Abstract
Purpose. The purpose of this study is to investigate the use of PET/CT with fluorodeoxyglucose (FDG), fluorothymidine (FLT) and fluoromisonidazole (FMISO) for radiotherapy (RT) target definition and evolution in rectal cancer. Materials and methods. PET/CT was performed before and during preoperative chemoradiotherapy (CRT) in 15 patients with resectable rectal cancer. PET signals were delineated and CT images on the different time points were non-rigidly registered. Mismatch analyses were carried out to quantify the overlap between FDG and FLT or FMISO tumour volumes (TV) and between PET TVs over time. Results. Ninety sequential PET/CT images were analyzed. The mean FDG, FLT and FMISO-PET TVs showed a tendency to shrink during preoperative CRT. On each time point, the mean FDG-PET TV was significantly larger than the FMISO-PET TV but not significantly larger than the mean FLT-PET TV. There was a mean 65% mismatch between the FMISO and FDG TVs obtained before and during CRT. FLT TVs corresponded better with the FDG TVs (25% mismatch before and 56% during CRT). During CRT, on average 61% of the mean FDG TV (7 cc) overlapped with the baseline mean TV (15.5 cc) (n=15). For FLT, the TV overlap was 49% (n=5) and for FMISO only 20% of the TV during CRT remained inside the contour at baseline (n=10). Conclusion. FDG, FLT and FMISO-PET reflect different functional characteristics that change during CRT in rectal cancer. FLT and FDG show good spatial correspondence, while FMISO seems less reliable due to the non-specific FMISO uptake in normoxic tissue and tracer diffusion through the bowel wall. FDG and FLT-PET/CT imaging seem most appropriate to integrate in preoperative RT for rectal cancer.
Rectal cancer patients at higher risk for local recurrence, in particular patients with a positive circumferential resection margin (CRM), could benefit from higher radiotherapy (RT) doses, provided this is associated with an acceptable toxicity Citation[1–3]. This can be achieved with 3D conformal and intensity modulated RT techniques.
Functional imaging is a promising tool for the detection of specific areas in the tumour that appear more radiation resistant Citation[4]. Moreover, it can provide us with the heterogeneity in the spatial distribution of biological characteristics, which can be targeted with non-uniform customized dose distributions Citation[5].
The use of PET/CT with fluorodeoxyglucose or 18F-FDG (FDG) and MRI for tumour volume (TV) definition has recently been reported by our group Citation[6]. Although FDG-PET has a high sensitivity in rectal Cancer, there are some limitations in specificity, mainly due to the uptake of FDG in macrophages. This Limitation becomes important when assessing tumour response to chemoradiation (CRT), as radiation Induces mucositis of the rectal wall surrounding the tumour.
Efforts have been made to develop new 18F-labeled tracers that are more tumour specific. Fluorothymidine or 18F-FLT (FLT) images cellular proliferation by entering the salvage pathway of DNA synthesis. It has been investigated for detection of various cancer types, including lymfoma, sarcoma, melanoma, lung, breast, brain and colorectal cancer (CRC) Citation[7].
Francis et al. found a 100% sensitivity in detecting primary CRC and concluded that FLT was a valuable tracer for the detection of CRC Citation[8]. No correlation could be found between the uptake of FLT and FDG, confirming that FLT and FDG reflect distinct processes Citation[8].
Hypoxia has been well documented as a significant obstacle to successful treatment with radiation Citation[9]. Therefore, it would be highly interesting to locate the hypoxic regions in the tumour and target them with higher RT doses. However, assessing tumour hypoxia is challenging. Most invasive methods lack consistency and reproducibility or have technical limitations that prohibit wide acceptance. Non-invasive ways of measuring hypoxia have been tested, including PET imaging with fluoromisonidazole or 18F-FMISO (FMISO). This tracer selectively binds to hypoxic cells. It has been tested for quantitative assessment of tumour hypoxia in lung, brain, head and neck cancer (HNC) and soft tissue sarcoma patients Citation[10–12]. One study showed that FMISO-PET imaging can detect hypoxia in rectal cancer patients Citation[13]. Voxel-based comparison of FMISO with FDG uptake in HNC and soft tissue sarcoma patients showed a poor correlation between FDG and FMISO uptake in corresponding FDG-defined TVs, as well as a mixture of characteristic patterns of distributions with a large inter-patient correlation variability Citation[14], Citation[15].
Recently, repeated imaging during RT has been investigated to track geometrical and biological changes of the tumour and adapt the treatment plan accordingly Citation[16]. Biological changes in response to neoadjuvant chemoradiation have been evaluated with FDG-PET in patients with locally advanced rectal cancer Citation[6], Citation[17]. Likewise, several studies have been published on the reproducibility of FMISO distribution in the tumour in HNC Citation[18], Citation[19] and non-small cell lung cancer (NSCLC) Citation[20], showing variance in intra-tumour distribution. Repeating FLT-PET imaging during RT is of interest because it does not accumulate in inflammatory cells and thereby provides a more specific tumour label compared to FDG. Preclinical data suggest that FLT can detect dose-dependent changes soon after irradiation in murine breast cancer cells Citation[21]. No clinical data are available on the assessment of FLT during or after RT for rectal cancer.
