Abstract
Background: 177Lu-octreotate is an effective treatment modality for patients with metastatic neuroendocrine tumors. The kidney is a critical dose-limiting organ in that modality. We investigated the absorbed doses in the kidney and compared whole kidney volume (WKV) and small (4 cm3) volume of the kidney (SV) methods. We also evaluated a new calculation method that was based on two single photon emission computed tomography/computed tomography (SPECT/CT) scans.
Methods: Absorbed radiation doses in the kidneys were calculated for 24 patients with neuroendocrine tumors. All patients received four cycles of 177Lu-octreotate given at eight-week intervals with a mean activity of 7.1 GBq (range 3.28–8.79 GBq). Absorbed doses and half-lives were calculated by the WKV and SV methods. Dosimetry was determined for the cortex and medulla in the first treatment cycle.
Results: The mean absorbed radiation dose was 0.44 ± 0.15 Gy/GBq for the WKV method and, 0.74 ± 0.28 Gy/GBq for the SV method. Three patients had a 20% increase of the absorbed dose over the four treatment cycles for the WKV method compared to eight patients for the SV method. The mean absorbed dose in the medulla was 0.62 ± 0.27 Gy/GBq, whereas the mean absorbed dose in the cortex was 0.41 ± 0.22 Gy/GBq. Both regions had similar half-lives. Patients who received lower activities for medical reasons still had similar absorbed doses to kidneys compared to those who received the full activities. Our study indicates that absorbed doses can be calculated reliably using two SPECT/CT scans, at 24 and 168 hours after each treatment.
Conclusions: Absorbed doses in the kidneys from systemic radionuclide therapy that are measured by the WKV method and SV method cannot be directly compared. There were regional differences within kidneys for the uptake of 177Lu-octreotate. Two SPECT/CTs are sufficient for kidney dosimetry based on our new calculation method.
Introduction
177Lutetium DOTA-octreotate (177Lu-octreotate) is used in treating locally advanced and metastatic neuroendocrine tumors (NET). This treatment modality has been in clinical use since 2001 [Citation1]. The main indication is locally advanced or metastatic gastroenteropancreatic NET overexpressing somatostatin receptors upon imaging for somatostatin receptors [Citation2,Citation3]. The activity range of 177Lu-octreotate usually given is 5.5–7.4 GBq at eight-week intervals [Citation4–7]. It is common to give four treatments with the possibility of retreatment later on in progression. Progression-free survival of three years is often obtained and the disease control rate (partial response and stable disease) ranges between 60% and 80% [Citation8,Citation9]. The first results from the randomized study that compared 177Lu-octreotate with octreotide LAR 60 mg in patients with inoperable, progressive ileal NET were presented at the European Cancer Congress in 2015 [Citation10]. The data indicated that 177Lu-octreotate treatment significantly improved progression-free survival in patients with mid-gut NETs.
In addition to bone marrow, the kidney is a critical dose-limiting organ for 177Lu treatment. Renal irradiation occurs as a consequence of the tubular reabsorption of filtered radiopeptides, which causes damage to the glomeruli [Citation11]. Amino acid solutions are co-administered to decrease the re-uptake of radiopeptides and thus reduce the incidence of kidney toxicity. Some toxicity still occurs, although it occurs less often for 177Lu-octreotate treatment than for 90Yttrium peptide receptor radionuclide treatments. A study of a series of 290 patients that compared 90Y-DOTANOC with 177Lu-octreotate found that 24% of patients given 177Lu-octreotate treatment had grade 1 and 1.7% had grade 2 toxicity [Citation12]. Moreover, as many as 43.9% of the patients had nephrotoxicity after 90Y PRRT treatment. However, not every patient in that study had received protective amino acid solution. The long latency of late kidney injury, even after 19 years [Citation13], makes the accurate and precise modeling of kidney radiation damage difficult to accomplish.
177Lu emits beta radiation with a maximum energy of 0.5 MeV and a maximal tissue penetration of 2 mm. 177Lutetium emits low-energy gamma radiation at 113 keV (6.4%) and at 208 keV (11.0%). Planar imaging and single photon emission computed tomography/computed tomography (SPECT/CT) are used for post-treatment diagnostics and quantification of dosimetry for subsequent treatment [Citation8,Citation14,Citation15]. The tolerance levels used for exposure to PRRT were first obtained from data of the exposure to external radiation [Citation16]. Those results, however, might not be directly applicable to systemic radionuclide therapy because of the remarkably lower dose rates and non-uniformity of the absorbed dose distribution used in PRRT.
