Abstract
Background: The purpose was to evaluate safety and efficacy of intensity-modulated radiotherapy (IMRT) following extra-pleural pneumonectomy (EPP) for malignant pleural mesothelioma (MPM).
Material and methods: Patients with MPM of clinical stage I–III, which were macroscopic completely resected with EPP were eligible for this prospective study. The ipsilateral hemithorax was irradiated with a prescribed dose of 50.4 Gy. When the high-risk surgical margins or FDG-avid regions were identified, simultaneous integrated boost (SIB) with 56.0 Gy or 61.6 Gy was applied.
Results: Twenty-one patients were enrolled. SIB was applied to five patients. The planned IMRT fractions were completed in all, but four patients who suffered from severe fatigue or radiation pneumonitis. With a potential median follow-up of 6.3 years, overall survival was 37.5% at 3 years since the IMRT. The median survival time was 17.5 and 27.0 months since the IMRT and the initial treatment, respectively. Three patients have survived for more than 5 years. Distant metastasis was observed in 15 patients. Local recurrence was also observed in 2 of the 15 patients. Acute toxicities of Grade 3 or worse were observed in 15 patients, including 9 with hematological, 3 with pneumonitis and 6 with fatigue, nausea or vomiting. Five patients developed Grade 3 or worse late toxicities associated with IMRT, consisting of one with persistent Grade 4 thrombocytopenia, one with brain infarction and congestive liver dysfunction, and three with elevation of serum transaminase or biliary enzyme. No Grade 5 toxicity was observed. Patients with N2 showed significantly worse survival than those with N0–1 (18.2% vs. 60.0% at 3 years, p = .014).
Conclusion: IMRT following EPP achieved excellent local control for MPM, that might lead to the long-term survival in selected patients. However, treatment burden including acute and late toxicities should be considered in this treatment approach.
Introduction
Malignant pleural mesothelioma (MPM) is a rare, but increasing disease. The number of mesothelioma death in Japan has increased from 500 in 1995 to 1376 in 2014 [Citation1]. The incidence rate is predicted to reach its peak around 2020 or beyond in European countries, Australia and Japan [Citation2]. MPM is an aggressive tumor with a pessimistic prognosis of 12 months or less [Citation3]. Efficient and safe treatment strategy is awaited for MPM.
The standard treatment for MPM is still controversial. Trimodality approach consisting of extra-pleural pneumonectomy (EPP) with induction or adjuvant chemotherapy and following radiotherapy is the most radical treatment. However, local recurrence is a dominant pattern of failures even with such a radical treatment [Citation4]. Radiotherapy can contribute to control microscopic lesions after the surgery if the lesions are appropriately irradiated. However, a conventional way of radiotherapy is difficult to cover the entire lesions because the clinical target volume (CTV) is in an irregular shape adjacent to radiosensitive organs including the contralateral lung, heart, liver, kidneys and spinal cord [Citation5].
Intensity-modulated radiotherapy (IMRT) is an advanced form of radiotherapy delivery. IMRT has a potential to improve local control of MPM through conformal dose distributions to such an irregularly shaped target and through simultaneous integrated boost (SIB) to high-risk target volumes [Citation6]. We conducted a prospective trial of IMRT in the trimodality treatment for MPM. The purpose of the study was to evaluate safety and efficacy of IMRT.
Material and methods
Inclusion criteria
The inclusion criteria for the studies were: (1) histologically confirmed MPM, (2) clinical stage of I-III disease which were macroscopic completely resected with EPP, (3) age of <75, (4) ECOG performance status of 0–1, (5) normal function of the contralateral kidney, (6) no other serious organ dysfunction, and (7) written informed consent. The exclusion criteria were: (1) prior non-curative treatment including incomplete resection or palliative radiotherapy for the MPM, (2) no diaphragm reconstruction, (3) synchronous or metachronous cancer within 5 years, and (4) a prior history of thoracic irradiation. There were no exclusion criteria on histologic type of the MPM. This clinical trial was approved by the institutional review board of Kyoto University Hospital (UMIN-CTR 000002217).
