Abstract
Purpose. Locally advanced cervical cancer is usually treated with external beam radiotherapy followed by brachytherapy (BT). However, if response or tumour topography is unfavourable it may be difficult to reach a sufficient BT dose. The purpose of this study was to explore whether an applicator guided stereotactic IMRT boost could be combined with brachytherapy to improve dose volume parameters. Material and methods. Dose plans of 6 patients with HR CTV volumes of 31–100cc at the time of BT were analysed. MRI was performed with a combined intracavitary (IC)-interstitial (IS) ring applicator in situ. A radiotherapy schedule consisting of 45Gy (1.8Gy×25) IMRT followed by boost of 28Gy (7Gy×4fx) was modelled. Four different boost techniques were evaluated: IC-BT, IC/IS-BT, IC-BT+IMRT and IMRT. Dose plans were optimised for maximal tumour dose (D90) and coverage (V85Gy) while respecting DVH constraints in organs at risk: D2cc <75Gy in rectum and sigmoid and <90Gy in bladder (EQD2). In combined BT+IMRT dose plans, the IMRT plan was optimised on top of the BT dose distribution. Volumes irradiated to more than 60 Gy EQD2 (V60Gy) were evaluated. Results. Median dose coverage in IC plans was 74% [66–93%]. By using IC/IS or IC-BT+IMRT boost, the median coverage was improved to 95% [78–99%], and to 96% [69–99%] respectively. For IMRT alone, a median coverage of 98% [90–100%] was achieved, but V60Gy volumes were significantly increased by a median factor of 2.0 [1.4–2.3] as compared to IC/IS. It depended on the individual tumour topography whether IC/IS-BT or IC-BT+IMRT boost was the most favourable technique. Conclusion. It is technically possible to create dose plans that combine image guided BT and IMRT. In this study the dose coverage could be significantly increased by adding IS-BT or IMRT boost to the intracavitary dose. Using IMRT alone for boost cannot be advocated since this results in a significant increase of the volume irradiated to 60Gy.
Standard radiotherapy for cervical cancer combines external beam radiotherapy (EBRT) with a boost of intracavitary (IC) brachytherapy (BT). Currently, there is a huge interest to include 3D image modalities in the planning of BT. The primary advantage of 3D IC-BT technique is the possibility to conform the dose given by BT to the anatomy of each patient, taking into account both the tumour regression obtained by external beam radiotherapy and the position of nearby organs at risk (OAR). It has been shown that 3D image based BT significantly improves the dose distribution Citation[1], Citation[2]. Recently, both European Citation[3], Citation[4] and American guidelines Citation[5] for 3D image based IC-BT have been published. To date only the European guidelines are supported by clinical data from studies in which systematic use of magnetic resonance imaging (MRI) based planning was used. Pötter et al. Citation[6] published strong indications that 3D image based brachytherapy has the potential to improve the local control for tumours while reducing the rate of gastrointestinal and urinary late morbidity (G3, G4). 3D IC-BT requires both a gynaecologic examination and 3D imaging with the BT applicator in situ. It is difficult to visualize the tumour with computed tomography (CT) and MRI is advocated as the preferred imaging modality Citation[3], Citation[7]. Tumour and OAR is delineated before every boost, and a dose optimisation is made, to optimise target coverage and to spare the OAR.
Prior studies show, that during radiotherapy of locally advanced cervical cancer the target volume changes position and size due to internal organ motion and tumour regression Citation[8], Citation[9]. According to Hatano et al. Citation[8] substantial tumour regression can be expected after having delivered 30 Gy. Van de Bunt et al. Citation[9] show that the average target volume after around 30 Gy was reduced from 71ccm to 39 ccm and that by replanning with IMRT plans they obtained a reduction of dose to the OAR. The substantial anatomical changes during radiotherapy of locally advanced cervical cancer underline the importance of investigating 4D image based dose planning where radiotherapy dose is adapted in both spatial and time domains for both OAR and tumour.
Large tumours with distal parametrial involvement at diagnosis, insufficient response, and/or unfavourable topography after radiochemotherapy represent a therapeutic challenge Citation[10]. In these cases it can be difficult to reach a sufficient IC-BT dose to the high risk-clinical target volume (HR-CTV). This problem is currently mainly solved in two different ways, parametrial boost or interstitial (IS)-BT. Parametrial boost is an EBRT boost where AP-PA fields are often used. IS-BT can be combined with IC-BT by placing needles in the parametrial region Citation[1], Citation[10], Citation[11].