A considerable number of different quantification methods have been used to calculate the absorbed radiation doses in the kidneys. For example, Garkavij et al. [Citation8] used three different methods to evaluate the absorbed kidney dose, namely: planar scintigraphy images, SPECT data and quantitative SPECT images. Other researchers, Sandström et al. [Citation14] and Garske et al. [Citation17,Citation18] used the small volume of interest analysis (VOI) and also the planar scintigraphy method, whereas Larsson et al. [Citation6] used a conjugate view method with planar scintigraphy.
We calculated, evaluated and compared the absorbed kidney doses for two methods, which were based on SPECT/CT, namely: whole kidney volume (WKV) and small volume (SV) methods. The patients had received four successive courses of 177Lu-octreotate given at eight-week intervals. The differences in absorption of doses between the two methods, between patients and over time were all recorded. We also evaluated the numbers and timing of SPECT/CT scans needed to indicate whether the commonly used three scans protocol could safely be reduced to two or even to one while retaining sufficient accuracy.
Materials and methods
Patients
Twenty-four consecutive patients (15 male, nine female) with metastatic NETs, WHO grade 1–2, were treated with 177Lu-octreotate in the Department of Radiotherapy, Nuclear Medicine, Cancer Center, Helsinki University Central Hospital, from February 2011 to August 2013. Their mean age was 63 years (range 41–77 years).
Treatment
The 24 patients who were studied had four treatments of 177Lu-octreotate at eight-week intervals. The intended activity administered per treatment cycle was 7.4 GBq, which amounted to a total activity of 29.6 GBq for the four successive treatments. The reasons for lowering the administered activity in the dosage were older age, previous chemotherapy, diabetes, hypertension, fragility or any combination of these. The activity was also reduced to 75% or even 50% if there were signs of bone marrow toxicity that had been sustained during earlier treatment cycles.
All patients received an eight-hour infusion of 2000 ml of commercially available amino acid solution (Vamin14 g N/l®) for kidney protection and antiemetics to counteract the associated nausea. The infusion of the amino acid solution began half an hour before the infusion of 177Lu-octreotate and continued for up to eight hours.
Kidney toxicity was estimated by serum creatinine levels that were taken at four time points according to CTCAE version 4.0 for two years after the first treatment. Hematological toxicity was also recorded.
The labeling of 177Lu was measured using TLC/Merck chromatography and impurities were measured by using ITLC/Varian chromatography for quality control before treatment. The labeling was 99% or higher for all the treatment cycles administered. The amount of free 177Lutetium was less than 0.2% and the impurities in the form of colloids and hydroxyls were less than 0.5%.
Imaging
All patients were scanned using Symbia T2 SPECT/CT at 24, 72 and 168 hours after treatment. SPECT/CT scans were taken using the step and shoot acquisition mode with 64 views in 360° gantry rotation with the matrix size of 128 × 128 and medium energy collimators. The collection time for one frame was 30 seconds. The energy peak for Lu-177 was set at 208 keV with a 20% widow level and with subwindows also set at 20%. The CT images were taken using 130 kV tube voltage and 3.0 mm slice thickness settings.
Tomographical reconstruction was based on an iterative calculation method with scatter, attenuation and Flash 3D corrections. Scatter corrections were made using lower and upper subwindows of the main window. Attenuation correction was based on CT images and the Flash 3D algorithm calculations for the higher peak of Lu-177 (208 keV). Corrected images were visually compared with uncorrected images for quality assurance purposes.
Determination of the absolute activity within patients
Calibration of the imaging system for absolute activity distribution (Bq/counts/voxel) was performed before patient imaging commenced, and it was followed regularly. The standard activity measurements were calibrated on the anthropomorphic Alderson phantom using the identical imaging protocol for both SPECT and CT that are currently being used in most patient studies.
The standard cylindrical source (60 MBq, height 6.9 cm, diameter 3.4 cm, volume 62 cm3) was implanted inside the abdominal section of the Alderson phantom during the calibration. The SPECT images of the phantom were reconstructed and compensated for scatter, collimator-detector response (Flash3D, which is known also as resolution recovery) and attenuation. The source boundaries were delineated based on CT images. The total number of counts from the corrected SPECT image was divided by the known activity value to form the calibration factor (Bq/count/voxel). Multiplying by the calibration factor, the counts from SV and WKV VOIs were converted to activities.
Dosimetric calculation
Left and right kidney volumes (cm3) were determined separately from each of the three SPECT/CT scans from the transversal CT-slices by using VOI-analysis. A SV sample (4 cm3) of the maximal activity region of the lateral kidney cortex and outer medulla was selected. The kidney pelvis was avoided in this selection. The maximum uptake volume varied only little, but it did not always occur in the same place. The detected counts from the SV samples were then scaled up to represent the counts for the whole kidney for each of the four treatment cycles when the WKV was known. The SV method thus represents the absorbed dose value for a SV and the estimated maximal absorbed dose for the whole kidney. The counts obtained from the subregions of the cortex and medulla were also determined (4 cm3) from transverse slices in cycle 1 as described by Sandström et al. [Citation14]. However, we also used a new derived EquationEquation (1)(1) and two points SPECT/CT scans at 24 and 168 hours after each treatment to determine the absorbed doses in the cortex and the medulla.
