Abstract
Background: Breath-holding (BH) is often used to reduce abdominal organ motion during radiotherapy. However, for inhale BH, abdominal tumor motion during BH has not yet been investigated. The aim of this study was to quantify tumor motion during inhale BH and tumor position variations between consecutive inhale BHs in pancreatic cancer patients.
Material and methods: Twelve patients with intratumoral fiducials were included and asked to perform three consecutive 30-second inhale BHs on each of three measurement days. During BH, lateral fluoroscopic movies were obtained and a two-dimensional (2D) image correlation algorithm was used to track the fiducials and the diaphragm, yielding the tumor and diaphragm motion during each BH. The tumor position variation between consecutive BHs was obtained from the difference in initial tumor position between consecutive BHs on a single measurement day.
Results: We observed tumor motion during BH with a mean absolute maximum displacement over all BHs of 4.2 mm (range 1.0–11.0 mm) in inferior-superior (IS) direction and 2.7 mm (range 0.5–8.0 mm) in anterior-posterior (AP) direction. We found only a moderate correlation between tumor and diaphragm motion in the IS direction (Pearson’s correlation coefficient |r|>0.6 in 45 of 76 BHs). The mean tumor position variation between consecutive BHs was 0.2 [standard deviation (SD) 1.7] mm in the inferior direction and 0.5 (SD 0.8) mm in the anterior direction.
Conclusion: We observed substantial pancreatic tumor motion during BH as well as considerable position variation between consecutive BHs on a single day. We recommend further quantifying these uncertainties before introducing breath-hold during radiation treatment of pancreatic cancer patients. Also, the diaphragm cannot be used as a surrogate for pancreatic tumor motion.
During fractionated radiation therapy, pancreatic tumors show large inter-fractional as well as intra-fractional position variation [Citation1,Citation2]. Daily positioning of the patient based on intratumoral fiducials improves the treatment accuracy significantly [Citation3]. To account for the respiratory-induced tumor motion, often a four-dimensional computed tomography (4DCT) is obtained and an internal target volume, encompassing the clinical target volume during the entire respiratory cycle, is created. Alternatively, other planning techniques can be used such as mid-ventilation. In mid-ventilation, the tumor is delineated in its mean respiratory position and a safety margin is calculated by regarding the respiratory tumor motion as random motion [Citation4]. Abdominal compression or technically more challenging techniques such as gating or tracking can also be employed to limit or account for tumor motion [Citation5,Citation6].
To eliminate respiratory-induced tumor motion, breath-holding (BH) techniques, either at exhale or at inhale, are widely used for a variety of clinical applications [Citation7–14]. For example, inhale BH is often used for breast cancer patients to increase the distance between the heart and the planning target volume [Citation13]. For abdominal tumors, BH is often applied to achieve a more stable tumor position. However, not all patients are able to perform BH, especially exhale BHs [Citation9–12]. Several groups investigated the positional reproducibility and stability of tumors and organs between and during multiple BHs within one fraction. Between consecutive exhale BHs within a single fraction, the position variation of anatomical landmarks close to the target volume has been reported to be up to 3.3 mm for pancreatic cancer patients [Citation12]. For the diaphragm and liver tumors during exhale BHs, good stability has been reported [Citation9,Citation11]. When using BH during radiotherapy, daily positioning based on cone-beam CT (CBCT) imaging while the patient is in BH should be used to account for inter-fractional position variations [Citation15]. Also, to improve the accuracy of the daily positioning of the patient, the image registration between the daily CBCT and pretreatment CT should be based on the diaphragm instead of bony anatomy [Citation16]. However, exhale BH durations are generally shorter compared with inhale, as performing exhale BHs can be more challenging for patients [Citation9,Citation11].