IMRT procedures
An 18F-fluorodeoxyglucose positron emission tomography (FDG-PET) scan was done to evaluate residual lesions on the day for IMRT simulation. The FDG-avid region was determined in consultation with nuclear medicine physicians. At the simulation, the patient’s body was immobilized in an individualized vacuum pillow (BodyFIX; Elekta AB, Stockholm, Sweden) with both arms overhead. The incision and drainage sites were marked with radio-opaque wires. No bolus was used. Ten respiratory phases of 4D CT were acquired in axial cine mode using a multi-slice CT scanner (LightSpeed RT; GE Healthcare, Little Chalfont, UK) and a real-time positioning management system (Varian Medical Systems, Palo Alto, CA). Averaged images from the ten phases were used for target delineation and dose calculation.
CTV was defined as the ipsilateral hemithorax including 5-mm margins plus the pleural space, diaphragm, its crus, drain sites, and nodal stations of ipsilateral mediastinum, hilum, subcarina and involved nodes. CTV-c was set for the surgical clips with 5-mm margins where surgical margins were close to the resected tumor. CTV-g was defined as the FDG-avid regions plus 5-mm margins. These contours were drawn in consultation with the surgeon to identify specific regions. The PTV, PTV-c and PTV-g were respectively defined as the CTV, CTV-c and CTV-g plus 5-mm margins. Prescribed dose was 50.4 Gy in 28 fractions to the PTV, with SIB of 56.0 Gy to the PTV-c and 61.6 Gy to the PTV-g. Organs at risk (OARs) including the contralateral lung, heart, bilateral kidneys, liver and spinal cord were delineated. IMRT plan was built with a commercial treatment planning system (Eclipse, Varian Medical Systems). The restricted field technique proposed by Allen et al. [Citation7] was applied to reduce doses to the contra-lateral lung. Dose constraints for the OARs are indicated in .
Table 1. Pre-determined constraints on dose-volume parameters for organs at risk and those from treatment plans.
Irradiation was performed with 15-MV x-ray beams from a linear accelerator (Clinac 2300 C/D till April 2008 and Clinac iX thereafter; Varian Medical Systems) using a sliding window technique in multiple coplanar ports till February 2013, and, thereafter, using volumetric modulated arc therapy (VMAT) in 3 or 4 arcs. No concurrent chemotherapy was administered.
Follow-up
Follow-up visit was planned every 3 month and CT was performed every 6 month within the initial 2 years after the treatment. Thereafter, every 4-month visit and annual CT was scheduled. Local recurrence was defined as recurrence in the ipsilateral thorax or mediastinal lymph nodes. The present paper defines overall survival (OS) as a period from the start date of IMRT to the date of death of any cause, and it is censored at the last follow-up date when the patient is alive. Toxicities were assessed based on the Common Terminology Criteria for Adverse Events (CTCAE) version 3.0. Acute toxicities were defined as treatment-related adverse events which occurred within the initial 6 months since the IMRT started. The treatment-related adverse events later than 6 months were classified as late toxicities.
Statistical analysis
The potential median follow-up period was estimated with the reverse Kaplan–Meier method [Citation8]. OS and disease progression were estimated with the Kaplan–Meier method. Survival difference was evaluated by the log-rank test. p Value < .05 was considered as a statistical significance.
Results
Patients
Twenty-one patients who had received EPP with pre- or post-operative chemotherapy were enrolled into the study between November 2006 and June 2013. Patient characteristics and the details on treatments before IMRT were summarized in . The initial treatment was EPP followed by adjuvant chemotherapy in 16 patients, and neo-adjuvant chemotherapy and EPP in the remaining 5 patients. Median interval between the initial treatment and IMRT was 6.0 months (range, 4.4–16.7 months).
Table 2. Patient characteristics.