The main purpose of this study was to investigate the role of three PET tracers (FDG, FMISO and FLT), which provide different biological tumour characteristics, for RT target definition in rectal cancer. We also assessed the spatial distribution of the different PET signals and the quantitative and spatial evolution of the PET TVs during radiation.
Patients and methods
Patient selection
Between May 2005 and August 2007, 15 patients were analysed. Patient and treatment characteristics have been described in detail in a previous article Citation[6]. In brief, all patients had biopsy-proven resectable adenocarcinoma of the rectum, clinical stage T2/3-N1/2M0 on MRI and/or rectal endosonography. All patients were treated with a long course of preoperative chemoradiation (CRT) consisting of 25 fractions of 1.8 Gy, 5 days a week, during 5 weeks, in combination with a continuous infusion of 5-fluorouracil (5-FU) (225 mg/m2). During simulation and treatment, patients were positioned in prone position on a belly board device. Six to 8 weeks after completion of CRT, patients were operated. All patients underwent radical rectum resection with a total or partial mesorectal excision.
Image acquisition
Imaging was performed before and after 10 fractions of CRT in each patient. All patients underwent PET/CT imaging with 2 different tracers. Both PET/CT scans were planned with an interval of at least 24 hours between both injections. In 10 patients, FDG and FMISO were used. Five other patients were injected with FDG and FLT. In most of the patients, FMISO-PET/CT was performed one or two days before FDG-PET/CT. FLT-PET/CT imaging was acquired the day before or after FDG-PET/CT, except for 2 scans with a 5 or 6 days interval (during CRT).
PET/CT studies were acquired with a Siemens Biograph 2 scanner (Siemens®, Erlangen, Germany), consisting of a full ring PET and a dual-slice helical CT (4 patients), or with a Siemens HiRez Biograph scanner (Siemens®, Erlangen, Germany), consisting of a full ring PET and a 16 slice helical CT (11 patients). The PET transaxial field of view (FOV) was 585 mm/162 mm for both PET/CT scanners. The transaxial and axial PET resolution for the HiRez Biograph PET camera, represented by the full width at half maximum (FWHM), was 4.6 mm at 1 cm and 5.8 mm at 10 cm from the center. The Biograph 2 PET camera has a transaxial resolution of 6.3 mm and 7.4 mm and an axial resolution of 5.8 mm and 7.1 mm at 1 cm and 10 cm source distance respectively. For the HiRez Biograph PET images, the reconstruction was done with the system software, using Fourier rebinning and 2D-OSEM; 5 iterations with 8 subsets were used. For data of the Biograph 2 (Ecat Accel), 3D-OSEM was applied using off-line reconstruction software (developed at UCL by C. Michel and M. Sibomana). Only 10 iterations with 2 subsets were applied to prevent excessive noise propagation. The reconstructed images were filtered and processed as described in Citation[22].
FDG-PET/CT
Specific details on the FDG acquisition protocol have been fully reported in a previous article Citation[6]. A dose between 282 MBq (min) and 404 MBq (max) 18F-FDG was administered intravenously (injected dose=(body weight×4)+ 20), sixty minutes before scanning. Each PET/CT scan prior to treatment was performed using a high dose CT with IV contrast, since these images were used for RT treatment planning. CT scans during CRT were obtained with low doses and without IV contrast. The emission scan was obtained in 3D mode, covering the region of interest (ROI) (from L4 to the buttock folds) in 2 or 3 bed positions with a scan time of 4 minutes (min.) per bed position. All PET/CT acquisitions were performed with the patient in treatment position on a belly board device.
FMISO-PET/CT
Four patients were examined on the Biograph 2 and 6 patients on the HiRez Biograph PET/CT. No fasting protocol was recommended. FMISO was injected intravenously at a dose of 370 MBq (range: 330–398 MBq). To allow optimal accumulation of the tracer in the hypoxic cells, a time interval of 3 hours was respected between injection and scan. At each time point, a low dose CT scan was obtained without IV contrast. Other CT characteristics were identical to the FDG-PET/CT. The Biograph 2 FMISO scan was performed in a 3D mode with 4 min. per bed position. The HiRez Biograph FMISO scan was acquired in a 3D mode with 4 min. per bed position in 1 patient and a 10 min. per bed position in the 5 other patients. Total acquisition time was about 45 min.