Inhale BHs may be more suitable for certain patient groups. For example, in abdominal cancer patients inhale BH is being used on a daily base in many clinics [Citation10,Citation14]. For inhale BHs, the position variation between consecutive BHs within one fraction has been reported to be up to 7.0 mm for the diaphragm [Citation7] and 2.5 mm for pancreatic tumors [Citation10]. Organ motion during inhale BHs has only been investigated for lung cancer patients; studies have reported motion magnitudes during inhale BHs of up to approximately 5.4 mm for the diaphragm and 3.8 mm for lung tumors [Citation7,Citation8]. During inhale BHs in healthy volunteers, drifts of the diaphragm of up to 15.0 mm in the superior direction have been observed [Citation17,Citation18]. These drifts may (partially) be attributed to the known decrease in lung volume during BH, which likely results in motion of the diaphragm [Citation19,Citation20]. Regarding abdominal tumor stability during inhale BH, nothing has been reported so far. Therefore, more research is needed to quantify the uncertainties during inhale BH.
The aim of this study was to quantify the motion of pancreatic tumors during voluntary inhale BHs as well as the position variation between consecutive BHs on a single day. We extensively investigated the tumor motion during inhale BH and the position variation between inhale BHs by using intratumoral fiducials and fluoroscopy in pancreatic cancer patients. The motion of the diaphragm during BH was measured as well to investigate the relation between tumor and diaphragm motion and to establish whether the diaphragm motion can be used as a surrogate for tumor motion during BH.
Material and methods
Patient population and feasibility
Twelve pancreatic cancer patients were included in the period April 2014 through June 2015. Included patients should be able to hold their breath for at least 30 seconds and should receive radiotherapy. As two patients quit the study before any measurements were performed, we included 12 consecutive patients in order to obtain BH measurements for 10 patients. Patients 11 and 12 were included in very close succession and were therefore both included in the analysis even though Patient 12 quit the study prematurely. The study was approved by the local ethics committee and all patients had given written informed consent. As part of standard clinical practice, each patient had been implanted with 2–4 intratumoral Visicoil fiducials (RadioMed, Barlett, TN, USA; thickness, 0.35 mm; length, 1.0 cm). The intratumoral fiducials were implanted using an endoscopic ultrasound-guided procedure [Citation21].
The patient group consisted of seven males and five females and the mean age was 66.7 years (range 57–80 year). Each patient was asked to perform 30-second inhale BHs as is used for many treatment sites, including abdominal tumors [Citation14]. On each measurement day, the patient, if able, performed three consecutive BHs on day 5, 10 and the last day of the treatment (i.e. day 13 or 15 depending on the treatment scheme). The BH measurements were performed after the patient was irradiated. For Patient 11, who had a treatment scheme of 20 fractions, the measurements were done on day 5, 10 and 15. On each measurement day, the patient was asked to score the feasibility of performing the three BHs by giving a grade from 1 (not achievable) to 10 (very easily achievable).
Breath-hold procedure and measurements
To compare the tumor motion during BH with the respiratory-induced tumor motion, for each patient the respiratory motion magnitude prior to each treatment fraction was obtained from the daily CBCT, using the method described in an earlier study from our group [Citation2].
Similar to the clinical BH protocol that is used for breast cancer patients at our department, each patient attended a 60-minute instruction session before he or she received the pretreatment planning CT scan. During this session the BH measurement protocol was explained and the ability of the patient to hold his or her breath for 30 seconds was tested. The physicians and radiation technologists that were involved in the study were extensively informed on the study protocol and during each BH measurement one of the main investigators was present.
During BH, patients were monitored using the SpiroDynr’X (SDX) system (Dyn’R, Aix-en-Provence, France), which registered airflow through a mouthpiece using a spirometer (). The airflow was logged and visualized and real-time visual feedback of the airflow was given to the patient through video goggles (). Airflow through the nose was prevented by using a nose clip.
Figure 1. The SpiroDynr’X (SDX) system with (a) the mouthpiece to measure airflow and the video goggles that are used to give visual feedback to the patient and (b) the image that is shown to the patient using the video goggles. The patient sees his or her breathing pattern and the green bar indicates the breath-hold window.