Compliance of IMRT
The planned prescription dose to the PTV was 50.4 Gy, except for the first patient with 50 Gy. SIB was applied to 5 patients (2 patients to the PTV-c and 3 patients to the PTV-g, respectively). Dose-volume parameters in the OARs are shown in . The constraint of V5 (volume receiving ≥5 Gy) for the contralateral lung was cleared in all patients except one who had an expansile tumor to the contralateral mediastinum. The V40 for the heart were hardly satisfied, especially in left-sided cases (median, 34.5% and 60.2% for right- and left-sides cases, respectively). The mean heart dose was 31.0 Gy and 40.6 Gy in median for right- and left-side cases, respectively. The liver constraint was not satisfied in three patients with right-sided disease. The planned IMRT fractions were completed in 17 patients. The delivered dose to the PTV was reduced to 44 Gy in one patient because of Grade 3 fatigue, who recovered from the fatigue at 2 months after IMRT completion. Three patients developed Grade 2 or 3 radiation pneumonitis at 41.4–45.0 Gy of IMRT course. The acute radiation pneumonitis was successfully treated with corticosteroids.
Toxicities
Toxicities related to IMRT were summarized in . Acute toxicities of Grade 3 or worse were observed in 15 patients including the four patients described above with reduced IMRT doses. Of the 18 patients who had survived for 6 months or more, five patients developed Grade 3 or worse late toxicities associated with IMRT. One patient suffered from persistent Grade 4 thrombocytopenia which continued from the acute toxicity. One patient who had left-sided mesothelioma developed Grade 4 brain infarction due to cardiogenic embolism at 5.5 years. The patient also developed Grade 3 elevation of serum biliary enzyme, which was diagnosed as congestive liver dysfunction at 4 years after the IMRT. These late toxicities in the patient were considered to be likely related with irradiation to the heart with V40 of 60.7% and mean dose of 41.0 Gy (). Three patients experienced Grade 3 elevation of serum transaminase or biliary enzyme at 6–8 months, which showed spontaneous remission to Grade 2 or less without any medication. The liver V30 and the heart V40 in the three patients were 37.5–57.0 and 29.9–48.5%, respectively. No Grade 5 toxicity was observed.
Figure 1. Dose distribution in a patient who developed severe cardiac toxicity. Maximal dose, mean dose and V40 of the heart were 57.0 Gy, 41.0 Gy and 60.7%, respectively.
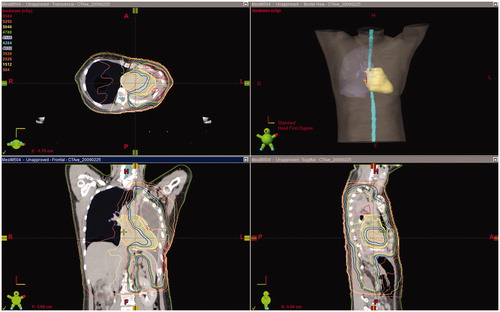
Table 3. Acute and late toxicities grade 2 or worse.
Survival outcomes
The potential median follow-up was 6.3 years (range, 0.3–8.7 years). The median survival time was 17.5 months (95% confidence interval [CI], 9.0–48.3 months) since the start date of IMRT (). One-, 2- and 3-year OS was 61.9, 47.6 and 37.5% (95%CI, 38.1–78.8, 25.7–66.7 and 17.7–57.4%), respectively. A median survival since the initial treatment was 27.0 months (95%CI, 17.1–53.2 months). Three patients have survived for more than 5 years after the IMRT. Disease progression was observed in 15 patients with a 3-year incidence of 75.8% (95%CI, 55.4–91.7%). The most dominant pattern of disease progression was distant metastasis, which was observed in all of the 15 patients. Local recurrence was also observed in 2 of the 15 patients. The 3-year incidence of local recurrence was 12.3% (95%CI, 3.2–41.2%).
When dividing the patient cohort into two groups according to nodal status, patients with N2 showed significantly worse OS than those with N0–1 (18.2% vs. 60.0% at 3 years, p = .014; ). Survival difference between epithelioid and non-epithelioid histology was not significant (34.3% for epithelioid and 50.0% for non-epithelioid at 3 years, p = .286).