The kidney activities Ak(t) in MBq units were calculated at three time points after infusion (t = 24, 72 and 168 hours) and used as input data into the OLINDA program. When the kidney volume was determined, the OLINDA software converted the kidney activities into absorbed doses expressed as Gy units.
The doses were calculated per GBq and used to compare the absorbed doses of the four cycles for left and right kidneys. The SV method entails that a volume of 4 cm3 is individually sampled from the high activity region of the kidney for each treatment. The absorbed dose of the SV region was scaled up to the WKV to compare and determine the ratio between these two methods. The most reliable and convenient way to measure the absorbed dose was obtained by the OLINDA calculations for the time points 24, 72 or 168 hours.
Dose calculation based on three SPECT/CT scans is time consuming and requires that the patient returns to hospital twice. Using EquationEquations (1)(1) and Equation(2)
(2) below we were able to produce the necessary data for reliable and fast dosimetry using only two SPECT/CT scans taken at 24 and 168 hours after each treatment cycle.
The results obtained for the single time points or combinations of two time points (24 and 168 hours) were then compared against monoexponential kinetics that was assumed for the individual volumes of the kidneys and corrected. Kidney dose calculations were based on the following equations:
(1)
(2)
where k = 0.021 mGy*g/MBq*s is a scaling factor that converts the absorbed radiation doses into Gy units. The T½ term is the effective half-life (hours) and C24 is the kidney counts at 24 hours, C168 kidney counts at 168 hours after administration and Vk is the kidney volume (cm3) or mass (g), when the kidney density is 1.00 g/cm3. The scaling factor was the optimal fit of the linear EquationEquation 1
(1) to the absorbed doses. It takes into account the following: the sensitivity of the gamma camera, the reconstruction algorithm of the gamma camera including scattering, the attenuation corrections and the sensitivity of the activity determination by the calibrator. Those factors must remain sufficiently stable through the whole chain from activity measurements to image processing and therefore these require monitoring. Moreover, the absolute results must be corrected using standard sources of known activity.
Statistical methods
The absorbed kidney doses and kidney volumes were compared by the paired two-sided t-test. Differences between absorbed doses in different activities given were tested by the two-sided unpaired t-test. The dose calculations correlation coefficient R2 was tested in the comparisons to find a simpler way to image for optimum dosimetry. Absorbed kidney activities and kidney volumes were expressed as mean values with their standard deviations and ranges of each of the parameters. The effective half-lives were compared by a paired two-sided t-test.
Results
A total of 96 177Lu-octreotate treatments were given to 24 consecutive patients. The mean activity per cycle was 7.1 GBq (3.28–8.79 GBq) and the total activity 28.4 GBq (15.3–33.9 GBq). Seventeen patients received the intended activity of 7.4 GBq in all four courses. The activity administered to seven patients was reduced at least once in a total of 18 (19%) treatments.
None of the patients had kidney toxicity within the two-year follow-up after the start of the treatment as measured by serum creatinine levels. Twelve patients had grade 1 hematological toxicities.
The median volume of the right kidney was 165 cm3 (67–241 cm3) compared to 165 cm3 (103–269 cm3) for the left kidney. The median volumes of the kidneys were 181 cm3 (114–241 cm3) for men and 149 cm3 (67–236 cm3) for women.
There were no significant differences in the kidney volumes between cycles. However, the kidney volumes that were measured at the first and the fourth treatments lay within 10% of each other for only 12 of 24 patients (50%). One patient had an increase in size of more than 20% in the right side kidney and another patient had a similar increase on the left side. One patient had a left-sided decrease by more than 20%.
There were significant differences for the absorbed doses measured between the WKV and SV methods. The mean absorbed dose in the kidneys was 0.44 ± 0.15 Gy/GBq (range 0.22–1.05 Gy/GBq) for the WKV and 0.74 ± 0.28 Gy/GBq (range 0.36–1.92 Gy/GBq) for the SV method and thus was significantly (p < 0.001) different between the two methods. There was, however, a good correlation (R2=0.9031) between these two methods (). Individual results are presented in . Those patients who received the intended activity of 7.4 GBq had a mean absorbed dose of 0.43 ± 0.13 Gy/GBq (range 0.24–0.98 Gy/GBq) (). The seven patients who received lower activities still had equal absorbed doses to the patients who received the full activity, 0.49 ± 0.21 Gy/GBq (range 0.22–1.06 Gy/GBq), (p = 0.106). When the absorbed doses were measured by the SV method the mean absorbed doses for the first and fourth treatments were 0.72 ± 0.35 Gy/GBq (range 0.38–1.74 Gy/GBq) and 0.84 ± 0.37 Gy/GBq (range 0.37–1.91 Gy/GBq), respectively.
