Abstract
Purpose. Recently, Varian Medical Systems have announced the introduction of a new treatment technique, in which dose is delivered over a single gantry rotation with variable MLC positions, dose rate and gantry speed. In February 2008, a preclinical installation of the RapidArc™ beam delivery approach was carried out on a Varian Clinac at Rigshospitalet in Copenhagen. The purpose of the installation was to perform measurements to verify the correctness of doses delivered with the RapidArc technique. In May 2008, the clinical release of the RapidArc application was installed at Rigshospitalet. Methods and materials. Nine treatment plans were generated in the Eclipse version 8.5 including the RapidArc optimizer for H&N and prostate cases. The plans were delivered to the Scandidos Delta4® cylindrical diode array phantom. First, the measured dose distributions were compared with the calculated doses. All plans were then delivered several times to verify consistency of the delivery. Gamma analysis was used to verify the correspondence between dose distributions. The temporal resolution of the delivery was analysed by investigating the arc segments between control points separately. Results. Overall, good agreement was observed between measured and calculated doses in most cases with gamma values above 1 in >95% of measured points. The reproducibility of delivery was also very high. Gamma analysis between two consecutive runs of the same delivery plan generally showed gamma values above 1 in none of the measured points, and dose deviation less than 1%. Temporal analysis showed small discrepancies between doses delivered between control points (∼2 degrees of the rotation) in consecutive runs of a plan, however these were cancelled out in the accumulated dose. Conclusion. The delivery of RapidArc beam delivery has been verified to correspond well with calculated dose distributions for a number of different cases. The delivery was very reproducible, and was carried out with high stability of the accelerator performance.
Radiotherapy beam delivery in arcs has the potential of delivering a highly conformal dose to the target while spreading the dose to the surrounding tissue and thereby sparing organs at risk form receiving high radiation doses.
The recent years have seen an increasing interest in arc delivery techniques, especially with the introduction of the helical treatment delivery concept by Tomotherapy Citation[4], Citation[5]. This spring, Varian Medical Systems, Inc., has introduced a new arc delivery technique termed RapidArc™. In this approach, the treatment is delivered in a single arc rotation of the linac gantry, during which the MLCs are moving dynamically while the dose rate and the gantry speed are also varied throughout the rotation of the gantry Citation[7]. This concept differs from other arc therapy techniques in several ways. Firstly, it is delivered in a single gantry rotation in a “cone-beam” fashion, as opposed to the helical technique which is delivered in a “fan-beam” fashion. Also, the general IMAT concept as described in Citation[9] makes use of several arcs, all delivered in a cone-beam fashion, but as an “arc-in-arc” approach where each arc patches in the missing dose levels from the previous arcs to obtain the desired dose distribution. With the RapidArc technique, a sufficient number of degrees of freedom to obtain a highly conformal dose distribution is obtained in a single arc delivery through the combination of varying dose rate and gantry speed combined with dynamic MLCs, all specified in a large number of control points over the rotation. The limiting factor is basically the amount of MLC movement allowable per second, and thereby per degree of gantry rotation, which effectively sets a limit to the modulation factor.
Whenever a new technique is introduced into clinical practice, extensive dosimetric verification is required to ensure correct treatment delivery. This encompasses both general machine performance and quality assurance on the patient specific level. The RapidArc approach can be considered an extension to dynamic MLC (DMLC) IMRT, and the machine commissioning and QA should validate standard DMLC delivery and the combination with dose rate variations and gantry rotation with variable speed which is specific to RapidArc. A recent paper describes a programme for RapidArc commissioning and QA using designed delivery plans to test accuracy in DMLC position, precise dose rate control during gantry rotation, and accurate control of gantry speedCitation[2]. We here present the quality assurance programme and measurements at the patient specific level in the introduction of RapidArc into clinical practice at our institution.
Treatment plans for RapidArc delivery were inversely optimized in the Eclipse environment (Varian Medical Systems), version 8.5. This technique was released for clinical use in late April 2008, and installed at Rigshospitalet, Copenhagen University Hospital, in early May 2008. Treatment using the technique was started up for prostate cancer patients in early May 2008.