Discussion
This study revealed that IMRT contributed to the excellent local control after EPP for MPM. According to our previous report on trimodality treatment including EPP and radiotherapy (with conventional technique for most of the patients) [Citation4], the most dominant pattern of failure was local recurrence (7 of the 16 cases). The present study had only two local recurrences in the 21 enrolled cases. Better target coverage using IMRT can lead to the reduction of local recurrence. Gupta et al. analyzed dose distributions in 78 patients who received EPP followed by conventional radiotherapy with photons and electrons [Citation9]. They identified 15 patients who had developed locoregional failure without any distant metastasis. Ten of the 15 patients failed in regions of dose inhomogeneity with the conventional method. They concluded that IMRT could offer benefits to such patients with better target coverage. Routine use of FDG-PET before the IMRT planning and SIB to high-risk regions might also contribute to the local control. FDG-PET plays important roles in post-treatment surveillance and radiotherapy planning for MPM [Citation10]. Fodor et al. investigated usefulness of FDG-PET in IMRT for unresectable MPM [Citation11]. They prescribed 56 Gy to the whole pleura with or without SIB of 62.5 Gy to the FDG-avid areas. A significant impact of SIB on local relapse after IMRT was observed (1-year local relapse-free rate, 16% without SIB vs 81% with SIB). The present study applied SIB of 61.6 Gy to FDG-avid areas, in addition to SIB of 56 Gy to high-risk regions which was determined by surgeons. The low incidence of local recurrence in the present study was among the best of reports [Citation12–17] on IMRT combined with EPP ().
Table 4. Selected series of IMRT following EPP.
Early series of IMRT for MPM resulted in high incidence of fatal pneumonitis. Allen et al. reported six cases of fatal pneumonitis in 13 patients treated with IMRT after EPP [Citation18]. They suggested that the contralateral lung V5, V20 and mean lung dose (MLD) should be limited for prevention of fatal pneumonitis. Kristensen et al. analyzed dose-volume metrics of 26 patients including 4 patients with Grade 5 lung toxicity [Citation19]. They found significant differences in MLD and V10 between patients with fatal lung toxicity and those without the toxicity. Rice et al. reported dose-dependency in fatal pulmonary toxicities [Citation20]. They concluded V20 of the contralateral lung was the independent determinant for risk of pulmonary-related death after IMRT for MPM. Chi et al. reviewed publications on IMRT after EPP for MPM, and suggested dose constraints for the contralateral lung of MLD <8.5 Gy, V5 <60% and V20 < 20% [Citation21]. Based on these reports, we set dose constraints as shown in . No fatal pulmonary toxicity was observed with this approach, and the occurrence rate of severe pulmonary toxicity was comparable with other publications ().
Severe late toxicities were observed in a long-term survivor. Among three patients who have survived for more than 5 years since IMRT, one patient developed heart-related toxicities including congestive liver dysfunction and cardiogenic brain embolism. New IMRT techniques including helical tomotherapy [Citation22] and VMAT [Citation23,Citation24] have a potential to improve dose distributions for target lesions and organs at risk. Even with such good dose distributions, some risks for severe toxicities still remain. It is important to inform probabilities of the severe toxicities to patients before the treatment.
The excellent local control and low incidence of pulmonary toxicity did not always lead to survival benefit in patients of the present study. High incidence of distant metastasis and its lethality could explain for such unsatisfying survival. Distant metastasis occurred frequently (15 of the 21 patients) and early after the treatment (median time of 8.6 months). Thirteen of the 15 patients died at a median of 2.0 months after development of the distant metastasis. The MARS trial was a randomized feasibility study which compared EPP followed by radiotherapy with non-EPP treatment [Citation25]. Overall survival in the EPP group tended to be worse than that in the non-EPP, due to high morbidity of EPP. The SAKK 17/04 study was a randomized phase 2 study evaluating efficacy and safety of hemithoracic radiotherapy for MPM after EPP [Citation26]. Locoregional relapse-free survival from surgery was not significantly different between the no-radiotherapy and the radiotherapy groups (median, 7.6 and 9.4 months, respectively). They concluded that the routine use of hemithoracic radiotherapy combined with EPP was not supported. Considering results from these studies, radical local treatment does not offer benefit to all MPM patients. Gomez et al. suggested patients with epithelioid histology and N0–1 stage could be candidate for EPP + IMRT because OS was significantly better in such patients [Citation15]. Indeed, our study also showed significantly better OS in patients with N0–1 stage, who might gain survival benefit from the IMRT. We need to limit application of IMRT combined with EPP to selected patients who have low risk of distant metastasis.