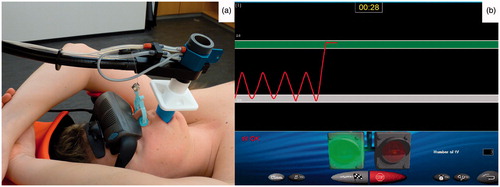
BHs were performed in inhale at 75% of the maximum inhalation volume, as is standard clinical practice in breast cancer patients at our institute. When the patient received his or her pretreatment planning CT scan, the maximum inhalation volume was determined using the SDX system and the BH window was set to 75% of that volume ±100 ml (indicated by the green bar in ). For each BH, the patient was asked to inhale until this window was reached and then hold their breath, after which the measurement was started. A BH was considered to be successful if the patient remained within the predetermined BH window for 30 seconds (i.e. no substantial airflow during BH), and this was monitored using the SDX system. When the patient was unable to reach or stay within the window, the measurement was aborted and the patient was asked to retry the BH.
During each BH, a fluoroscopic movie was made using the on-board CBCT imaging system (Synergy, Elekta Oncology systems, Crawley, UK) with the gantry angle fixed at 0° (lateral movies). Each 30-second movie consisted of 150 projection images (512 × 512 pixel2) in which the implanted intra-tumoral fiducials were visible. As these fiducials have been shown to be representative of tumor position [Citation1], we equated their motion to that of the tumor.
To track the tumor position, we measured the displacement of the fiducials in each frame using an in-house developed algorithm in MATLAB (The MathWorks Inc., Natick, MA). In the first frame a rectangular region, placed tightly around the fiducials, was selected as the template. To save computation time all frames were cropped to a smaller region that contained all possible fiducials positions during the entire movie. Next, a Prewitt edge enhancement filter was applied to each image. Then, 2D image correlation was used to find the most likely location of the template within each subsequent frame. This was done by calculating a zero-normalized cross-correlation coefficient for every possible template position and selecting the position with the highest coefficient [Citation22]. This method provided the displacement of the template for every movie frame, resulting in the motion of the intratumoral fiducials in the inferior-superior (IS) and anterior-posterior (AP) direction during a single BH. The motion was obtained with a resolution of one pixel, which in the patient corresponded to 0.5 mm at the isocenter. For each movie we visually confirmed that the template was correctly matched to the fiducials.
The motion of the diaphragm was also tracked to investigate the relation with tumor motion. The diaphragm motion was obtained using the same tracking procedure, only now the template was defined as a thin (width of 3–5 pixels) vertical region including the diaphragm–lung transition at the top of the dome of the diaphragm. For the diaphragm we only extracted the motion in the IS direction. To illustrate the motion during breath-hold, the first BH movie of the first three patients are given in the supplementary materials (Supplementary Movies A1–A3, available online at http://www.informahealthcare.com). Projection images from these movies demonstrate fiducial tracking by the algorithm (Supplementary Figures B1–B3 and Supplementary Table C1, available online at http://www.informahealthcare.com).
To quantify the tumor position variation between consecutive BHs within a single fraction in IS and AP direction, the positions of the fiducials in the first frame of each movie were determined manually. This allowed us to determine the positions of the individual fiducials and from these positions the center of mass of the fiducials was calculated. The difference in center of mass position between the second and first BH and between third and first BH resulted in two inter-BH data points per measurement day. The same was done for the top of the dome of the diaphragm position variation in the IS direction.
Both the tumor motion during individual BHs and the position variation between consecutive BHs on a single day only contribute to the intra-fractional uncertainty; as all displacements were measured relative to the first BH of that day, the inter-fractional uncertainty was eliminated. Also, we assumed that there was no fiducial migration during a single measurement day. When a treatment fraction requires multiple BHs and the patient is positioned using a CBCT obtained during the first BH, the only relevant uncertainty is that of the tumor position relative to the isocenter within the following BHs. To determine whether there were patient shifts between BHs, we measured the position variation of the vertebra at the level of the tumor using the aforementioned 2D image correlation.
Data analysis
For each fluoroscopic movie the tumor displacement over time relative to the initial tumor position during the first BH was plotted for the IS and AP direction separately; inferior and anterior were defined as the negative directions. We determined the maximum displacement in each of the four directions and defined the magnitude of motion in IS and AP as the distance between the two maximum displacements in the respective directions within a single BH. The same was done for the diaphragm (IS only).