FLT-PET/CT
All 5 patients were examined on the HiRez Biograph PET/CT. Patients were asked to fasten for 6 hours before the examination. FLT was injected intravenously at a dose of 370 MBq (range: 328–470 MBq) one hour before the scan started. At each time point, a low dose CT scan was obtained without IV contrast. Other CT characteristics were identical to the FDG-PET/CT. The HiRez Biograph FLT scan was acquired in a 3D mode with 4 min. per bed position in the 5 patients. Total acquisition time was about 45 min.
Delineation of tumour volumes
FDG, FMISO and FLT-PET images were automatically processed and outlined by use of a gradient-based segmentation method. This method was developed at UCL (Université Catholique de Louvain, Belgium) and has been described and validated for segmentation of PET images in head and neck cancer (HNC) patients Citation[22]. Details on the processing and reconstruction of PET images and on the segmentation procedure have been described elsewhere Citation[6]. For the FMISO images, a ROI was used, related to the corresponding FDG TV, since a large background activity of FMISO in the rectum prohibits an accurate and correct differentiation between tumour uptake and physiological uptake in the bowel. Non-rigid registration of the FDG with the FMISO-PET allowed us to limit the segmentation to this transferred FDG-based ROI. However, most patients presented with a FMISO signal extending outside this ROI. In those cases, the corresponding CT data were used to evaluate whether the delineated FMISO signal still projected within the suspected tumour area.
Nonparametric statistics were used to compare TV (Wilcoxon Matched Pairs Test) and to correlate TV (Spearman Rank Order Correlations).
Image coregistration
We have applied a non-rigid image registration algorithm using a B-spline transformation model and mutual information (MI) constraints. Details of this registration process have been described previously Citation[23]. Registration of the different PET/CT images was performed on the CT intensities, but all PET images and delineations could be deformed according to the resulting deformation field. A small smoothness penalty was used to promote a regular deformation Citation[24]. By limiting the calculation of the mutual information to the region inside the mesorectum the influence from the bladder was minimized. In the last multiresolution stage a volume penalty was used to limit the volume change of the tumour when registering images over time Citation[25].
A mismatch analysis of TVs was performed between FDG and FLT or FMISO-PET/CT and between the different time points. The mismatch between a certain volume A and a certain volume B is defined as the percentage of A that does not belong to B. It is 0% if A falls entirely inside B and is 100% if A and B do not overlap. In order to quantify the spatial extent of the mismatch between volumes A and B, we calculated the mean (Dmean) and maximal (Dmax) distance to the intersection of A and B over all points in the mismatch of A to B (). In case no FMISO signal could be detected, no mismatch analysis was performed.
Figure 1. Maximum of shortest distances between mismatch volume (A-B volume) and intersection (A⋂B) (Dmax): the maximum of all shortest distances between A-B volume (P1,2, … ,Pn) and A⋂B (arrow from P4). Mean of shortest distances between mismatch volume (A-B volume) and intersection (A⋂B) (Dmean): mean of all shortest distances between A-B volume (P1, P2, … ,Pn) and the intersection (A⋂B).
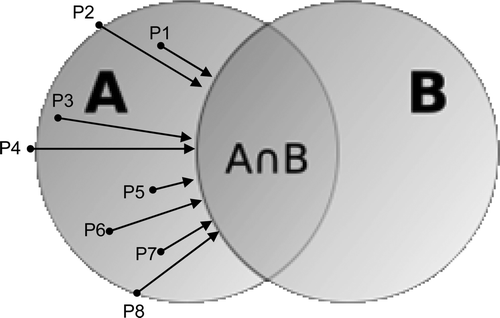
Results
Tumour volume analysis
We have analyzed 90 sequential PET/CT images from 15 patients (). FDG and FLT TVs could be delineated on each time point. In one patient, no baseline FMISO signal was seen within the tumour region, whereas during CRT, a signal appeared. In 3 patients, the FMISO signal disappeared on the scan during CRT.
Table I. Absolute (cc) and relative (%) FDG and (a) FMISO- or (b) FLT-PET tumour volumes measured before and during chemoradiotherapy (a: n = 10, b: n = 5). PET tumour volumes were obtained with a gradient-based segmentation method. CRT: chemoradiotherapy, PAT: patient.
The mean FDG-PET TV before and during CRT was significantly larger than the FMISO-PET TV (p < 0.001). There was no significant difference between the mean FDG TV and the mean FLT TV. Although most FDG TVs were larger, in 2 of 5 patients, the baseline FLT was similar or larger than the FDG TV (patient 6 and 7). This was also the case in 2 patients during CRT (patient 6 and 10).
TVs during CRT were significantly smaller than the baseline TV for all three PET tracers (p < 0.05) (). The relative TV reduction after 10 fractions of CRT was 54% for FDG-PET (n = 15), 58% for FLT-PET and 66% for FMISO-PET.