In this dose planning study we have evaluated different boost techniques in large and/or topographically unfavourable tumours at the time of BT. The purpose of the study was to investigate whether an applicator guided IMRT boost could be used to improve tumour coverage. Different scenarios were investigated: IMRT-boost delivered instead of BT, and IMRT combined with IC-BT in order to boost those areas where the IC-BT does not give a sufficient dose.
Methods
MRI-based dose plans of 6 patients (1 IIB, 4 IIIB, 1 IVB) with large or topographically unfavourable tumours were analysed. All tumours involved the distal parametria or pelvic wall (unilaterally 2, bilaterally 4) with a median clinical tumor width of 8 cm (range 6–10) at diagnosis and 6 cm (range 5–8 cm) at time of BT. The HR-CTV and OAR (bladder, rectum and sigmoid) were contoured according to the GEC-ESTRO guidelines Citation[3], Citation[4]. The HR-CTV volumes in the 6 cases were 31, 44, 62, 80, 90 and 100 cm3, respectively
A radiation schedule of whole pelvic EBRT of 45Gy/25fx and boost of 28Gy/4fx was modelled. The prescribed dose was in total 84 EQD2 Gy (α/β = 10Gy).
A CT scan was obtained for planning of whole pelvis EBRT. The elective target (CTV-E) was contoured according to the recommendations of Taylor et al. Citation[12]. CTV-E included the GTV, uterus and whole cervix, lymph nodes in the parametria and along the external iliac, internal iliac, common iliac/aorta to the L4-L5 interspace, and ≥2 cm of the vagina below the tumour. Planning target volume (PTV) margin was 0.5 cm in anterior-posterior direction and lateral direction, and 0.9 cm in cranio-caudal direction. IMRT plans with 7 fields were produced with a homogeneous dose within 95 to 107% of the prescribed dose.
MR-scan for dose planning of the brachytherapy boost was performed with a combined intracavitary/interstitial ring applicator in situ after delivery of around 40Gy whole pelvic EBRT. Interstitial needles were inserted through steering holes drilled in the ring cap in an approach similar to the Vienna applicator described by Dimopoulos et al. Citation[10] and Kirisits et al. Citation[11]. Furthermore, in three patients, free needles were inserted in target regions which could not be reached by needles placed through the ring. The target and OARs (bladder, rectum and sigmoid) were contoured on T2 weighted sequences of the MR-scan. The boost target was the HR-CTV which includes the whole cervix and the presumed extracervical tumour extension at time of BT Citation[3]. The MRI was matched with the planning CT-scan in order to make it possible to do EBRT dose calculations with the use of Hounsfield units. It was assumed that the most exposed parts of all three OARs were exposed to the full EBRT dose of 45Gy/25fx. The dose was recalculated into equivalent dose in 2Gy fractions (EQD2) using α/β = 10Gy for tumour and α/β = 3Gy for normal tissue. Dose volume constraints for accumulated dose (whole pelvic EBRT + boost) to the organs at risk were D2cc <90 Gy EQD2 for the bladder, and D2cc <75 Gy EQD2 for rectum and sigmoid.
Four different boost techniques were evaluated and compared: 1) intracavitary brachytherapy (IC), 2) intracavitary/interstitial brachytherapy (IC/IS-BT), 3) intracavitary brachytherapy combined with IMRT (IC-BT + IMRT) and 4) IMRT.
Intracavitary BT boost (IC-BT)
Dwell positions in ring and tandem applicator was used. Manual 3D dose optimisation was performed with a standard loading pattern as a starting point Citation[1].
Intracavitary/interstitial BT boost (IC/IS-BT)
Dwell positions in ring, tandem and needles were used. Manual 3D dose optimisation was performed with a standard loading pattern as a starting point Citation[1]. Dwell times in needles were kept low so that the main dose contribution was delivered from tandem and ring.
Intracavitary BT combined with IMRT boost (IC-BT + IMRT)
In order to enable a precise intersection of IMRT and BT dose distributions it was assumed that the IMRT-patch-plan would be delivered in immediate continuation of the IC-BT with the applicator still in situ. It was assumed that the target moves together with the applicator, so that applicator guided IMRT would minimise set-up uncertainties to below 3 mm.