For the respiratory motion magnitude, the motion magnitudes during BH as well as the position variations between BHs, the mean and standard deviation (SD) over the patient group were determined by first calculating the mean per patient and averaging these means over all patients.
Correlation plots of the tumor motion during BH in both IS and AP versus the diaphragm motion in IS were generated and simple linear regression was performed. Pearson’s correlation coefficients (r) were calculated for each BH. We also calculated r between the position variation between consecutive BHs of the diaphragm in IS and the position variation of the tumor in IS and in AP over all patients and measurement days. Correlations were defined as strong when 0.6≤|r|<0.8 and very strong when |r|≥0.8.
The airflow over time, as obtained from the SDX system, could not be related one-to-one to the fluoroscopic movies, because the system saved the airflow data using a variable time scaling. Therefore, only a qualitative comparison was performed between the airflow during BH and the motion of tumor and diaphragm.
Results
We obtained a total of 76 30-second inhale BHs. The BH procedure was reasonably well tolerated by the majority of the patients; for six patients, all nine BHs were performed successfully. For six patients a total of 32 BHs were not performed due to technical difficulties (eight BHs) or physical inability of the patient to perform the requested BH (24 BHs). Patients 7 and 12 quit the study before completing a single BH. A mean feasibility grade of 7.2 was given by the patient group ().
Table 1. Breath-holding feasibility, tumor motion magnitudes during breath-holding, tumor position variations between breath-holds and mean respiratory magnitude for each patient.
The group mean absolute tumor motion magnitude during BH was 4.2 (SD 2.3) mm and 2.7 (SD 1.1) mm in the IS and AP direction, respectively, whereas the group mean absolute respiratory motion magnitude in IS as measured on CBCT was 6.9 (SD 2.8) mm (). The range of motion magnitudes during all BHs was 1.0–11.0 mm and 0.5–8.0 mm in the IS and AP direction, respectively. The tumor motion was predominantly in the superior and posterior directions (), compared with the inferior and anterior directions, respectively, and mostly manifested itself as a slow drift during the entire 30-second BHs (). The data for all patients are shown in Supplementary Figures B6–B15 (available online at http://www.informahealthcare.com). We observed large day-to-day variations in the tumor motion during BH per patient as well as between patients ().
Figure 2. For each patient, the daily mean respiratory-induced motion magnitudes of the fiducials measured on CBCT (light boxes) and the motion magnitudes during breath-hold of the fiducials measured using fluoroscopy (dark boxes). The group mean respiratory motion magnitude and group mean motion magnitude during breath-hold were calculated over the 12 and 10 available patients, respectively. Boxes: median value and upper and lower quartiles; whiskers: lowest and highest data point within 1.5 × inter-quartile range; dots: outliers.
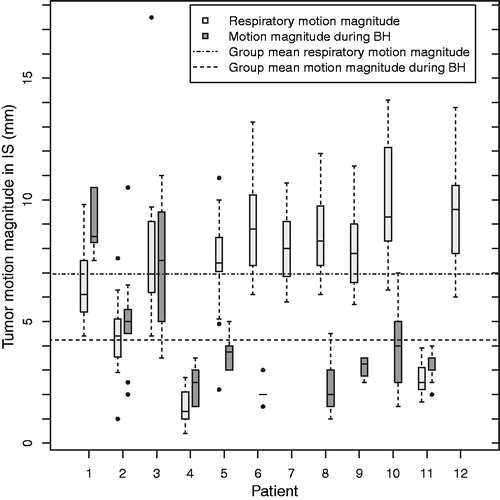
Figure 3. Histograms for the motion during breath-hold and position variation between consecutive breath-holds for fiducials in IS (a,b), fiducials in AP (c,d) and diaphragm in IS (e,f) for all 76 analyzed breath-holds.