Figure 2. Box-whisker plot of FDG and FMISO-PET (a) and FLT-PET (b) tumour volumes measured before and during chemoradiotherapy (a: n = 10, b: n = 5). PET tumour volumes were obtained with a gradient-based segmentation method. RT: radiotherapy, SE: standard error, SD: standard deviation.
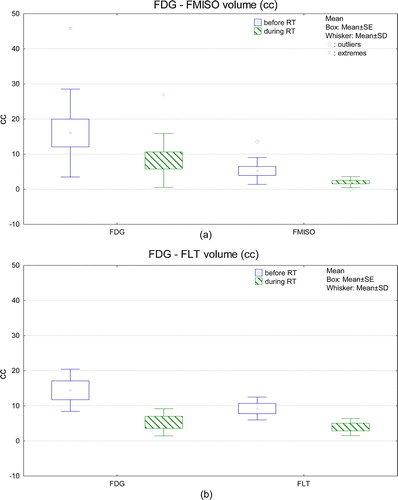
No correlation was found between the FDG TV and the FMISO or FLT TVs.
Mismatch analysis
Mismatch between different PET TVs per time point
Since different PET tracers were used in order to better define the potential target volumes, it is important to know their spatial relationship. FDG and FMISO or FLT-PET TV are illustrated for 3 patients with a good, average and poor overlap, respectively ().
Figure 3. FDG-PET (blue) and corresponding FMISO (red) (a) or FLT (red) (b) tumour contours in axial, sagittal and coronal plane for 3 patients, showing a good, average and poor overlap between both imaging modalities (from top to bottom).
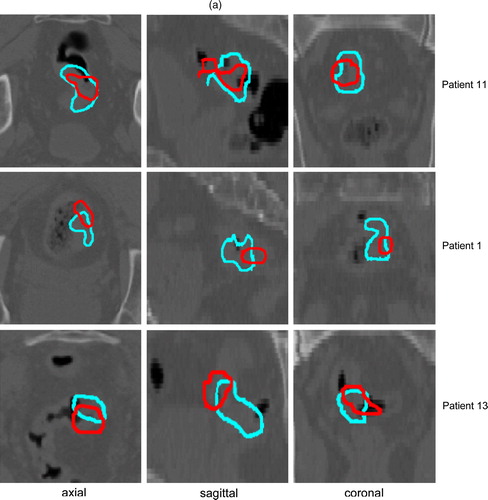
If the FDG TV is taken as reference volume, a mean 25% (median: 23%) FLT mismatch was found at baseline and a 56% (median: 69%) mismatch during CRT (). Compared to FLT, the mismatch between FMISO and FDG (reference volume) was much larger before CRT (mean: 65%, median: 68%). During CRT, the mean mismatch between FMISO and FDG (reference volume) was 65% (median, 65%). The difference in overlap between FLT- and FMISO-based TVs with the corresponding FDG TV at baseline was translated in a clearly different Dmax (6mm for FLT and 14mm for FMISO) and Dmean (2mm and 6mm, respectively) (). During CRT, similar distances were measured between FDG and FLT TVs or FDG and FMISO TVs (Dmax: 10mm; Dmean: 4mm).
Table II. Mismatches (%) (mean and range [min-max]) between FDG and FMISO-PET (a) or FLT-PET (b) tumour volumes per time point and between time points. B is the reference volume.
Table III. Mean (±standard deviation) value of the maximum (Dmax) and the mean (Dmean) of the shortest distances (mm) between corresponding FDG and FLT (a) or FDG and FMISO (b) tumour volumes (TV) before and during CRT and between TVs during CRT and baseline TVs on FDG, FLT and FMISO PET/CT (c).
Mismatch between PET TVs before and during CRT per PET tracer
The mean mismatch results after registration of the first and the second time point are shown in for each PET/CT set. Considering all 15 FDG-PET/CT scans, a mismatch of almost 40% (median 33%) was calculated for the TV during CRT compared to baseline (reference volume) (). For FLT, this mismatch was approximately 50% (median: 55%) and for FMISO 80% (median: 90%). Corresponding Dmax and Dmean between the contours during and before CRT were comparable for FDG and FLT (c). The higher mismatch result for FMISO TVs is in agreement with the larger Dmax and Dmean. The evolution of the FMISO or FLT signal within time in relation to the evolution of the FDG signal is illustrated for 2 patients in .
Discussion
This study investigated the use of PET/CT with FDG, FLT and/or FMISO for radiotherapy (RT) target definition and evolution in rectal cancer. Rectal cancer patients could benefit from higher RT doses to (sub)volumes that appear more radiation resistant, provided this is associated with an acceptable toxicity Citation[1–3]. This type of high precision irradiation requires a good definition, delineation and location of the target volumes.