The IC-BT plan was 3D optimised for the best possible dose coverage of HR-CTV, while not going to the DVH limit for OARs in order to leave space for additional dose delivered to OAR by the IMRT part. shows an IC-BT plan optimised for combination with IMRT. The coloured region received at least prescribed dose, and covered the central region around the tandem of the HR-CTV (the red contour). In those regions where the IC-BT plan did not cover the HR-CTV with the prescribed dose, a PTV margin of 3 mm was added to the HR-CTV to take into account setup uncertainties for an IMRT-patch-plan (PTV is indicated as the blue contour on ). The IMRT-patch-plan was a 7-field IMRT plan with field entrances different from the field entrances of the EBRT plan (whole pelvis) in order to avoid a highway of dose through the patient. The IMRT-patch-plan was optimised on top of the IC-BT plan (Eclipse 7.5.51, pencilbeam, Aria 8.0) by using an inverse dose optimisation tool which allows using DVH constraints on the total dose of IC-BT and IMRT. shows the dose contribution from the IMRT-patch-plan, which gives dose to the part of the target, which was not covered by the IC-BT plan.
IMRT boost
The IMRT boost was assumed to be applicator guided like the IMRT-patch-plan as described above. A 7-field IMRT plan with field entrances different from the field entrances in the EBRT plan (whole pelvis) was used. PTV margin of 3 mm around the HR-CTV was added to take into account setup uncertainties. The central part of the target was dose escalated to mimic the dose inhomogeneity in BT (). A volume with 1cm inner margin to HR-CTV was optimised to 150% of prescribed dose (roughly equivalent to a BT dose distribution) which is equivalent to 115 Gy EQD2 in total. Further dose escalation towards the centre was not performed since it was assumed that doses larger than 115 Gy would not result in increased local control. No upper dose limit to HR-CTV was used when optimising the boost plan.
Dose parameters
For the target the D90, D100 and V100% was evaluated. For IC-BT boost and IC/IS-BT boost D90, D100 and V100% was evaluated in HR-CTV. In boost plans with IMRT, the D90, D100 and V100% was evaluated in PTV. V60Gy defines the total volume irradiated to more than 60 EQD2 Gy (normal tissue) by the accumulated dose from EBRT (whole pelvis) and boost. V60Gy was assessed in cumulated dose plans in which elective EBRT was added to boost dose. The boost plans were renormalized to EQD2 doses (α/β = 3Gy) at the physical isodose level of 3.3 Gy, since 45Gy/25fx plus 4×3.3Gy corresponds to 60Gy in EQD2 dose.
A t-test was used to perform pair-wise comparison between the IC/IS-BT boost and the other boost modalities: IC, IC + IMRT and IMRT alone.
Finally, it was investigated whether hot spots or cold spots would appear in the target region in combined BT-IMRT plans. The dosimetric impact of displacing the IMRT-patch-plan by 3mm towards or away from the high dose area in the IC-BT plan was assessed by evaluating dose profiles across the intersection of BT and IMRT dose distribution.
Results
The target coverage was always limited by the DVH constraints for OARs except for one IMRT dose plan where full target coverage was reached without reaching the DVH constraints for any OARs. It was technically feasible to combine BT and IMRT by optimising the IMRT boost on top of the IC-BT dose distribution. An example of a combined dose distribution is shown in .
Figure 4. Cumulated dose from IC-BT and IMRT-patch-plan. The coloured area receives at least the prescribed dose (7Gy). The red contour is HR-CTV.
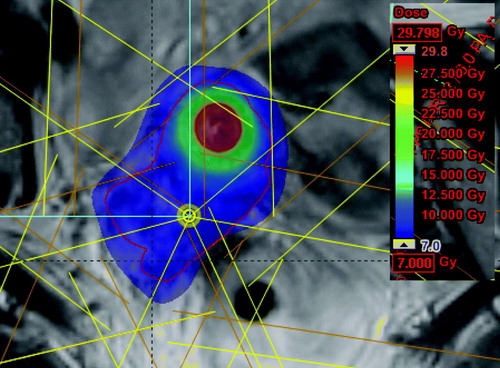
Dose and volume parameters for the four boost modalities are shown in . The target coverage, D90 and D100 were significantly improved by using IC/IS-BT, IC-BT + IMRT or IMRT instead of IC-BT. D100 was in 5/6 cases higher for IC-BT + IMRT and IMRT boost than for IC/IS-BT boost. D90 was in 4/6 cases higher for IMRT than for IC/IS-BT. In 4/6 cases D90 was higher for IC/IS-BT than for IC-BT + IMRT.