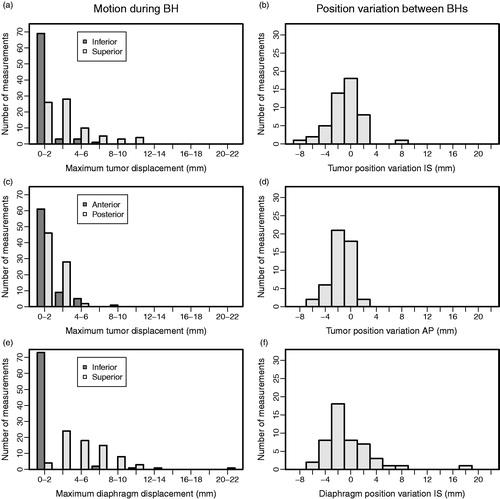
Figure 4. Motion during breath-hold of the fiducials (a–c) and the diaphragm (d–f) and the correlation plots of diaphragm motion versus fiducial motion (g–i) for two measurement days of Patient 3 and one of Patient 4, illustrating the large variations that can be seen within days, between days and between patients. IS displacements of fiducials and diaphragm (g–i) are relative to their position in the first frame of that breath-hold.
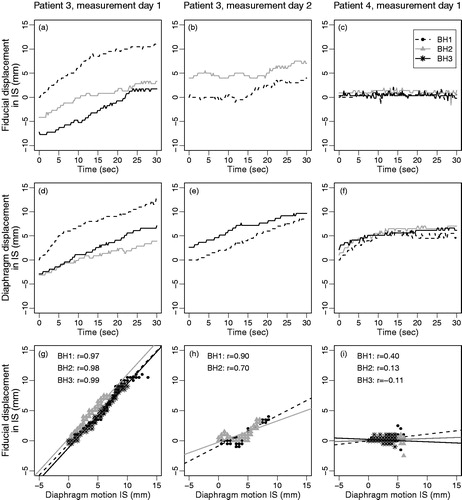
For the diaphragm we observed a group mean absolute motion magnitude during BH of 5.9 (SD: 2.5) mm in the IS direction, with a range of motion magnitudes during all BHs of 1.0–21.0 mm. The motion was almost exclusively in the superior direction (). Also, this motion manifested itself as a slow drift ().
We observed a group mean tumor position variation between consecutive BHs of -0.2 (SD 1.7) mm and -0.5 (SD 0.8) mm in the IS and AP direction, respectively (). For the diaphragm position variation in IS between consecutive BHs we found a group mean of 0.5 (SD 2.9) mm (). For tumor and diaphragm, the range over all BHs was large, up to 19.9 mm (). For the vertebra, the position variation between consecutive BHs was in IS always ≤1 mm and in AP ≤1 mm in 45 of 49 measurements (Supplementary Figures B4 and B5, available online at http://www.informahealthcare.com).
Between the diaphragm motion in IS and tumor motion we observed a very strong correlation (|r|≥0.8) in only 31 (IS) and 17 (AP) of 76 BHs; a strong correlation (0.6≤|r|<0.8) was found in 14 (IS) and 20 (AP) of 76 BHs (). However, the relation between the tumor and diaphragm motion often deviated considerably from a one-to-one relation (). A mean slope of the lines fitted to the correlation plots between tumor and diaphragm motion in IS during all BHs of 0.6 (range -0.5–2.3; SD 0.5) was observed and between the tumor motion in AP and diaphragm motion in IS the mean slope was 0.2 (range -0.5–1.8; SD 0.4) (). This indicates that there was no clear relation between the motion of the tumor and of the diaphragm. For the position variation between BHs, the Pearson’s correlation coefficient between the diaphragm and the fiducials was 0.81 in IS and -0.08 in AP direction.
Figure 5. Histograms of the Pearson’s correlation coefficients r (left) and of the slopes of the linear fits (right) in the correlation plot of tumor motion versus inferior-superior diaphragm motion during breath-hold, for tumor motion in (a and b) inferior-superior and (c and d) anterior-posterior direction for all 76 breath-holds.