Figure 1. Correlations of the absorbed kidney doses (Gy/GBq) for the whole kidney and the small volume methods.
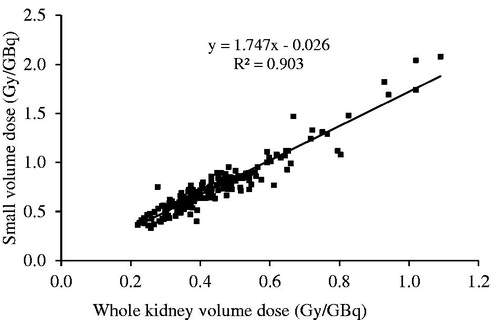
Figure 2. Absorbed doses (Gy/GBq) for patients who had received the intended activity of 7.4 GBq (78 treatments, mean 0.43 Gy/GBq black squares) compared to those who had lower activities (18 treatments, mean 0.49 Gy/GB black triangles) in their 177Lu-octreotate treatments. Method: whole kidney method.
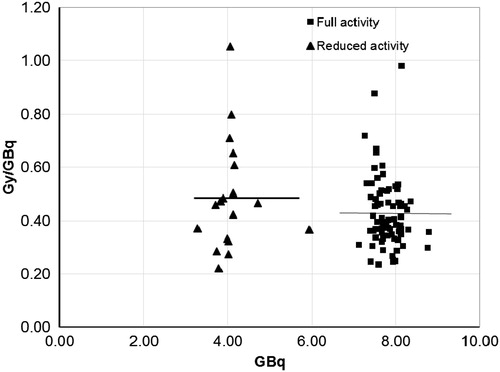
Table 1. Absorbed doses measured by the whole kidney and by the small volume methods and their ratios among the 24 consecutive patients who received four 177Lu-octreotate treatments at eight-week intervals.
The effective half-life of 177Lu-octreotate in kidney tissue of the 24 patients (averaged over the four cycles) were considered normally distributed for both methods according to the Shapiro-Wilk statistic. The mean of the half-life was 45.3 ± 5.9 hours (range 35.5–58.9 hours) in the case of the whole kidney method and 46.2 ± 5.6 hours (range 37.8–57.7 hours) in the case of the SV method. A paired-samples t-test was conducted to compare the half-lives resulting from using the WKV and the SV method. There was no significant difference between the methods (p = 0.122). The effective half-lives using both methods for each patient are presented in .
There were no statistically significant differences between either method for the absorbed doses for all four treatment cycles (). However, three patients (12.5%) had an increase of 20% of absorbed for the fourth treatment compared to the first for the WKV method compared with eight patients (33.3%) when measured by the SV method for the corresponding treatment cycles. There were no significant differences between left or right kidneys (p = 0.86).
Table 2. A comparison of the whole kidney and the small volume methods for determining the mean absorbed kidney doses (Gy/GBq) and their ratios for right and left kidneys for 24 patients given four successive treatment cycles of 177Lu-octreotate at eight-week intervals.
The regional heterogeneity of the uptake of 177Lu-octreotate was detected in the ratio between the two methods (SV/WKV) when the results of the SV method were expanded to WKVs. When this ratio is 1.0, it means that the activity distribution is uniform in the WKV and the absorbed doses between the WKV and SV methods are equals. In this study the mean ratio for the 24 patients was 1.7 in four cycles (), ranging from 1.3 to 2.0. We could not verify a significant change in the heterogeneity of the Lu-177 activity, when the first two cycles to the last two cycles were compared (p = 0.064).
The correlation coefficient (R2) was low when using a single SPECT/CT scan compared to the results of the three time points by OLINDA (). The best correlation with all three time points for the OLINDA algorithm was obtained when time points of both 24 and 168 hours were used and when kidney volumes were also taken into account (EquationEquation 1(1) ). Results of the SV method 24 and 168 hours also correlated significantly with the results of all three time points ().
The mean calculated absorbed radiation dose for the cortex region determined by the SV method for the first treatment cycle was significantly (p < 0.001) lower, 0.41 ± 0.22 Gy/GBq (range 0.09–1.08) than that for the medulla region, 0.62 ± 0.27 Gy/GBq (range 0.28–1.59), (). No difference in the effective half-life was detected between cortex and the medulla.
Figure 3. (a) Calculated kidney doses (Gy) for the whole kidney method based on the OLINDA calculation with three time points correlated with the new equation for activities measured at 24 and 168 h after the 177Lu-octreotate treatment including individual kidney volumes. (b) Calculated kidney doses (Gy) for the small volume method based on the OLINDA calculation at three time points (dots) and the new equation for two time points at 24 and 168 h (line) after the 177Lu-octreotate treatment.