Table I. Tumour coverage, tumour dose, volume irradiated to more than 60Gy (V60Gy), and dose to OARs. The median, minimum and maximum values are listed for each parameter. P-values correspond to pair-wise comparison to IC/IS-BT as a reference.
V60Gy was almost the same for IC-BT and IC/IS-BT, but it was higher for IC-BT + IMRT. For IMRT alone V60Gy was substantially higher than in IC/IS-BT. V60Gy was in this case increased by a median factor of 2.0 [1.4–2.3]. The extension of the 60Gy volume is shown for IMRT alone and IC/IS in .
Figure 5. The coloured area is the volume receiving more than 60Gy EQD2 when using A) IMRT boost, B) IC/IS-BT boost.
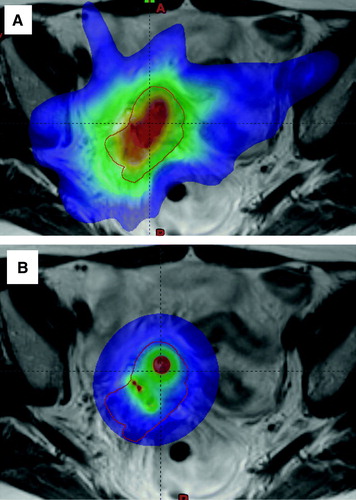
In IMRT boost plans the PTV often overlapped with OARs, and this limited the possibilities to obtain full target coverage. IMRT inverse optimisation often took more than one OAR to the dose limit in order to increase the target coverage as much as possible, and therefore IMRT induced an increase in the dose to the OARs as compared to BT although DVH constraints were respected with all boost techniques.
Displacing the IMRT-patch-plan towards or away from the high dose BT area did not lead to hot or cold spots in the junction region between BT plan and IMRT plan. shows a dose profile from the target region-distance is measured relative to tandem. The IMRT-patch-plan contributes with dose from around 2 cm to the PTV edge which is situated at 4 cm from the tandem. Displacement of the IMRT patch-plan did not result in any cold spots with dose below 100%, and there were also not induced hot spots which were significant as compared to the dose inhomogeneity induced by BT.
Figure 6. Dose as function of distance from the tandem. Green curve show the dose when the IMRT-patch-plan is moved 3 mm towards hot BT area. Blue curve show the dose when the IMRT-patch-plan is moved 3 mm away from the hot BT area. Red curve show the original dose as function of distance from the tandem.
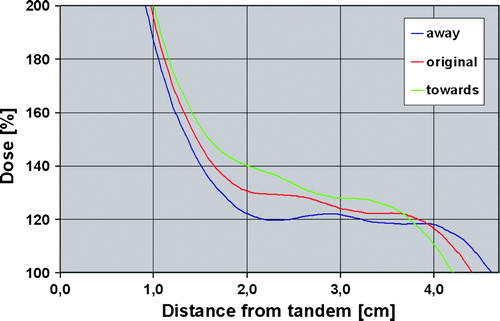
Discussion
The results from this dose planning study shows, that it was possible to improve the IC-BT dose distribution by adding dose from either interstitial needles or IMRT to the intracavitary dose distribution. The results also show that excluding IC-BT and using applicator guided IMRT boost instead will result in a substantially larger volume irradiated to more than 60 Gy. The clinical impact of increased V60Gy volume is not known in detail, but prior studies have pointed out, that large volume in the pelvic region receiving more than 60Gy correlate to side-effects Citation[13]. Due to this fact we would recommend to keep IC-BT in the boost in locally advanced cervical cancer.
During this dose planning study it became clear, that it depends on the individual tumour topography whether IC/IS-BT or IC-BT + IMRT boost was the most favourable technique. For very asymmetric tumours it can be difficult to get good tumour coverage with IC/IS-BT because of the challenge to place needles in an optimal configuration as in the case shown in . Especially the minimum target dose was sensitive to the geometry of the implant in IC/IS-BT, and in such cases IC-BT + IMRT may be useful to improve tumour coverage. On the other hand, it was difficult to improve the target coverage with a combination of IC-BT and IMRT when the tumour was in close relation to OARs (). In this case the PTV volume overlapped with OARs and it was necessary to compromise the dose coverage of the target. For such tumour topography IC/IS-BT was superior.