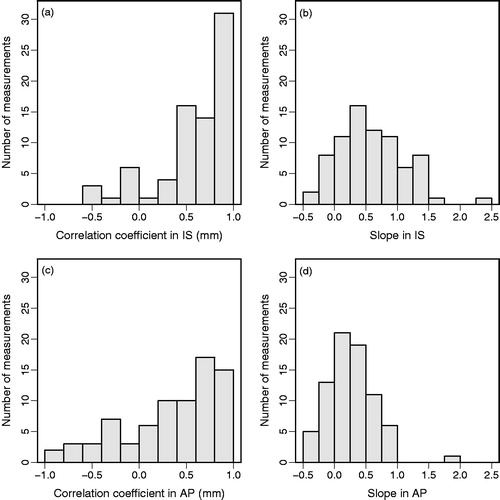
Figure 6. For the three consecutive breath-holds on measurement day 1 of patient 9, the motion during breath-hold of (a) the fiducials and (b) the diaphragm. In (c), the airflow as measured by the spirometer, with the x-axis in arbitrary units (see text).
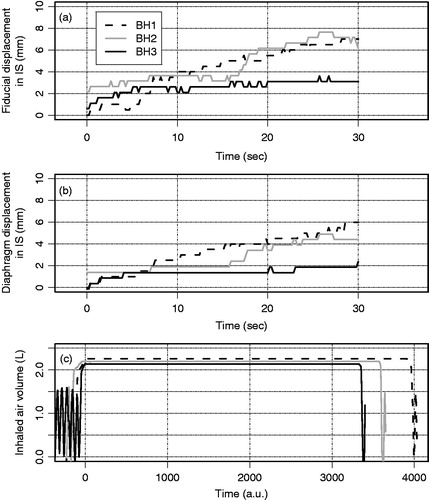
The airflow data from the SDX system were obtained for 72 BHs; for four BHs no airflow data were obtained due to technical difficulties. A non-zero airflow was observed in only a minority of BHs. In these cases, the airflow was small, mostly stepwise in nature and did not explain the observed tumor and diaphragm motion. shows an example of three consecutive BHs for patient 9, during which considerable tumor and diaphragm motion during BH was observed in the IS direction, but no airflow had occurred.
Discussion
We investigated the pancreatic tumor motion during 30-second inhale BHs as well as the position variation between consecutive inhale BHs using intratumoral fiducials and fluoroscopy while monitoring the airflow through the patient’s mouth. We showed that there was substantial tumor motion during individual BHs as well as considerable tumor position variation between consecutive BHs during a single measurement. These observed uncertainties may have a high dosimetric and thus clinical impact when using BH during radiation treatment.
During BH, uptake of O2 from the lungs continues without replenishment of CO2 from the bloodstream, resulting in a net decrease in lung volume during BH [Citation20]. The strong to very strong correlations between tumor and diaphragm motion in IS (59% of BHs) indicate that the decrease of lung volume, and concomitant motion of diaphragm, may play a large role in tumor motion during BH. However, because the correlation was not consistent and because we observed a wide range of slopes of the correlation plots, it is impossible to describe the relation between the motions of the two structures with a single model. Therefore, the diaphragm is unsuitable as a surrogate for pancreatic tumor motion during BH. The patients might also slowly relax their thoracic or abdominal muscles after the effort of inflating their lungs and this could partially explain the observed motion. However, the absence of a relation between the tumor motion during BH and the airflow measured with the spirometer indicates that the motion cannot be explained by air escaping during BH.
Whether the use of BH is beneficial compared with free breathing depends on multiple factors. Between the group mean respiratory-induced motion magnitude obtained from daily CBCTs and the group mean absolute tumor motion during a single BH () we observed only a small difference (2.7 mm in IS) for this patient group. However, there is a large variation between patients in the motion magnitudes during BH, the respiratory motion magnitudes as well as in the difference between these two motion magnitudes. Also, in earlier work we showed that there can be a large mismatch between the daily respiratory motion and the motion measured on the pretreatment 4DCT [Citation2], which can lead to a suboptimal treatment if the patient is breathing freely during treatment. Further research is needed to be able to determine beforehand which patients may benefit from BH and to investigate whether it is beneficial compared with other techniques to control respiratory motion such as abdominal compression or shallow breathing [Citation5,Citation23].