Tumour volume analysis
In a previous article, the advantages and limitations of FDG-PET images and the applied FDG-PET segmentation methods were discussed Citation[6]. Two delineation algorithms were tested; an adaptive threshold-based method Citation[26] and a gradient-based method Citation[22]. Our results confirmed that the latter segmentation technique provides improved accuracy for TV delineation, based on validation with the pathological TV. Since the method does not depend on the PET tracer and the PET camera, it was chosen for the segmentation of FLT and FMISO-PET images Citation[27].
Most studies that investigate FMISO for RT target definition use a standardised uptake value (SUV) threshold-based segmentation technique. Generally, a tumour-to-blood or tumour-to-muscle SUV ratio of 1.2 or higher is applied as a cut-off between normoxic and hypoxic tissues, as suggested by Rajendran et al. Citation[15]. Using a fixed SUV threshold to identify hypoxic regions has limitations. In 4 of our patients, this delineation method resulted in a larger FMISO TV compared to FDG, resulting in a complete hypoxic FDG TV.
There are two major limitations related to FMISO-PET imaging. One is the limited contrast between the trapping of FMISO by tumours in comparison with normal tissues due to the relatively low cellular uptake of FMISO. The other limitation is the slow cellular washout of tracers in non-hypoxic tissue. Therefore a delay of approximately 2 to 3 hours after injection of FMISO is needed to permit clearance of this tracer from normal background tissues. In CRC, there seems to be another drawback related to a high background activity of FMISO in the normal rectum. This has been reported in the GI tract of rat tumour models Citation[28], and also in colorectal cancer patients Citation[13] and is confirmed in our study. Moreover, colonic excretion of the tracer was also seen in some of our patients, as has been described by Loi S et al. Citation[13]. Therefore, the FDG-defined TV was used to focus the FMISO segmentation on the tumour region.
In most FLT studies, a semi-quantitative analysis of tracer uptake is carried out using SUVs. The delineation is then performed by constructing for instance a 50% isocontour of the maximum SUV Citation[29]. Other authors used a visual interpretation of the PET signal, starting with a fixed window/level according to a gray intensity scale and allowing adjustment of this scale according to the judgement of the observer Citation[30]. This method can obviously change the size of the TV depending on the applied PET window/level and can explain the relatively large PET TVs obtained in this study compared to CT.
Limitations related to FLT-PET imaging mostly come from the interaction of the tracer with chemotherapeutic agents, like 5-FU, which can occur early after chemotherapy. This drawback has been used to explain the mixed results in studies on the value of FLT for early response assessment. Combined use of 5-FU might obscure the effective proliferation changes and precludes accurate interpretation of the effects of RT Citation[31]. In our study, we saw a clear reduction in physiological FLT uptake in the bone marrow that was included in the radiation field (hips and lumbar spine) after 10 fractions of RT. This finding contrasts with the increase in FLT accumulation after 5-FU administration as observed during chemotherapy for oesophageal cancer Citation[31]. But the latter study was performed on oesophageal cell lines treated to relatively high doses of 5-FU compared to the doses used in this patient study and before the cells were exposed to FLT.
Our results show that the mean FDG-PET TV before and during CRT was significantly larger than the FMISO-PET TV (p < 0.001). No correlation was found between the FMISO and the FDG TV. Loi et al. only performed a qualitative interpretation of the FMISO uptake within a FDG-defined TV on pretreatment PET images. Evidence of hypoxia was defined as a higher uptake of FMISO compared to the cardiac blood pool and normal bowel activity. They found detectable hypoxia in 4 of 6 patients. No absolute FMISO TVs were calculated and no correlation with FDG was performed Citation[13].
The mean FLT TV was more comparable, but still smaller than the mean FDG TV on both time points. No correlation was found between the FLT TV and the FDG TV. These findings confirm the results of Francis et al., who demonstrated smaller FLT TVs and lower FLT SUV values compared to FDG Citation[8]. Patel and al. evaluated the impact of combined FDG and FLT-PET/CT on inter-observer variability of target volume delineation in rectal cancer Citation[30] against CT. In 5 of the 6 patients enrolled, the FDG and FLT TV was larger than the CT TV. The inter-observer similarity index (SI) for the primary GTV, improved modestly for FDG-PET/CT and also slightly for FLT-PET/CT compared to CT alone. There was no difference in SI when FLT-based TVs were compared to FDG-based TVs. The authors report the greatest impact of the PET information on the superior/inferior extent of the primary TV. It should be noted that improving the agreement in target definition does not necessarily result in improved target definition. Firstly, accurate target definition requires reliable and validated delineation methods, especially for PET images. In the study of Patel et al., the contouring of the PET signals was based on a visual interpretation by adjusting a predefined window level. The drawback of this method has been discussed earlier. Secondly, the use of different imaging modalities for target definition requires accurate registration techniques. In their study, FLT-PET/CT and FDG-PET/CT images were registered manually and rigidly with the aid of surface fiducial markers visible on PET and CT. This method does not take into account the large internal organ variation that exists between two examinations, even if they are performed in the same patient position.