Figure 7. Dose distribution from IC-BT in a patient. The PTV volume (blue contour) for IMRT-patch-plan extends into OARs, and the patient would be a good candidate for IC/IS-BT.
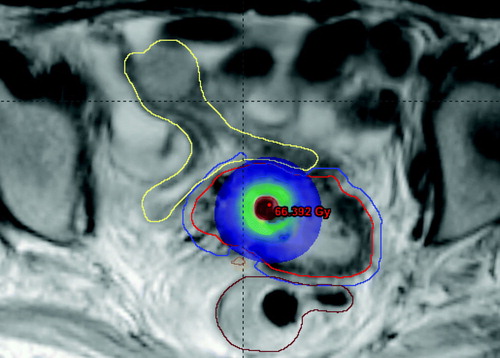
A limitation of the study is that the setup uncertainty for IMRT is not taken into account for the OAR. With IMRT the uncertainties on delivered dose to OAR is larger than for BT due to set-up errors. This means that the OAR DVH constraints obtained from clinical experience in BT may not be directly transferable to IMRT. One could argue that harder OAR constraints should be used with IMRT. In addition it could be relevant to evaluate other OAR DVH constraints than D2cc in EBRT dose distributions.
Our results are in overall agreement with the dose planning study of Georg et al. Citation[14], Citation[15] where comparison between IC-IS-BT and IMRT was performed. They concluded that IC/IS-BT was most often superior to IMRT dose plans, and that IMRT resulted in a considerable increase in V60Gy volume. The mean V60Gy volume found in our study was around 130 cc smaller (17%) than the V3Gy boost dose volume (corresponding to around 58Gy EQD2) assessed in the study of Georg et al. This may be due to the fact that we evaluated the V60Gy volume in cumulative dose plans. Part of the V3.3Gy boost volume is located outside the 45Gy EBRT dose plateau, and therefore V3.3Gy (in boost) will be larger than V60Gy (cumulative).
Several prior studies have concluded that IMRT is an alternative to BT Citation[16–19]. In general, these studies do not apply image guided and optimised brachytherapy, and the conclusions cannot be extrapolated directly into a setting with modern 3D image guided brachytherapy. Another important issue, which has often not been taken into account, is that EBRT and BT are fundamentally different with regard to need of margins that take set-up uncertainties into account. Geometric uncertainties are much smaller in BT than in EBRT, and any study that compares BT and EBRT should take into account that the dose should be delivered to a larger volume with EBRT (CTV + margins) than with BT (CTV). Finally, great care should be shown with drawing conclusions from comparing homogeneous EBRT dose distributions to inhomogeneous BT dose distributions. Even with the same coverage and the same dose to the outer part of the tumour, the dose in the central part of the tumour may be very different with EBRT and BT dose distributions. In our study we tried to compensate for this by applying a dose escalation to the central part of the target with IMRT, but at the moment there is no evidence telling us to which dose level this escalation should be performed in order to obtain the same level of local control.
Conclusion
In this study it was shown that it is technically possible to create robust dose plans that combines image guided IC-BT and applicator guided IMRT. Combination of IC-BT with either interstitial brachytherapy or IMRT-patch-plan provided dosimetrically options for boost, and the dose coverage for large and/or topographically unfavourable cervical tumours could be significantly increased when compared to IC-BT alone. It depended on the patient anatomy which of the two techniques was the most favourable. Image guided IMRT alone with a tight margin of 3 mm significantly increased the volume irradiated to more than 60Gy, and therefore IMRT alone cannot be advocated as a replacement of BT for boost in cervical cancer. Declaration of interest: The authors report no conflicts of interest. The authors alone are responsible for the content and writing of the paper.
References
- Lindegaard JC, Tanderup K, Nielsen SK, Haack S, Gelineck J. MRI-guided 3D optimization significantly improves DVH parameters of pulsed-dose-rate brachytherapy in locally advanced cervical cancer. Int J Radiat Oncol Biol Phys 2008.
- De Brabandere M, Mousa AG, Nulens A, Swinnen A, Van Limbergen E. Potential of dose optimisation in MRI-based PDR brachytherapy of cervix carcinoma. Radiother Oncol 2007.