For the clinical startup, we chose to use two different dosimetric equipments for patient specific quality assurance based on our experiences with RapidArc dosimetry in the pre-clinical testing period. For each patient, measurements were performed using the Delta4® cylindrical diode array phantom from Scandidos, as well as ion chamber measurements in solid water.
In this study, we report the dosimetric verification measurements performed with the Delta4 phantom in connection with the quality assurance programme during the first period of RapidArc treatment at Rigshospitalet.
Methods and materials
In the Eclipse 8.5 environment, the arc optimizer was used to generate treatment plans for four head and neck test cases (patients 5–8), one prostate test case (patient 4), and three prostate patients (patients 1–3, two separate plans for one of them) for actual RapidArc treatment. The Eclipse system was configured using the beam data specific to the treatment machine, a Clinac 21iX, on which the plans were subsequently delivered. In the treatment planning system, the treatment couch (Exact couch) was modeled both for optimization and for dose calculation. Characteristics of the tested plans are shown in . The prostate plans had jaw openings of approximately 8–10 cm in each direction, and the head and neck plans had jaw openings of approximately 15 cm in each direction. For the prostate plans, homogeneous dose inside the PTV was sought, whereas in the head and neck cases, three prescription dose levels were specified in three embedded PTVs. For all plans, the prescribed fraction dose was 2 Gy (in the head and neck plans 2 Gy was prescribed per fraction to the highest dose level).
Table I. Description of measurement cases.
Verification plans were generated and recalculated on the CT-image of the Delta4 phantom for comparison between planned and measured doses.
In the treatment room, the Delta4 phantom was placed isocentrically and centrally on the treatment couch for the measurements, see . The Delta4 is a cylindrically shaped PMMA phantom, surrounding two crossing orthogonal planes with a total of 1069 p-Si diodes. The diodes are disc shaped with a volume of 0.04 mm3, with a centre to centre distance of 0.5 cm in the central area (6 cm×6 cm) and 1 cm in the outer area (up to 20 cm×20 cm) of the planes. Because of the phantom's cylindrical shape and orthogonal detector planes it is convenient for irradiation from 360° around the cylinder. An inclinometer attached to the accelerator gantry and connected to the Delta4 system was giving the instrument continuous, independent information about the gantry angle during the arc delivery. The RapidArc dose plans were exported from the Treatment Planning System (TPS) and imported into the Delta4 software (DICOM RT format), capable of reading the information about the MLC and gantry positions planned. For each measurement the Delta4 system was able to sort the dose information into sub-beam-structures, corresponding to the control points of the plan from the TPS. In the TPS, a RapidArc delivery plan is divided into 177 control points identifying MLC positions, dose rate and gantry speed for each control point. The sorting was made by associating the measured dose from each dose pulse from the accelerator with the actual gantry angle at the dose pulse delivery, measured by the inclinometer. The dose pulses measured during the gantry angle interval of a control point were summed together, giving the dose of that control point. All the treatment plans contained 177 control points, for which the measured dose distributions could be compared with a reference, step by step with a high temporal resolution.
Figure 1. The Delta4 phantom on the treatment couch. The red arrow indicates the inclinometer attached to the gantry and shown in large in the insert, which measures the gantry angle independently.
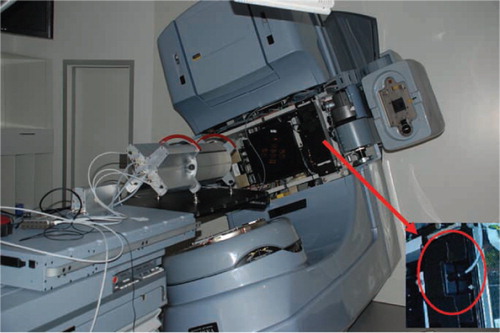
The Delta4 phantom is calibrated to absolute dose measurement using a farmer reference ion chamber for the specific linac, and can thus be used to measure absolute dose levels.
