Introduction
Low-grade gliomas (LGGs) are the most common pediatric central nervous system tumors, comprising 35% of new diagnoses [Citation1]. They can arise in any intracranial site and affect children of all ages. Surgery consisting of maximal safe excision is typically the mainstay of treatment for resectable tumors. However, when these tumors arise in central locations less amenable to resection due to unacceptable morbidity, such as the brainstem or optic pathway, a multi-modality approach including adjuvant therapies like chemotherapy and radiotherapy are often employed. The decision to incorporate radiotherapy in the treatment plan may be influenced by numerous variables, particularly the age of the child, extent of resection, risk of significant neurologic morbidity in the event of tumor progression, and presenting symptoms [Citation2,Citation3]. The debate regarding the use of radiotherapy stems from the concern of late effects in survivors, including neurocognitive deficits, hearing loss, vasculopathy, endocrinopathies and second malignancies [Citation4–8]. As therapeutic techniques have improved, some studies have reported overall survival rates as high as 94–100% at 10 years [Citation3,Citation9–13]. Thus, there has been significant focus on employing advanced radiotherapeutic techniques and reducing target volumes to reduce late treatment toxicity.
Modern technologies, including better immobilization techniques, high-resolution computed tomography (CT), magnetic resonance imaging (MRI), image guidance, fractionated stereotactic radiation, and proton radiation therapy offer a decrease in set-up errors and increasingly favorable dosimetry [Citation9,Citation11,Citation12,Citation14,Citation15]. In an effort to spare normal brain, clinical target volume expansions (CTV) have decreased from 10 mm to 5 mm on the most recent Children’s Oncology Group trials [Citation10]. We have adopted a similar strategy by using a 5-mm CTV expansion for pediatric LGG.
The reduction in margins prompted further studies to examine potential interfractional variation in central nervous system tumors. Measurements of interfractional variation and set-up error have been investigated in ependymomas, demonstrating that the planning target volume (PTV) and CTV margins can be safely decreased with image guidance [Citation16]. Meanwhile, assessment of craniopharyngiomas has revealed the necessity for regular tumor cyst monitoring with MRI and adaptive replanning to account for clinically significant cyst growth during the treatment course [Citation17,Citation18]. Given that low-grade gliomas also often contain mixed solid and cystic components, there can similarly be concern for tumor cyst expansion during treatment, and mid-treatment MRI is often performed during the course of radiation treatment to monitor potential tumor enlargement [Citation5,Citation10].
The purpose of this study was to report our experience with mid-treatment MRI in the treatment of pediatric intracranial LGG with proton therapy with regard to the incidence of clinically significant tumor growth during treatment requiring target adjustment and adaptive replanning.
Material and methods
Patient selection
Under an institutional review board-approved study, the medical records of 82 patients ≤18-year-old with a diagnosis of localized LGG treated at our institution between March 2007 and September 2013 were reviewed. Four patients were excluded owing to a spinal primary site, and 23 intracranial LGG patients were excluded as they had not undergone mid-treatment MRI. Patients treated for intracranial disease with evaluable mid-treatment MRI images were selected for further evaluation. Patients’ diagnoses were made by a surgical pathologist except in cases where there was an elevated risk of morbidity due to tumor location, in which case the diagnosis of LGG was rendered on radiographic features.
Radiation planning and treatment
Patients were treated with a three-dimensional conformal passively scattered proton radiation therapy to a total dose between 50.4 Gy (RBE) and 54 Gy (RBE) at 1.8 Gy (RBE) per daily fraction over 5 to 5.5 weeks. Treatment planning included simulation with CT, followed by rigid registration between the simulation CT scans and diagnostic MRI to assist in the delineation of targets. All patients had a defined gross tumor volume (GTV) or tumor bed, CTV and PTV. The CTV was created with a 5-mm expansion of the GTV in 53 patients and an 8-mm expansion of the GTV in 2 patients treated. The CTV was modified with respect to anatomical boundaries. Daily image guidance was used in all cases to achieve a PTV margin of 3 mm. The distal and proximal margins were based on the CTV while the aperture margins were based on the PTV. Customized apertures and compensators were created for each treatment field. Proton therapy treatment plans were generated in the Eclipse™ Treatment Planning System (Varian Medical Systems, Palo Alto, CA, USA). Standard institutional planning goals dictated that the PTV be encompassed by the 95% isodose line and the CTV by the 99% isodose line.