- Haie-Meder C, Pötter R, Van Limbergen E, et al. Recommendations from Gynaecological (GYN) GEC-ESTRO Working Group (I): Concepts and terms in 3D image based 3D treatment planning in cervix cancer brachytherapy with emphasis on MRI assessment of GTV and CTV. Radiother Oncol 2005; 74: 235–45
- Pötter R, Haie-Meder C, Van LE, et al. Recommendations from gynaecological (GYN) GEC ESTRO working group (II): Concepts and terms in 3D image-based treatment planning in cervix cancer brachytherapy-3D dose volume parameters and aspects of 3D image-based anatomy, radiation physics, radiobiology. Radiother Oncol 2006; 78: 67–77
- Nag S, Cardenes H, Chang S, et al. Proposed guidelines for image-based intracavitary brachytherapy for cervical carcinoma: Report from Image-Guided Brachytherapy Working Group. Int J Radiat Oncol Biol Phys 2004; 60: 1160–72
- Pötter R, Dimopoulos J, Georg P, et al. Clinical impact of MRI assisted dose volume adaptation and dose escalation in brachytherapy of locally advanced cervix cancer. Radiother Oncol 2007; 83: 148–55
- Viswanathan AN, Dimopoulos J, Kirisits C, Berger D, Pötter R. Computed tomography versus magnetic resonance imaging-based contouring in cervical cancer brachytherapy: Results of a prospective trial and preliminary guidelines for standardized contours. Int J Radiat Oncol Biol Phys 2007; 68: 491–8
- Hatano K, Sekiya Y, Araki H, et al. Evaluation of the therapeutic effect of radiotherapy on cervical cancer using magnetic resonance imaging. Int J Radiat Oncol Biol Phys 1999; 45: 639–44
- van de Bunt L, van der Heide UA, Ketelaars M, de Kort GA, Jurgenliemk-Schulz IM. Conventional, conformal, and intensity-modulated radiation therapy treatment planning of external beam radiotherapy for cervical cancer: The impact of tumor regression. Int J Radiat Oncol Biol Phys 2006; 64: 189–96
- Dimopoulos JC, Kirisits C, Petric P, et al. The Vienna applicator for combined intracavitary and interstitial brachytherapy of cervical cancer: Clinical feasibility and preliminary results. Int J Radiat Oncol Biol Phys 2006; 66: 83–90
- Kirisits C, Lang S, Dimopoulos J, Berger D, Georg D, Pötter R. The Vienna applicator for combined intracavitary and interstitial brachytherapy of cervical cancer: Design, application, treatment planning, and dosimetric results. Int J Radiat Oncol Biol Phys 2006; 65: 624–30
- Taylor A, Rockall AG, Reznek RH, Powell ME. Mapping pelvic lymph nodes: Guidelines for delineation in intensity-modulated radiotherapy. Int J Radiat Oncol Biol Phys 2005; 63: 1604–12
- Barillot I, Horiot JC, Maingon P, et al. Impact on treatment outcome and late effects of customized treatment planning in cervix carcinomas: Baseline results to compare new strategies. Int J Radiat Oncol Biol Phys 2000; 48: 189–200
- Georg D, Kirisits C, Hillbrand M, Dimopoulos J, Pötter R. Preliminary results of a comparison between high-tech external beam and high-tech brachytherapy for cervix carcinoma. Strahlenther Onkol 2007; 183: 19–20
- Georg D, Kirisits C, Hillbrand M, Dimopoulos J, Pötter R. Image-guided radiotherapy for cervix cancer: High-tech external beam therapy versus high-tech brachytherapy. Int J Radiat Oncol Biol Phys 2008.
- Molla M, Escude L, Nouet P, et al. Fractionated stereotactic radiotherapy boost for gynecologic tumors: An alternative to brachytherapy?. Int J Radiat Oncol Biol Phys 2005; 62: 118–24
- Low DA, Grigsby PW, Dempsey JF, et al. Applicator-guided intensity-modulated radiation therapy. Int J Radiat Oncol Biol Phys 2002; 52: 1400–6
- Aydogan B, Mundt AJ, Smith BD, et al. A dosimetric analysis of intensity-modulated radiation therapy (IMRT) as an alternative to adjuvant high-dose-rate (HDR) brachytherapy in early endometrial cancer patients. Int J Radiat Oncol Biol Phys 2006; 65: 266–73
- Guerrero M, Li XA, Ma L, Linder J, Deyoung C, Erickson B. Simultaneous integrated intensity-modulated radiotherapy boost for locally advanced gynecological cancer: Radiobiological and dosimetric considerations. Int J Radiat Oncol Biol Phys 2005; 62: 933–9