The present study has a limitation of selection bias because we evaluated only patients who were treated with IMRT, not whole MPM patients. We have no data on how many patients were canceled to receive IMRT before registration to the study. In the SAKK 17/04 study, one third of the registered 151 patients were not eligible to the radiotherapy part, and additional one third was excluded before the randomization [Citation26]. Consequently, only 54 patients were randomly assigned to the hemithoracic radiotherapy or none. In the Japan Mesothelioma Interest Group 0601 Trial, 19 of 42 registered patients (45%) could receive radiotherapy following induction chemotherapy and EPP [Citation27]. These results indicated that treatment burden of EPP was not negligible, and treatment approach for MPM should be optimized.
In conclusion, IMRT following EPP achieved excellent local control for MPM, that might lead to the long-term survival in selected patients. However, treatment burden including acute and late toxicities should be considered in this treatment approach.
Disclosure statement
The authors report no conflicts of interest. The authors alone are responsible for the content and writing of this article.
Additional information
Funding
References
- Japan Ministry of Health Labour and Welfare [Internet]. Trends in the number of deaths from mesothelioma by prefecture (21 major cities regrouped), based on Vital Statistics of Japan. 2015 [cited 2015 Dec 19]. Available from: http://www.mhlw.go.jp/toukei/saikin/hw/jinkou/tokusyu/chuuhisyu14/index.html. Japanese.
- Robinson BM. Malignant pleural mesothelioma: an epidemiological perspective. Ann Cardiothorac Surg. 2012;1:491–496.
- Robinson BWS, Musk AW, Lake RA. Malignant mesothelioma. Lancet. 2005;366:397–408.
- Okubo K, Sonobe M, Fujinaga T, et al. Survival and relapse pattern after trimodality therapy for malignant pleural mesothelioma. Gen Thorac Cardiovasc Surg. 2009;57:585–590.
- Yajnik S, Rosenzweig KE, Mychalczak B, et al. Hemithoracic radiation after extrapleural pneumonectomy for malignant pleural mesothelioma. Int J Radiat Oncol Biol Phys. 2003;56:1319–1326.
- Ahamad A, Stevens CW, Smythe WR, et al. Intensity-modulated radiation therapy: a novel approach to the management of malignant pleural mesothelioma. Int J Radiat Oncol Biol Phys. 2003;55:768–775.
- Allen AM, Schofield D, Hacker F, et al. Restricted field IMRT dramatically enhances IMRT planning for mesothelioma. Int J Radiat Oncol Biol Phys. 2007;69:1587–1592.
- Schemper M, Smith TL. A note on quantifying follow-up in studies of failure time. Control Clin Trials. 1996;17:343–346.
- Gupta V, Krug LM, Laser B, et al. Patterns of local and nodal failure in malignant pleural mesothelioma after extrapleural pneumonectomy and photon-electron radiotherapy. J Thorac Oncol. 2009;4:746–750.
- Basu S, Saboury B, Torigian DA, et al. Current evidence base of FDG-PET/CT imaging in the clinical management of malignant pleural mesothelioma: emerging significance of image segmentation and global disease assessment. Mol Imaging Biol. 2011;13:801–811.
- Fodor A, Fiorino C, Dell’oca I, et al. PET-guided dose escalation tomotherapy in malignant pleural mesothelioma. Strahlenther Onkol. 2011;187:736–743.
- Sylvestre A, Mahé M-A, Lisbona A, et al. Mesothelioma at era of helical tomotherapy: results of two institutions in combining chemotherapy, surgery and radiotherapy. Lung Cancer. 2011;74:486–491.