The use of a window at 75% of the maximum inhalation volume in this study was derived from the breast cancer treatment protocol used in our department. For breast cancer the objective of BH is to increase the distance between the irradiated volume and the heart. In this study, BH was aimed at increasing positional stability and reproducibility of the tumor position; therefore, it is possible that a different approach such as a window at >75% would yield better results. Future studies investigating different BH windows at different lung volumes should therefore be initiated. Also, the patient might benefit from a more elaborate training in stabilizing the abdominal muscles; it has been shown that using an active breath control system to induce BH in combination with training extended BH duration significantly [Citation24].
In the literature, it has been shown in 12 volunteers that the duration of BH can be safely extended to a mean of up to 5.5 minutes when pre-oxygenation and hypocapnia are introduced [Citation25]. If BHs of several minutes can be used during treatment, the daily positioning of the patient and the actual delivery of the radiation could be executed within a single BH. As this will eliminate inter-BH position variation, the use of a single BH may reduce geometric uncertainty.
If the remaining uncertainties of using an optimized BH technique will require safety margins that are still comparable to the clinical target volume enlargement needed to form an internal target volume, it may be concluded that the use of BH to stabilize the tumor is not optimal. Due to the nature of the observed uncertainties during and between BHs (e.g. systematic drifts in a part of the patients), calculation of the required safety margins is not straightforward and more research is needed to determine these margins. Also, the tumor motion magnitude during BH varied considerably from one BH to the next and this could result in substantial inter-fractional uncertainties in the tumor position that would also need to be accounted for. It might be better to use a mid-ventilation approach combined with free breathing as this results in target volumes that are significantly smaller compared with the use of an internal target volume [Citation4].
In conclusion, we observed substantial tumor motion during 30-second BHs as well as considerable tumor position variation between consecutive BHs. We strongly recommend quantifying these residual uncertainties further before introducing breath-hold during radiation treatment of pancreatic cancer patients. The tumor and diaphragm motion during BH manifested itself as a slow drift. However, due to the absence of a clear relation between tumor and diaphragm motion, the diaphragm cannot be used as a surrogate for tumor motion. Future research should focus on improving the breath-hold procedures so that the benefit compared with free breathing can be augmented.
Appendix_Movie_A3.wmv
Download (10.6 MB)Appendix_Movie_A2.wmv
Download (10.5 MB)Appendix_Movie_A1.wmv
Download (10.6 MB)Supplementary_material__revised_.pdf
Download PDF (2.6 MB)Acknowledgements
This work was supported by the foundation Bergh in het Zadel through the Dutch Cancer Society (KWF Kankerbestrijding) project no. UVA 2011-5271.
Disclosure statement
Dr Bel does consultancy work for Nucletron, outside the submitted work. The authors alone are responsible for the content and writing of the paper.
References
- van der Horst A, Wognum S, Dávila Fajardo R, et al. Interfractional position variation of pancreatic tumors quantified using intratumoral fiducial markers and daily cone beam computed tomography. Int J Radiat Oncol Biol Phys 2013;87:202–8.
- Lens E, van der Horst A, Kroon PS, et al. Differences in respiratory-induced pancreatic tumor motion between 4D treatment planning CT and daily cone beam CT, measured using intratumoral fiducials. Acta Oncol 2014;53:1257–64.
- van der Horst A, Lens E, Wognum S, et al. Limited role for biliary stent as surrogate fiducial marker in pancreatic cancer: stent and intratumoral fiducials compared. Int J Radiat Oncol Biol Phys 2014;89:641–8.
- Lens E, van der Horst A, Versteijne E, et al. Dosimetric advantages of midventilation compared with internal target volume for radiation therapy of pancreatic cancer. Int J Radiat Oncol Biol Phys 2015;92:675–82.
- Heinzerling JH, Anderson JF, Papiez L, et al. Four-dimensional computed tomography scan analysis of tumor and organ motion at varying levels of abdominal compression during stereotactic treatment of lung and liver. Int J Radiat Oncol Biol Phys 2008;70:1571–8.