When the evolution of the PET TV is considered over time, we found a significant decrease after 10 fractions of RT for the FDG TV (n = 15, p < 0.001), FMISO TV (p < 0.05) and FLT TV (p < 0.05), suggesting that reoxygenation and reduced proliferation took place in a shrinking TV. No study has been reported in rectal cancer on the changes in tumour hypoxia during RT measured with FMISO-PET. However, this has been investigated in HNC Citation[18], Citation[19] and NSCLC Citation[20], showing quite some variance in intra-tumour FMISO distribution. Nehmeh at al. evaluated the correspondence of FMISO-PET intensities of corresponding spatial voxels within a FDG-defined ROI between 2 pretreatment FMISO-PET/CT scans Citation[19]. For most patients the fractional hypoxic volume (FHV) was consistent over both FMISO scans. However, when taking into account the spatial distribution of the signal, there was a poor voxel-by-voxel correlation between the SUV of the hypoxic zones over time. This variance in intra-tumour distribution can compromise the coverage of FMISO-based hypoxic TVs by dose-painting IMRT Citation[32]. Koh et al. analyzed 4 serial FMISO scans in 7 NSCLC patients and compared the FHV in the CT-based TV Citation[20]. They found no acute changes in FHVs following 1 or 2 fractions of RT. At mid-treatment, there was a general trend towards increased oxygenation with substantial residual FMISO uptake by the end of treatment in 5/6 patients. They also noted a clear heterogeneity within a plane and between different planes for each patient (no spatial correlation).
Mismatch analysis
As variation in patient position and additional organ movement and deformation hamper an accurate comparison of multi-temporal images, we aimed at bringing all images into a common reference frame by using a non-rigid registration technique. However, the registrations were quite challenging due to differences in position and shape of internal organs, especially variances in bladder and rectal filling. Carefully choosing the regularization parameters, in this case smoothness and volume penalties or limiting the registration to a certain part of the image assisted in facing these challenges.
The promising results of the validation of the non-rigid registration for monomodal registration have been described by our group Citation[6], Citation[23]. Perfect TV preservation cannot be expected due to ‘inaccuracies’ in PET/CT imaging. These include the projection of parts of the PET signal in air cavities and volume shifts between PET and CT caused by physiological processes during image acquisition. However, the actual TV change of the FDG, FMISO and FLT-PET signals induced by CRT was much larger than the TV changes found after registration of the different PET/CT images over time.
We showed that the overlap between FMISO and FDG TVs was worse compared to the FLT-FDG TV overlap at baseline, which translated in a clearly different Dmax and Dmean. This poor correspondence between FDG and FMISO is probably due to false positive signal uptake in the normal bowel or diffusion of FMISO tracer through the wall around the tumour region. Although we focussed the segmentation to a FDG-based ROI, most patients presented with FMISO signal extending this ROI, whereas the largest part of the FLT signal was located inside the FDG ROI. During CRT, the mismatch between FLT and FDG TVs increased to 56%. This is probably related to the small TVs as determined on both modalities. Small TVs are more influenced by delineation errors, registration errors and/or possible intrafraction organ deformation. Even with the gradient-based segmentation, small TVs and insufficient PET resolution are major limitations for accurate delineation Citation[27]. In addition, the overlap of the TVs obtained during CRT with the baseline TVs was better for FDG and FLT compared to FMISO, demonstrating that FMISO signal uptake is more variable over time. These findings favour the use of FDG and FLT as potential tracers for target volume definition in dose escalation RT. Moreover, the change in TV during CRT and the relative stable position over time support the use of FDG and FLT as a target for adaptive RT in rectal cancer.
In a previous article, we have shown that FDG-PET can substantially alter the anatomically-defined GTV Citation[6]. This can be explained by a better differentiation between tumour and benign soft tissue or by detection of small positive lymph nodes. However, mismatches can also be induced by registration errors when different non-integrated imaging modalities are used for target volume definition. With this study, we have proven that the use of other PET tracers induce additional volumetric and spatial changes. Since FDG, FLT and FMISO reflect different functional characteristics, they may be used for a different purpose in RT planning. FDG and FLT seem most appropriate for definition of a biological target volume, taking into account the small mismatches after registration of both image sets. In addition, the smaller FLT TV, potentially more sensitive for tumour tissue especially during RT, may be used as a subvolume inside the functional target volume that can benefit from even higher RT doses. The same rationale could hold for FMISO, since hypoxic cells are more radiation resistant. However, FDG and FMISO show a poor spatial correspondence and the FMISO signal uptake seems less stable over time compared to FDG and FLT.
