (Wright, P – personal communication). Using cone-beam CT and online adaptive RT strategies in bladder cancer may allow reduction of target margin and minimize the high dose volume to the ORs considerably Citation[27], Citation[28].
We are currently developing strategies for adaptive RT of bladder cancer which includes a boost to the tumour. To visualize the tumour we are evaluating tumour demarcation using contrast enhancement with injection of Lipiodol into the bladder wall. This may allow on-line corrections based on actual tumour position.
In conclusion, a simultaneous treatment approach of bladder and lymph nodes using IMRT results in considerable sparing of the bowel and the rectum. Studies combining IMRT with image-guidance in bladder cancer, including adaptive strategies may allow us to reduce margins with subsequent increase of radiation dose. This may result in improved local control with the lowest possible toxicity.
Acknowledgements
This study was supported by grants from The Danish Cancer Society, The Danish Graduate School for Clinical Oncology and Varian Medical Systems (Varian Oncology Systems, Palo Alto, CA, USA).
References
- Intensity Modulated Radiation Therapy Collaborative Working Group. Intensity-modulated radiotherapy: Current status and issues of interest Int J Radiat Oncol Biol Phys 2001;51:880–914.
- Nutting CM, Convery DJ, Cosgrove VP, Rowbottom C, Pachani AR, Webb S, et al. Reduction of small and large bowel irradiation using an optimized intensity-modulated pelvic radiotherapy technique in patients with prostate cancer. Int J Radiat Oncol Biol Phys 2000; 48: 649–56
- Jani AB, Su A, Milano MT. Intensity-modulated versus conventional pelvic radiotherapy for prostate cancer: Analysis of acute toxicity. Urology 2006; 67: 147–51
- Muren LP, Redpath AT, McLaren D, Mclaren D, Rorvik J, Halvorsen OJ, et al. A concomitant tumour boost in bladder irradiation: Patient suitability and the potential of intensity-modulated radiotherapy. Radiother Oncol 2006; 80: 98–105
- Wang-Chesebro A, Xia P, Coleman J, Akazawa C, Roach III M. Intensity-modulated radiotherapy improves lymph node coverage and dose to critical structures compared with three-dimensional conformal radiation therapy in clinically localized prostate cancer. Int J Radiat Oncol Biol Phys 2006; 66: 654–62
- Sengeløv L, von der Maase H. Radiotherapy in bladder cancer. Radiother Oncol 1999; 52: 1–14
- Fokdal L, Høyer M, von der Maase H. Treatment outcome and prognostic variables for local control and survival in patients receiving radical radiotherapy for urinary bladder cancer. Acta Oncol 2004; 43: 749–57
- Fokdal L, Høyer M, Meldgaard P, von der Maase H. Long-term bladder, colorectal, and sexual functions after radical radiotherapy for urinary bladder cancer. Radiother Oncol 2004; 72: 139–45
- Muren LP, Smaaland R, Dahl O. Organ motion, set-up variation and treatment margins in radical radiotherapy of urinary bladder cancer. Radiother Oncol 2003; 69: 291–304
- Fokdal L, Honore H, Høyer M, Meldgaard P, Fode K, von der Maase H. Impact of changes in bladder and rectal filling volume on organ motion and dose distribution of the bladder in radiotherapy for urinary bladder cancer. Int J Radiat Oncol Biol Phys 2004; 59: 436–44
- Redpath AT, Muren LP. CT-guided intensity-modulated radiotherapy for bladder cancer: Isocentre shifts, margins and their impact on target dose. Radiother Oncol 2006; 81: 276–83