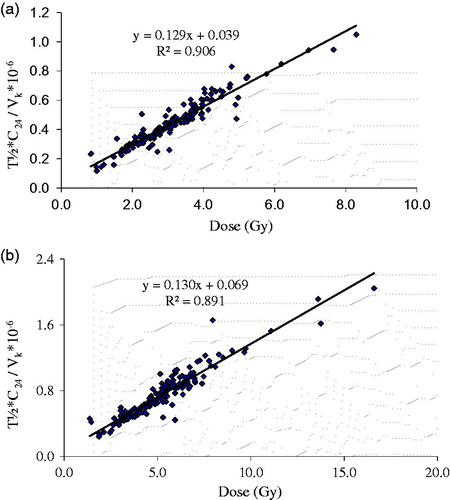
Figure 4. Calculated medulla and cortex doses for the small volume method based on the two time points calculation method and Equation 1.
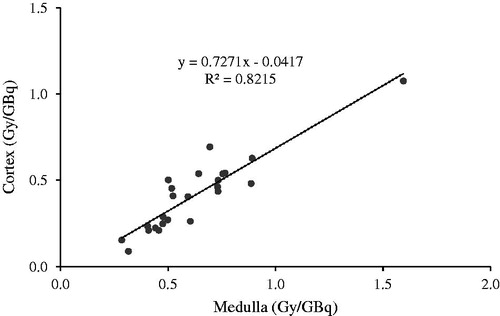
Table 3. Correlation coefficients R2 between the calculated kidney doses (Gy) and those given by the OLINDA method for three time points when the radiation dose calculations are based on a single SPECT/CT scan for different time points (a, b, c), and for two time points, 24 and 168 h from treatment (d) and when kidney volume is included to our current analysis (e).
Discussion
Prediction of the long-term toxicity of 177Lu-octreotate treatment is particularly important as this treatment modality is becoming widely used for patients with locally advanced or metastatic NETs with somatostatin-receptor expression. The treatment is often well tolerated. Acute side effects are mainly nausea caused by the amino acid solution that is used for kidney protection and pain that is probably due to the swelling of the metastases that occurs 3–5 days after the treatment. The dose-limiting organ in the long-term is the kidney, and it is more limiting for this treatment modality than bone marrow [Citation18]. However, persistent severe grade 3–4 kidney toxicity in 177Lu-octreotate treatment is a rare phenomenon when compared to 90Y-octreotide treatment modalities. A study by Kwekkeboom et al. [Citation9] reported that only two of the 504 patients that had been treated with 177Lu-octreotate that they investigated had severe renal insufficiency. We did not find any kidney toxicity with a follow-up time of two years from the first cycle.
The individual factors behind the increased susceptibility to nephrotoxicity are poorly understood. Age over 70 years and hypertension are two known risk factors for decreasing creatinine clearance and nephrotoxicity [Citation9,Citation19]. Barone et al. [Citation16] analyzed 18 patients that had been treated with 90Y-octreotide and found that those who received large radiation doses with little fractionation had the highest risk of developing nephrotoxicity. Bodei et al. [Citation12] studied dosimetry in 28 patients who were receiving radionuclide therapy with 177Lu-octreotate and found that the biologically effective dose of 28 Gy was safe and that 40 Gy was also safe for patients without the preexisting risk factors for renal disease, such as old age and hypertension. We gave reduced activity dosages to the following categories of patients: those who were older, frail, had hypertension, diabetes, received previous chemotherapy, signs of bone marrow toxicity or any combination of these. Despite this downward adjustment of the activity for seven patients, the absorbed dose to the kidneys was equal to that of full activity dosage patients.
Several methods that are based on planar imaging or SPECT/CT have been used in kidney dosimetry. Image correction methods have now reached a remarkably better level of performance than what could be achieved from SPECT without CT or by planar imaging. The extra challenge of in vivo dosimetry is caused by the kinetics/the pharmacokinetics of 177Lu-octreotate. A kidney time activity curve in SPECT/CT is close to a monoexponential function with a reasonably long half-life.
The overlapping uptake by hepatic metastases, spleen and intestine in planar imaging is often a confounding problem. This, however, is only a minor problem with the SV method where only a 4-cm3 volume of kidney is studied although the activity in the kidney pelvis may increase the apparent activity that is measured. The progressive heterogeneity of the uptake of 177Lu-octreotate in the kidney detected in the present study renders the SV method vulnerable to subjective variation between medical physicists. The WKV method gives a mean dose measurement for the kidneys, although nearby uptake can also affect this result to a minor extent compared to planar imaging.