The test case plans were delivered one time on one occasion. The plans for the patients actually treated using the RapidArc technique were run 2–4 times consecutively on each day of treatment for the 4–6 days of treatment (so far). The correspondence between planned and measured doses was analyzed using gamma test, and the correspondence between consecutive deliveries of the same plan was likewise analyzed using gamma tests. The gamma evaluation criteria were 3% dose difference (relative to the maximum dose in the delivery), and 3 mm distance to agreement. The gamma analysis was performed within the analysis software following the Delta4 phantom.
Consecutive deliveries were compared control point by control point using the dose deviation, distance to agreement and gamma test tools in the software.
Results
In , an example is shown of the gamma evaluation of a delivered plan compared to the planned dose in the Delta4 software user interface.
Figure 2. A screenshot from the Delta4 software showing the result of delivery of a prostate case. In the upper panels are shown the absolute dose measurements in the two diode arrays with color coding denoting the dose. In the lower panel is shown three graphs; the left-most graph shows the dose deviations from the planned dose, the middle graph shows the distance to agreement from the planned dose, and the right-most graph shows the gamma index distribution.
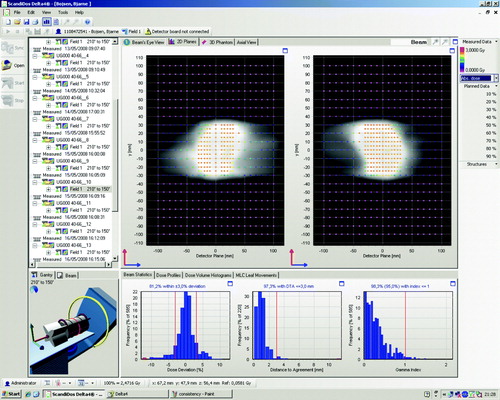
In , the fraction of passed gamma values for the delivered plans compared to the planned doses are shown for all plans. For all measurements, the fraction of passed gamma values is above 95%, and the mean fraction over all measurements is 98.5%. The maximum gamma value is between 2.56 in the worst case and 0.75 in the best case, and the mean of the maximum gamma values for all measurements is 1.33. Gamma values above 2 did only occur in two of the H&N cancer test cases, which were highly modulated and covering a larger volume compared to the rest of the plans.
Table II. Fractions of measurement points passing the gamma test compared to planned doses for all the deliveries. Gray indicates that no measurement was performed on the day.
Comparing consecutive deliveries of the same plan, there is very good agreement in all cases for the accumulated dose. For immediate re-delivery, all gamma values are below 1 in all cases. In a, an example is shown of the gamma evaluation comparing two immediately consecutive runs of the same plan in the Delta4 software environment. The dose deviations are below 1% in all measurement points in all cases.
Figure 3. Two screenshots from the Delta4 software showing consecutive deliveries of the same plan as illustrated in . a) shows a comparison between two immediately consecutive deliveries of the same plan, b) shows a comparison between deliveries from two different days.
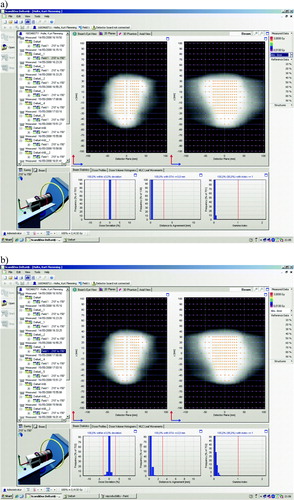
For the plans re-delivered on several days, there is also very good agreement from day to day. The gamma values are again below 1 in all points in all cases. There are slightly higher dose deviations and especially higher distances to agreement than for the immediate re-deliveries, which likely stem from the repositioning uncertainty of the Delta4 phantom from day to day, see b.