Conclusion
Our results show that FDG, FLT and FMISO-PET signals reflect different functional characteristics that change during CRT. FLT and FDG show a good spatial correspondence, while FMISO seems less reliable, due to its non-specific uptake in normoxic tissue and tracer diffusion through the bowel wall. Moreover, the FMISO signal uptake seems less stable over time compared to FDG and FLT. Therefore, FDG and FLT-PET/CT imaging seem more appropriate to be integrated in preoperative RT for rectal cancer.
Acknowledgements
Sarah Roels is supported by a grant from the ‘Belgian Foundation against Cancer’ (‘Belgische Stichting tegen Kanker’) (Katholieke Universiteit Leuven: No SCIE2003-23 (ZKB2747), Sigrid Stroobants by a grant from the FWO-Vlaanderen, Credit G.0177.04 and Karin Haustermans is supported by a fundamental clinical mandate of the FWO. This work was supported in part by a grant from Varian Medical system. None of the authors has a conflict of interest in connection with the paper and the material isnot under publication or consideration for publication elsewhere.
References
- Movsas B, Hanlon AL, Lanciano R, Scher RM, Weiner LM, Sigurdson ER, et al. Phase I dose escalating trial of hyperfractionated pre-operative chemoradiation for locally advanced rectal cancer. Int J Radiat Oncol Biol Phys 1998; 42: 43–50
- Chan AK, Wong AO, Langevin J, Jenken D, Heine J, Buie D, et al. Preoperative chemotherapy and pelvic radiation for tethered or fixed rectal cancer: A phase II dose escalation study. Int J Radiat Oncol Biol Phys 2000; 48: 843–56
- Myerson RJ, Valentini V, Birnbaum EH, Cellini N, Coco C, Fleshman JW, et al. A phase I/II trial of three-dimensionally planned concurrent boost radiotherapy and protracted venous infusion of 5-FU chemotherapy for locally advanced rectal carcinoma. Int J Radiat Oncol Biol Phys 2001; 50: 1299–308
- Ling CC, Humm J, Larson S, Amols H, Fuks Z, Leibel S, et al. Towards multidimensional radiotherapy (MD-CRT): Biological imaging and biological conformality. Int J Radiat Oncol Biol Phys 2000; 47: 551–60
- Yang Y, Xing L. Towards biologically conformal radiation therapy (BCRT): Selective IMRT dose escalation under the guidance of spatial biology distribution. Med Phys 2005; 32: 1473–84
- Roels S, Slagmolen P, Nuyts J, Loeckx D, Maes F, Vandecaveye V, et al. A protocol for image-guided dose-escalation in rectal cancer. Int J Radiat Oncol Biol Phys, submitted
- Been LB, Suurmeijer AJH, Cobben P, Jager P, Hoekstra HJ, Elsinga PH. [18F]FLT-PET in oncology: current status and opportunities. Eur J Nucl Med Mol Imaging 2004; 31: 1659–72
- Francis DL, Visvikis D, Costa DC, Arulampalam THA, Townsend C, Luthra SK, et al. Potential impact of [18F]3′-deoxy-3′-fluorothymidine versus [18F]fluoro-2-deoxy-D-glucose in positron emission tomography for colorectal cancer. Eur J Nucl Med Mol Imaging 2003; 30: 988–94
- Nordsmark M, Overgaard M, Overgaard J. Pretreatment oxygenation predicts radiation response in advanced squamous cell carcinoma of the head and neck. Radiother Oncol 1996; 41: 31–9
- Koh WJ, Rasey JS, Evans ML, Grierson JR, Lewellen TK, Graham MM, et al. Imaging of hypoxia in human tumors with [F-18]fluoromisonidazole. Int J Radiat Oncol Biol Phys 1992; 22: 199–212
- Eschmann SM, Paulsen F, Reimold M, Dittmann H, Welz S, Reischl G, et al. Prognosticimpact of hypoxia imaging with 18F-misonidazole PET in non-small cell lung cancer and head and neck cancer before radiotherapy. J Nucl Med 2005; 46: 253–60
- Bruehlmeier M, Roelcke U, Schubiger PA, Ametamey SM. Assessment of hypoxia and perfusion in human brain tumors using PET with 18F-fluoromisonidazole and 15O-H2O. J Nucl Med 2004; 45: 1851–9
- Loi S, Ngan SY, Hicks RJ, Mukesh B, Mitchell P, Michael M, et al. Oxaliplatin combined with infusional 5-fluorouracil and concomitant radiotherapy in inoperable and metastatic rectal cancer: A phase I trial. Br J Cancer 2005; 92: 655–61
- Thorwarth D, Eschmann SM, Holzner F, Paulsen F, Thorwarth AM. Combined uptake of [18F]FDG and [18F]FMISO correlates with radiation therapy outcome in head-and-neck cancer patients. Radiother Oncol 2006; 80: 151–6