- Verellen D, Depuydt T, Gevaert T, et al. Gating and tracking, 4D in thoracic tumors. Cancer/Radiothérapie 2010;14:446–54.
- Kim DJW, Murray BR, Halperin R, et al. Held-breath self-gating technique for radiotherapy of non – small-cell lung cancer: a feasibility study. Radiat Oncol 2001;49:43–9.
- Barnes EA, Murray BR, Robinson DM, et al. Dosimetric evaluation of lung tumor immobilization using breath hold at deep inspiration. Int J Radiat Oncol Biol Phys 2001;50:1091–8.
- Dawson LA, Brock KK, Kazanjian S, et al. The reproducibility of organ position using active breathing control (ABC) during liver radiotherapy. Int J Radiat Oncol Biol Phys 2001;51:1410–21.
- Murphy MJ, Martin D, Whyte R, et al. The effectiveness of breath-holding to stabilize lung and pancreas tumors during radiosurgery. Int J Radiat Oncol Biol Phys 2002;53:475–82.
- Eccles C, Brock KK, Bissonnette JP, et al. Reproducibility of liver position using active breathing coordinator for liver cancer radiotherapy. Int J Radiat Oncol Biol Phys 2006;64:751–9.
- Nakamura M, Shibuya K, Shiinoki T, et al. Positional reproducibility of pancreatic tumors under end-exhalation breath-hold conditions using a visual feedback technique. Int J Radiat Oncol Biol Phys 2011;79:1565–71.
- Nissen HD, Appelt AL. Improved heart, lung and target dose with deep inspiration breath hold in a large clinical series of breast cancer patients. Radiother Oncol 2013;106:28–32.
- Boda-Heggemann J, Knopf A-C, Simeonova A, et al. Deep inspiration breath hold-based radiation therapy: a clinical review. Int J Radiat Oncol Biol Phys 2015;94:478–92.
- Nakamura M, Akimoto M, Ono T, et al. Interfraction positional variation in pancreatic tumors using daily breath-hold cone-beam computed tomography with visual feedback. J Appl Clin Med Phys 2015;16:5123.
- Kawahara D, Ozawa S, Kimura T, et al. Availability of applying diaphragm matching with the breath-holding technique in stereotactic body radiation therapy for liver tumors. Phys Med 2016;32:557–61.
- Holland AE, Goldfarb JW, Edelman RR. Diaphragmatic and cardiac motion during suspended breathing: preliminary experience and implications for breath-hold MR imaging. Radiology 1998;209:483–9.
- Danias PG, Stuber M, Botnar RM, et al. Navigator assessment of breath-hold duration: impact of supplemental oxygen and hyperventilation. Am J Roentgenol 1998;171:395–7.
- Hong SK, Lin YC, Lally DA, et al. Alveolar gas exchanges and cardiovascular functions during breath holding with air. J Appl Physiol 1971;30:540–7.
- Parkes MJ. Breath-holding and its breakpoint. Exp Physiol 2006;91:1–15.
- Dávila Fajardo R, Lekkerkerker SJ, van der Horst A, et al. EUS-guided fiducial markers placement with a 22-gauge needle for image-guided radiation therapy in pancreatic cancer. Gastrointest Endosc 2014;79:851.
- Pan B, Qian K, Xie H, et al. Two-dimensional digital image correlation for in-plane displacement and strain measurement: a review. Meas Sci Technol 2009;20:062001.
- Uematsu M, Shioda A, Suda A, et al. Intrafractional tumor position stability during computed tomography (CT)-guided frameless stereotactic radiation therapy for lung or liver cancers with a fusion of CT and linear accelerator (FOCAL) unit. Int J Radiat Oncol Biol Phys 2000;48:443–8.
- Zhong R, Wang J, Jiang X, et al. Hypofraction radiotherapy of liver tumor using cone beam computed tomography guidance combined with active breath control by long breath-holding. Radiother Oncol 2012;104:379–85.
- Parkes MJ, Green S, Stevens AM, et al. Assessing and ensuring patient safety during breath-holding for radiotherapy. Br J Radiol 2014;87:20140454.