A comparison was done of the sub-arc reproducibility of delivery as per control point in one case (for the subarc analysis, the gamma dose criterium 3% ∼ <1–2 mGy, which means that deviations are on the same order as the measurement resolution). Gamma evaluation for each control point between two consecutive deliveries of this case showed large deviations on the control point level, although there were no deviations for the accumulated dose. For 45% of the sub-arcs, the fractions of measurement points passing the gamma test (compared to a previous delivery) was below 95%, and only for 37% of the sub-arcs was the statistic as good as for the accumulated dose (100% pass fraction between consecutive deliveries). The distance to agreement was not showing large deviations, but the effect was in the dose deviations (absolute magnitudes on the order of milligray within each sub-arc). Typically, sub-arcs with lower measured doses than in the previous delivery were immediately followed by sub-arcs with higher measured doses. This is consistent with a noise pattern stemming from small variations in the delivery pattern (single pulses “jumping” between the sub-arc rotations as measured by the independent inclinometer). This is not surprising since the accelerator is running with tolerance levels on all performance, and small variations within the tolerances are expected.
Discussion
We have demonstrated high consistency between planned doses as optimized and calculated in the Eclipse version 8.5, and corresponding doses delivered by the linac, for the RapidArc treatment application. The repeated delivery of planned doses was also investigated, and exhibited very high reproducibility both for immediate re-delivery and for re-delivery over several days. The discrepancies at the control point level between re-deliveries can have several reasons. For one, the reading of the gantry angle from the independent inclinometer may be a source of error, if it is not very stable. Also, the accelerator is running within finite tolerances on all parameters, and therefore each delivery may not be exactly identical.
In our quality assurance programme for the clinical implementation of RapidArc, the reported Delta4 measurements have been supplemented with ionization chamber measurements in a solid water phantom (white water RW-3, PTW) in 2–3 points for each treatment plan. Patient specific quality assurance has been performed on a daily basis using the Delta4 phantom and occasionally ionization chamber measurements. Furthermore, daily output control is performed each morning.
A machine performance commissioning programme has been run on the accelerator (picket fence type) using the EPID for imaging. This programme is intended to verify the correct interplay of gantry rotation with the leaf sequence, and of combinations of dose rate, gantry speed and MLC speed in the RapidArc mode, using designed RapidArc delivery plansCitation[2].
The programme is an extension of and addition to the general verification required to validate the correct running of the intricate delivery patterns resulting from the TPS optimization process for dynamic treatment delivery techniques. For DMLC IMRT delivery, a variety of designed test plans to validate machine performance has been suggested and are commonly used for regular machine QA Citation[8], Citation[3], Citation[1]. These test plans are intended to verify the interplay of MLC positions and delivery of correct amount of monitor units during dynamic treatment.
In addition to the machine specific QA, many clinics perform patient specific QA for all IMRT delivery courses. This is most often done by delivering the treatment plan to a solid water phantom with embedded 2D dosimeter array or films, and comparing the measured dose to the dose calculated for the patient plan as delivered to the phantom. Another method for patient specific QA is portal dosimetry, employed much in the same way but with measurement performed for the transmitted dose through the phantom using an electronic portal imagerCitation[6]. Extensive patient specific QA is often employed in the start-up process of implementing new dynamic delivery techniques, while being phased out when consistent delivery is continuously observed and replaced by regular machine specific QA and only occasional patient specific QA. This has been the process at our institution for DMLC IMRT, which we have used as a routine treatment technique for more than 7 years.
For RapidArc treatment, we now perform patient specific QA using the Delta4 phantom before start of the treatment course, and on at least 3 consecutive treatment days in the beginning of the treatment. This large amount of quality assurance is a safety precaution deemed appropriate by us as this is one of the first clinical installations of the technique. As we continue to observe consistent dosimetric accordance between planned and measured doses and between consecutive measurements, the amount of quality assurance applied for RapidArc treatments will be scaled down.
In 2007, a European council consisting of five clinics has been established to collaborate with Varian for the testing and development of the RapidArc treatment plan optimizer and for testing of the beam delivery application. Rigshospitalet is part of this council, and has been involved in RapidArc testing since fall 2007. In February 2008, a preclinical installation of the RapidArc capability was carried out at Rigshospitalet for dosimetric verification purposes and for testing of the delivery technique in a clinical environment. During the preclinical installation of the RapidArc application in February 2008 at Rigshospitalet, measurements were performed using a wide variety of dosimetric equipment, including the linac EPID, radiochromic film, gels, and ionization chamber arrays for arc dosimetry from PTW (seven 29 with OCTAVIUS) and IBA Dosimetry (I'mRT MatriXX with MULTICube). All of these modalitites generally exhibited good agreement between planned and measured doses as quantified by gamma evaluation in accordance with our observations in the present study.