- Rajendran JG, Wilson DC, Conrad EU, Peterson LM, Bruckner JD, Rasey JS, et al. [(18)F]FMISO and [(18)F]FDG PET imaging in soft tissue sarcomas: Correlation of hypoxia, metabolism and VEGF expression. Eur J Nucl Med Mol Imaging 2003; 30: 695–704
- Balter JM, Kessler ML. Imaging and alignment for image-guided radiation therapy. J Clinic Oncol 2007; 25: 931–7
- Cascini GL, Avallone A, Delrio P, Guida C, Tatangelo F, Marone P, et al. 18F-FDG PET is an early predictor of pathologic tumor response to preoperative radiochemotherapy in locally advanced rectal cancer. J Nucl Med. 2006; 47: 1241–8
- Eschmann SM, Paulsen F, Bedeshem C, Machulla HJ, Hehr T, Bamberg M, et al. Hypoxia-imaging with (18)F-Misonidazole and PET: Changes of kinetics during radiotherapy of head-and-neck cancer. Radiother Oncol 2007; 83: 406–10
- Nehmeh SA, Lee NY, Schröder H, Squire O, Zanzonico PB, Erdi YE, et al. Reproducibility of intratumor distribution of (18)F-fluoromisonidazole in head and neck cancer. Int J Radiat Oncol Biol Phys 2008; 70: 235–42
- Koh WJ, Bergman KS, Rasey JS, Peterson LM, Evans ML, Graham MM, et al. Evaluation of oxygenation status during fractionated radiotherapy in human nonsmall cell lung cancers using [F-18] fluoromisonidazole positron emission tomography. Int J Radiat Oncol Biol Phys 1995; 33: 391–8
- Huang SC, McBride W, Stout D, Sitko J, Liao YP, Daigle J, et al. Post-irradiation temporal changes in glucose metabolism and cell proliferation in implanted murine tumors as measured by FDG and FLT PET. J Nucl Med 2002; 43: 25
- Geets X, Lee J, Bol A, Lonneux M, Grégoire V. A gradient-based method for segmenting FDG-PET images: Methodology and validation. Eur J Nucl Med Mol Imaging 2007; 34: 1427–38
- Slagmolen P, Loeckx D, Roels S. Non-rigid registration of multi-temporal CT and MR images for radiotherapy treatment planning. In: Pluim JPW, et al, Likar B, Gerritsen FA, WBIR 2006: Third International Workshop on biomedical image registration. Lecture Notes in computer science 4057. Berlin, Heidelberg: Springer-Verlag; 2006. p 297–305.
- Rueckert D, Sonoda LI, Hayes C, Hill DL, Leach MO, Hawkes DJ. Nonrigid registration using free-form deformations: Application to breast MR images. IEEE Trans Med Imaging 1999;18:712\u201321.
- Rohlfing T, Maurer CRJ, Bluemke DA, Jacobs MA. Volume-preserving nonrigid registration of MR breast images using free-form deformation with an incompressibility constraint. IEEE Trans Med Imaging 2003;22:730\u201341.
- Daisne JF, Sibomana M, Bol A, Doumont T, Lonneux M, Grégoire V. Tri-dimensional automatic segmentation of PET volumes based on measured source-to-background ratios: Influence of reconstruction algorithms. Radiother Oncol 2003; 69: 247–50
- Grégoire V, Bol A, Geets X, Lee J. Is PET-based treatment planning the new standard in modern radiotherapy? The Head and Neck paradigm. Semin Radiat Oncol 2006; 16: 232–8
- Dubois L, Landuyt W, Haustermans K, Dupont P, Bormans G, Vermaelen P, et al. Evaluation of hypoxia in an experimental rat tumour model by [(18)F]fluoromisonidazole PET and immunohistochemistry. Br J Cancer 2004; 91: 1947–54
- Troost EG, Vogel WV, Merkx MA, Slootweg PJ, Marres HA, Peeters WJ, et al. 18F-FLT PET does not discriminate between reactive and metastatic lymph nodes in primary head and neck cancer patients. J Nucl Med 2007; 48: 726–35
- Patel DA, Chang ST, Goodman KA, Quon A, Thorndyke B, Gambhir SS, et al. Impact of Integrated PET/CT on variability of target volume delineation in rectal cancer. Technol Cancer Res Treat 2007; 6: 31–6
- Dittmann H, Dohmen BM, Kehlbach R, Bartusek G, Pritzkow M, Sarbia M, et al. Early changes in [18F]FLT uptake after chemotherapy: An experimental study. Eur J Nucl Med 2002; 29: 1462–9
- Lin Z, Mechalakos J, Nehmeh S, Schoder H, Lee N, Humm J, et al. The influence of changes in tumor hypoxia on dose-painting treatment plans based on (18)F-FMISO positron emission tomography. Int J Radiat Oncol Biol Phys 2008; 70: 1219–28