- Niemierko A. Reporting and analyzing dose distributions: A concept of equivalent uniform dose. Med Phys 1997; 24: 103–10
- Hysing LB, Skorpen TN, Alber M, Fjellsbø LB, Helle SI, Muren LP. On the influence of organ motion on conformal versus intensity-modulated pelvic radiotherapy for prostate cancer. Int J Radiat Oncol Biol Phys 2008; 71: 1496–1503
- Roeske JC, Bonta D, Mell LK, Lujan AE, Mundt AJ. A dosimetric analysis of acute gastrointestinal toxicity in women receiving intensity-modulated whole-pelvic radiation therapy. Radiother Oncol 2003; 69: 201–7
- Söhn M, Yan D, Liang J, Meldolesi E, Vargas C, Alber M. Incidence of late rectal bleeding in high-dose conformal radiotherapy of prostate cancer using equivalent uniform dose-based and dose-volume-based normal tissue complication probability models. Int J Radiat Oncol Biol Phys 2007; 67: 1066–73
- Wu Q, Mohan R, Morris M, Lauve A, Schmidt-Ulrich R. Simultaneous integrated boost intensity-modulated radiotherapy for locally advanced head-and-neck squamous cell carcinomas. I: Dosimetric results. Int J Radiat Oncol Biol Phys 2003; 56: 573–85
- Dice LR. Measures of the amount of ecologic association between species. Ecology 1945; 26: 297–302
- Cox JD, Stetz J, Pajak TF. Toxicity criteria of the radiation therapy oncology group (RTOG) and the European organization for research and treatment of cancer (EORTC). Int J Radiat Oncol Biol Phys 1995; 31: 1341–6
- Koper PCM, Stroom JC, van Putten WLJ, Korevaar A, Gert A, Heijmen BJM, et al. Acute morbidity reduction using 3DCRT for prostate carcinoma: A randomized study. Int J Radiat Oncol Biol Phys 1999; 43: 727–34
- Knap MM, Lundbeck F, Overgaard J. The role of pelvic lymph node dissection as a predictive and prognostic factor in bladder cancer. Eur J Cancer 2003; 39: 604–13
- Muren LP, Wasbø E, Helle SI, Hysing LB, Karlsdottir A, Odland OH, et al. Intensity-modulated radiotherapy of pelvic lymph nodes in locally advanced prostate cancer: Planning procedures and early experiences. Int J Radiat Oncol Biol Phys 2008; 71: 1034–1041
- Ashman J, Zelefsky M, Hunt M, Leibel SA, Fuks Z. Whole pelvic radiotherapy for prostate cancer using 3D conformal and intensity-modulated radiotherapy. Int J Radiat Oncol Biol Phys 2005; 63: 765–71
- Park CS, Kim Y, Lee N, Bucci KM, Quivey JM, Verhey CJ, et al. Method to account for dose fractionation in analysis of IMRT plans: Modified equivalent uniform dose. Int J Radiat Oncol Biol Phys 2005; 62: 925–32
- Tonoli S, Bertoni F, De Stefani A, Vital E, De Tomasi D, Caraffini B, et al. Radical radiotherapy for bladder cancer: Retrospective analysis of a series of 459 patients treated in an Italian institution. Clin Oncol 2006; 18: 52–9
- Guckenberger M, Baier K, Richter A et al Does intensity modulated radiation therapy (IMRT) prevent additional toxicity of treating the pelvic lymph nodes compared to treatment of the prostate only? Radiat Oncol 2008; Article No. 3.
- Hysing LB, Kvinnsland Y, Lord H, Muren LP. Planning organ at risk volume margins for organ motion of the intestine. Radiother Oncol 2006; 80: 349–54
- Burridge N, Amer A, Marchant T, Sykes J, Stratford J, Henry A, et al. Online adaptive radiotherapy of the bladder: Small bowel irradiated-volume reduction. Int J Radiat Oncol Biol Phys 2006; 66: 892–7
- Pos FJ, Hulshof M, Lebesque J, Lots H, Van Tienhoven G, Moonen L, et al. Adaptive radiotherapy for invasive bladder cancer: A feasibility study. Int J Radiat Oncol Biol Phys 2006; 64: 862–8