Activity quantification from the SPECT images is critical part of targeted radionuclide dosimetry. In this study, we have applied a calibration method similar to the method used by Sandström et al. [Citation14] and which was later used in a clinical study [Citation20]. For the system calibration, we chose the calibration source so that the diameter of the source was larger than 2 × Full Width at Half Maximum (FWHM) resolution of the point source for all directions in the media with this system. The source was drilled inside an Alderson phantom to introduce scatter and attenuation media similar to patient studies. The source was homogeneous within the delineated VOI, the deviation of the voxel intensities being less than 4% within the high activity volume. This implies that the effect of the partial volume is small on calibration source. Recently, we have also studied the effect of reconstruction methods on quantification accuracy [Citation21]. We found out that using OSEM reconstruction algorithm with attenuation, scatter and resolution recovery compensation methods the activity quantification accuracy was around 10% for volumes larger than 30 ml. Based on these findings, we consider that our calibration method is valid.
We found significant variation in kidney volumes between patients. The importance of kidney size was demonstrated when the best correlation to the OLINDA-based calculations was obtained, which was when kidney size was included into the analysis. However, making individual measurements in SPECT/CT can be time consuming, therefore any innovation or modifications that would simplify the method are warranted. Larsson et al. [Citation6] used planar imaging and CT for estimating kidney size and absorbed doses. They concluded that the use of a standard kidney size led to either an underestimation or an overestimation of absorbed doses for 50% of their patients because of the wide variation between individual kidney masses.
The mean absorbed dose was 0.74 Gy/GBq for the SV method compared to 0.44 Gy/GBq with the WKV method. These results are in accordance with measurements reported by Larsson et al. and Sandström et al. [Citation6,Citation14], but are smaller than earlier reports by Kwekkeboom et al. and Cremonesi et al. [Citation1,Citation22]. Infusions of amino acid solutions are given to reduce tubular reabsorption and thus reduce the incidence of kidney toxicity in radionuclides therapy, but they also decrease the mean absorbed dose to the kidneys [Citation11,Citation12].
Garske et al. [Citation18] found that the elimination time/the half-life (T1/2) of the absorbed dose was quite stable or decreased from the first to fourth treatment cycle when early and late treatments were compared. Differences in the absorption elimination time over treatment cycles might become important when unexpected nephrotoxicity is detected.
Methods that are based on planar imaging give higher absorbed dose determinations than those calculated by SPECT/CT [Citation7,Citation8]. The SV method in our study gave a 1.7-fold higher result than the WKV method. This is not surprising because the former method can find the maximum dose within a 4 cm3 kidney volume, whereas the latter method produces a parameter close to the mean kidney dose within limitations of the modeling and measurement accuracy. Whenever tolerance dose limits are studied for kidneys in radionuclide therapy, the respective ratios for the methods might be useful for converting the limits from one dosimetry method to another. The trends in performance differences between calculation methods for external beam radiation therapy are currently narrowing to insignificant levels.
The variation between individuals for the absorbed dose was large and ranged from 0.22 to 1.06 Gy/GBq with the WKV method and from 0.36 to 1.91 Gy/MBq for the SV method. Other investigations have also found significant variations. The study by Larsson [Citation6] showed that the results of their planar scintigraphy measurements varied between 0.33 and 2.4 Gy/GBq. Kwekkeboom et al. reported absorbed doses of 1.65 ± 0.47 Gy/GBq (no amino acid protection) [Citation3], Cremonesi et al. 0.45–17.74 Gy/GBq [Citation23], Wehrmann et al. 0.9 ± 0.3 Gy/GBq [Citation15] and Sandström [Citation14] reported results that varied between 0.32 and 1.67 Gy/GBq. Hitherto very little correlation between dosimetry and complications in radionuclide therapy has been found.
Little is known about the mechanism of kidney damage in the different regions of the kidney and this is especially the case for kinetics/pharmacokinetics of radionuclides, such as177Lu-octreotate. There is also a paucity of data on 177Lu-octreotate and its correlations with the activity levels given, the number of treatments and time intervals between treatments.177Lu-octreotate is first excreted, but then some of it is reabsorbed and it accumulates in the kidney cortex. Konijnenberg et al. [Citation24] used autography studies to detect that higher amounts of 177Lu had accumulated in the kidney cortex than in the medulla. We, however, found higher absorbed doses in the medulla of the kidney compared to the cortex. One reason for this difference in findings between the two studies may be because we detected high activities in the kidney pelvis, which due its proximity, increases activity in the medulla. It is possible that factors other than total absorbed activity might also correlate with late toxicity. It has been reported that differences in the time activity curves can manifest as two main patterns that were either maximal at the first time point of one hour, or were maximal at the two later time points of 24 and 168 hours [Citation6]. A variation in kinetics in different regions of the kidney might also have effects that are unknown as yet. There were no differences in the effective half-lives found between the cortex and medulla in our study. However, it would be interesting to investigate whether high locally absorbed doses with prolonged retention could be found in patients who have developed nephrotoxicity.