Presently (mid June 2008), eight patients are being treated with the RapidArc technique at our clinic. All eight are prostate cancer patients, but plans are to expand with further cancer sites, exploring possibilities for cervix cancer, anal canal cancer, head and neck cancer and brain metastases. Treatment planning for all of these cancer sites have been investigated in our own and other groups in the Varian initiated European “RapidArc Council” (see below in acknowledgements).
For starting up the RapidArc technique in clinical practice at Rigshospitalet, we have performed a study to compare five-field IMRT (which is our standard technique for prostate radiotherapy) plans with RapidArc plans for prostate cancer. This study showed that the RapidArc treatment technique can be used advantageously in the radiation treatment of prostate cancer patients. The RapidArc treatment plans gave better sparing of organs at risk, in most cases smaller amount of monitor units, and good target homogeneity (see paper by Kjær-Kristoffersen et al. in this issue of Acta Oncologica).
Conclusion
Delivery of RapidArc treatment plans is consistent with the dose calculated in the treatment planning system, Eclipse 8.5. This has been verified using the Delta4 phantom (Scandidos, Sweden). Also, there is high reproducibility of consecutive delivery within the same day and from day to day.
Acknowledgements
The authors wish to thank Varian Medical Systems for the fruitful collaboration in the RapidArc testing process, as well as the other clinics (the European “RapidArc council”) involved in the testing period – VU Medical center Amsterdam, CRLC Val d'Aurelle Montpellier, University Hospital Zürich, and Oncology Institute of Southern Switzerland Bellinzona.
We are also grateful to Scandidos for loan of the Delta4 phantom, and specifically to Ingemar Wiberg and Görgen Nilsson for assisting us in setup and measurements using the phantom.
References
- Ma L, Yu CX, Earl M, Holmes T, Sarfaraz M, Li XA, et al. Optimized intensity-modulated arc therapy for prostate cancer treatment. Int J Cancer 2001; 96: 379–84
- Mackie TR, Balog J, Ruchala K, Shepard D, Aldridge S, Fitchard E, et al. Tomotherapy. Semin Radiat Oncol 1999; 9: 108–17
- Otto K. Volumetric modulated arc therapy: IMRT in a single gantry arc. Med Phys 2008; 35: 310–7
- Yu CX. Intensity-modulated arc therapy with dynamic multileaf collimation: An alternative to tomotherapy. Phys Med Biol 1995; 40: 1435–49
- Ling C, Zhang P, Archambault Y, Bocanek J, Tang G, LoSasso T. Commissioning and quality assurance of RapidArc radiotherapy delivery system. Int J Radiat Oncol Biol Phys 2008; in press.
- van Esch A, Bohsung J, Sorvari P, Tenhunen M, Paiusco M, Iori M, et al. Acceptance tests and quality control (QC) procedures for the clinical implementation of intensity modulated radiotherapy (IMRT) using inverse planning and the sliding window technique: Experience from five radiotherapy departments. Radiother Oncol 2002; 65: 53–70
- LoSasso T, Chui CS, Ling CC. Comprehensive quality assurance for the delivery of intensity modulated radiotherapy with a multileaf collimator used in the dynamic mode. Med Phys 2001; 28: 2209–19
- Essers M, de Langen M, Dirkx ML, Heijmen BJ. Commissioning of a commercially available system for intensity-modulated radiotherapy dose delivery with dynamic multileaf collimation. Radiother Oncol 2001; 60: 215–24
- McDermott LN, Wendling M, van Asselen B, Stroom J, Sonke JJ, van Herk M, et al. Clinical experience with EPID dosimetry for prostate IMRT pre-treatment dose verification. Med Phys 2006; 33: 3921–30