- Tonoli S, Vitali P, Scotti V, et al. Adjuvant radiotherapy after extrapleural pneumonectomy for mesothelioma. Prospective analysis of a multi-institutional series. Radiother Oncol. 2011;101:311–315.
- Patel PR, Yoo S, Broadwater G, et al. Effect of increasing experience on dosimetric and clinical outcomes in the management of malignant pleural mesothelioma with intensity-modulated radiation therapy. Int J Radiat Oncol Biol Phys. 2012;83:362–368.
- Gomez DR, Hong DS, Allen PK, et al. Patterns of failure, toxicity, and survival after extrapleural pneumonectomy and hemithoracic intensity-modulated radiation therapy for malignant pleural mesothelioma. J Thorac Oncol. 2013;8:238–245.
- Kimura T, Doi Y. Nakashima T. Clinical experience of volumetric modulated arc therapy for malignant pleural mesothelioma after extrapleural pneumonectomy. J Radiat Res. 2015;56:315–324.
- Thieke C, Nicolay NH, Sterzing F, et al. Long-term results in malignant pleural mesothelioma treated with neoadjuvant chemotherapy, extrapleural pneumonectomy and intensity-modulated radiotherapy. Radiat Oncol. 2015;10:267.
- Allen AM, Czerminska M, Jänne PA, et al. Fatal pneumonitis associated with intensity-modulated radiation therapy for mesothelioma. Int J Radiat Oncol Biol Phys. 2006;65:640–645.
- Kristensen CA, Nøttrup TJ, Berthelsen AK, et al. Pulmonary toxicity following IMRT after extrapleural pneumonectomy for malignant pleural mesothelioma. Radiother Oncol. 2009;92:96–99.
- Rice DC, Smythe WR, Liao Z, et al. Dose-dependent pulmonary toxicity after postoperative intensity-modulated radiotherapy for malignant pleural mesothelioma. Int J Radiat Oncol Biol Phys. 2007;69:350–357.
- Chi A, Liao Z, Nguyen NP, et al. Intensity-modulated radiotherapy after extrapleural pneumonectomy in the combined-modality treatment of malignant pleural mesothelioma. J Thorac Oncol. 2011;6:1132–1141.
- Sterzing F, Sroka-Perez G, Schubert K, et al. Evaluating target coverage and normal tissue sparing in the adjuvant radiotherapy of malignant pleural mesothelioma: helical tomotherapy compared with step-and-shoot IMRT. Radiother Oncol. 2008;86:251–257.
- Scorsetti M, Bignardi M, Clivio A, et al. Volumetric modulation arc radiotherapy compared with static gantry intensity-modulated radiotherapy for malignant pleural mesothelioma tumor: a feasibility study. Int J Radiat Oncol Biol Phys. 2010;77:942–949.
- Krayenbuehl J, Riesterer O, Graydon S, et al. Intensity-modulated radiotherapy and volumetric-modulated arc therapy for malignant pleural mesothelioma after extrapleural pleuropneumonectomy. J Appl Clin Med Phys. 2013;14:4130.
- Treasure T, Lang-Lazdunski L, Waller D, et al. Extra-pleural pneumonectomy versus no extra-pleural pneumonectomy for patients with malignant pleural mesothelioma: clinical outcomes of the Mesothelioma and Radical Surgery (MARS) randomised feasibility study. Lancet Oncol. 2011;12:763–772.
- Stahel RA, Riesterer O, Xyrafas A, et al. Neoadjuvant chemotherapy and extrapleural pneumonectomy of malignant pleural mesothelioma with or without hemithoracic radiotherapy (SAKK 17/04): a randomised, international, multicentre phase 2 trial. Lancet Oncol. 2015;16:1651–1658.
- Hasegawa S, Okada M, Tanaka F, et al. Trimodality strategy for treating malignant pleural mesothelioma: results of a feasibility study of induction pemetrexed plus cisplatin followed by extrapleural pneumonectomy and postoperative hemithoracic radiation (Japan Mesothelioma Interest Group 0601. Int J Clin Oncol. 2016;21:523–530.