We found major heterogeneity in the uptake of 177Lu-octreotate among a subgroup of patients. This heterogeneity was characterized by high ratios between the SV and the WKV methods for the absorbed doses. However, other patients had an almost uniform uptake as measured by the ratio between absorbed doses for the compared methods.
We found that it was possible to reduce the number of SPECT/CT images in our study from three to two time points taken at 24 and 168 hours after each treatment of 177Lu-octreotate. The study by Larsson et al. [Citation6] found that apart from Day 7, the imaging resulted in large variations in the absorbed dose. However, SPECT/CT imaging for dosimetry calculations should always be performed after each treatment as major individual changes in the absorbed doses occurred in some of our patients. The kidney volume should also be measured at least once after every treatment because kidney size was not stable for all patients. Garske et al. [Citation17] concluded that it is safe for most patients to use only the 24 hours activity concentration after the first treatment and assume that the half-life is stable in the following treatments. However, changes in tumor burden might also have consequences for the excretion of 177Lu-octreotate.
Kidney toxicity in 177Lu-octreotate treatment is a multidimensional phenomenon and its late manifestation after several years makes it difficult to detect and predict. Long-term follow-up is therefore most important. There is an urgent need for a standardized dosimetric method to be made available to all institutions that provide these treatment modalities. The method should concentrate on the components of toxicity that are the most relevant. The cutoff limits chosen will also predict major risks of clinical consequences, if they are exceeded. Different risk groups, such as patients with older age, hypertension, diabetes and former chemotherapy, should have their own stratifications for activity dosage. Individual patients with kidney toxicity should be carefully evaluated to find their specific predictive clinical and dosimetric variables.
Conclusions
Differences between the different dosimetric methods can be considered to be theoretical as clinical nephrotoxicity data cannot reliably be related to clinical effects. The kidney is considered to respond to irradiation as a parallel organ, therefore the most meaningful single parameter to describe the response is a mean absorbed dose for both kidneys. Therefore, mean absorbed dose calculations, such as those used in OLINDA, when used for calculating individual patient pharmacokinetics, should produce meaningful results when voxel-based methods are not available. The SV method is also a simple tool for estimating kidney dosimetry. We obtained around 1.7-fold higher for the SV method compared to the WKV method, but the SV method is more sensitive to subjective factors, such as the location of the selected kidney region.
Disclosure statement
The authors report no conflicts of interest. The authors alone are responsible for the content and writing of the paper.
References
- Kwekkeboom DJ, Bakker WH, Kooij PP, Konijnenberg MW, Srinivasan A, Erion JL, et al. [177Lu-DOTAOTyr3] octreotate: comparison with [111In-DTPAo] octreotide in patients. Eur J Nucl Med 2001;28:1319–25.
- Öberg K, Knigge U, Kwekkeboom D, Perren A, ESMO Guidelines Working Group. Neuroendocrine gastro-entero-pancreatic tumors: ESMO clinical practice guidelines for diagnosis, treatment and follow-up. Ann Oncol 2012;23:vii124–30.
- Janson ET, Sorbye H, Welin S, Federspiel B, Grønbæk H, Hellman P, et al. Nordic guidelines 2014 for diagnosis and treatment of gastroenteropancreatic neuroendocrine neoplasms. Acta Oncol 2014;53:1284–97.
- Kwekkeboom DJ, Kam BL, van Essen M, Teunissen JJ, van Eijck CH, Valkema R, et al. Somatostatin-receptor-based imaging and therapy of gastroenteropancreatic neuroendocrine tumors. Endocr Relat Cancer 2010;17:R53–73.
- Paganelli G, Sansovini M, Ambrosetti A, Severi S, Monti M, Scarpi E, et al. 177 Lu-Dota-octreotate radionuclide therapy of advanced gastrointestinal neuroendocrine tumors: results from a phase II study. Eur J Nucl Med Mol Imaging 2014;41:1845–51.
- Larsson M, Bernhardt P, Svensson JB, Wängberg B, Ahlman H, Forssell-Aronsson E. Estimation of absorbed dose to the kidneys in patients after treatment with 177Lu-octreotate: comparison between methods based on planar scintigraphy. EJNMMI Research 2012;2:49–61.
- Kam BLR, Teunissen JJ, Krenning EP, de Herder WW, Khan S, van Vliet EI, et al. Lutetium-labelled peptides for therapy of neuroendocrine tumours. Eur J Nucl Med Mol Imaging 2012;39:S103–12.
- Garkavij M, Nickel M, Sjögreen-Gleisner K, Ljungberg M, Ohlsson T, Wingårdh K, et al. 177Lu-[DOTA0,Tyr3] octreotate therapy in patients with disseminated neuroendocrine tumors: analysis of dosimetry with impact on future therapeutic strategy. Cancer 2010;116:1084–92.
- Kwekkeboom DJ, de Herder WW, Kam BL, van Eijck CH, van Essen M, Kooij PP, et al. Treatment with the radiolabeled somatostatin analog [177 Lu-DOTA 0,Tyr3]octreotate: toxicity, efficacy, and survival. J Clin Oncol 2008;26:2124–30.
- Strosberg J, Wolin E, Chasen B, Kulke M, Bushnell D, Caplin M, et al. 177Lu-DOTATATE significantly improves progression-free survival in patients with mid-gut neuroendocrine tumours: results of the phase III NETTER-1 trial [abstract LBA 6]. Presented at the ECC; 2015.
- Vegt E, de Jong M, Wetzels J, Masereeuw R, Melis M, Oyen W, et al. Renal toxicity of radiolabelled peptides and antibody fragments: mechanisms, impact on radionuclide therapy, and strategies for prevention. J Nucl Med 2015;51:1049–58.
- Bodei L, Cremonesi M, Ferrari M, Pacifici M, Grana CM, Bartolomei M, et al. Long-term evaluation of renal toxicity after peptide receptor radionuclide therapy with 90Y-DOTANOC and 177Lu DOTATATE: the role of associated risk factors. Eur J Nucl Med Mol Imaging 2008;35:1847–56.
- Thompson PL, Mackay IR, Robson GS, et al. Late radiation nephritis after gastric x-irradiation for peptic ulcer. Q J Med 1971;40:145–57.
- Sandström M, Garske U, Granberg D, Sundin A, Lundqvist H. Individual dosimetry of patients undergoing therapy with 177Lu-DOTA-D-Phe-Tyr-octreotate. Eur J Nucl Med Mol Imaging 2010;37:212–25.
- Wehrmann C, Senftleben S, Zachert C, Muller D, Baum RP. Results of individual patient dosimetry in peptide receptor radionuclide therapy with 177Lu DOTA-TATE and 177Lu DOTA-NOC. Cancer Biother Radiopharm 2007;22:406–16.
- Barone R, Borson-Chazot F, Valkema R, Walrandt S, Chaufin F, Gogou L, et al. Patient-specific dosimetry in predicting renal toxicity with 90Y-DOTATOC: relevance of kidney volume and dose rate in finding a dose-effect relationship. J Nucl Med 2005;46:995–1065.
- Garske U, Sandström M, Johansson S, Sundin A, Granberg D, Eriksson B, et al. Minor changes in effective half-life during fractionated 177Lu-octreotate therapy. Acta Oncol 2012;51:86–96.
- Garske U, Sandström M, Johansson S, Granberg D, Lundqvist H, Lubberink M, et al. Lessons on tumour response: imaging during therapy with (177)Lu-DOTA-octreotate. A case report on a patient with a large volume of poorly differentiated neuroendocrine carcinoma. Theranostics 2012;2:459–71.
- Valkema R, Pauwels SA, Kvols LK, Kwekkeboom DJ, Jamar F, de Jong M, et al. Long-term follow-up of renal function after peptide receptor radiation therapy with (90)Y-DOTA(0),Tyr(3)-octreotide and (177)Lu-DOTA(0), Tyr(3)-octreotate. J Nucl Med 2005;46:83S–91S.
- Sandström M, Garske-Roman U, Granberg D, Johansson S, Widström C, Eriksson B, et al. Individualized dosimetry of kidney and bone marrow in patients undergoing 177Lu-DOTA-octreotate treatment. J Nucl Med 2013;54:33–41.
- Hippelainen E, Tenhunen M, Maenpaa H, Sohlberg A. Quantitative accuracy of Lu-177 SPECT reconstruction using different compensation methods: phantom and patient studies. EJNMMI Res 2016;6:16–23.
- Cremonesi M, Ferrari M, Bodei L, Bartolomei M, Chinol M, Mei R, et al. Dosimetry in patients undergoing 177Lu-DOTATATE therapy with indications for 90Y-DOTATATE. Eur J Nucl Med Mol Imaging 2006;33:s102.
- Cremonesi M, Botta F, Di Dia A, Ferrari M, Bodei L, De Cicco C, et al. Dosimetry for treatment with radiolabelled somatostatin analogues. A review. Q J Nucl Mol Imaging 2010;54:37–51.
- Konijnenberg M, Melis M, Valkema R, Krenning E, de Jong M. Radiation dose distribution in human kidneys by octreotides in peptide receptor radionuclide therapy. J Nucl Med 2007;48:134–42.