Mid-treatment MRI
Each patient completed a mid-treatment MRI at the approximate midway point in their treatment course. The mid-treatment MRIs were acquired with a low-field 0.23-Tesla MRI (Panorama 0.23T open MRI, Philips, Andover, MA, USA). Images acquired included fast field echo (FFE) and balanced-FFE (B-FFE) sequences, comparable to a T1 non-enhanced and T2 diagnostic MRI sequence with a significantly shorter acquisition time. The images were co-registered to the initial CT simulation planning scan with rigid registration using MIMVISTA™ (Mimvista Corp., Cleveland, OH, USA). Initial and mid-treatment GTV were volumetrically computed in milliliters (cm3). The delineated GTV on the mid-treatment MRI was visually compared to the initial GTV and CTV, and a clinically significant increase in size was scored if the mid-treatment GTV touched the original CTV. If the mid-treatment GTV extended to the CTV, immediate adaptive replanning was performed.
Analysis
The crude incidence of the clinically significant increase in GTV requiring adaptive replanning was calculated.
Results
We evaluated the medical records of 55 patients treated for localized intracranial LGG who underwent a mid-treatment MRI. The median age at the time of diagnosis was 6.6 years (range, 0.38–17.6 years). Of the 55 patients, 25 were female and 30 were male. At the time of radiation treatment, 27 patients had undergone at least 1 prior surgical resection; 25 patients had received at least 1 line of chemotherapy, with 15 patients having received more than 2 lines of chemotherapy; 9 patients underwent both prior surgical resection and chemotherapy. The median time from diagnosis to initiation of radiation was 2.46 years (range, 0.03–8.46 years) at a median age of 9.9 years (range, 4.4–18.8 years). Twelve patients were treated with primary radiotherapy while 43 patients received adjuvant or salvage treatment for residual or recurrent disease. Tumor locations were optic pathway/hypothalamus (n = 25), cerebrum (n = 7), thalamus (n = 6), cerebellum (n = 6), brainstem (n = 4) and tectal region (n = 7). The histologic subtypes of LGG included juvenile pilocytic astrocytoma, pilomyxoid astrocytoma, ganglioglioma and oligodendroglioma. Patient, tumor and prior treatment characteristics are summarized in .
Table 1. Patient characteristics.
The median proton therapy prescription dose delivered was 54 Gy (RBE) (range, 50.4–54 Gy [RBE]). The initial median tumor volume was 26.1 ml (range, 1–361 ml), which was the same as the minimum, median and maximum mid-treatment tumor volumes. Fifty-four of the 55 patients did not exhibit a clinically significant change in tumor volume requiring adaptive replanning.
One patient required adaptive replanning secondary to significant tumor growth in both its solid and cystic components extending beyond the initial CTV. The initial GTV was 103.2 ml, and increased by 50% mid-treatment to 155.1 ml. The patient was diagnosed with a hypothalamic juvenile pilocytic astrocytoma before 1 year of age. He was initially treated with a subtotal resection owing to the tumor’s proximity to the optic chiasm as well as adjuvant chemotherapy with vincristine and carboplatin. His tumor progressed 6 years after initial treatment. He then underwent another subtotal resection, with pathology again demonstrating pilocytic astrocytoma, followed by systemic chemotherapy. Due to disease progression, he received an additional 5 lines of chemotherapy including the following agents: vinblastine, cyclophosphamide, temozolamide, etoposide, bevacizumab, irinotecan, lenolidomide and vorinostat. After the 6th line of chemotherapy, the patient became steroid-dependent owing to nausea and intracranial progression. He was then referred for radiation consultation. Pretreatment MRI demonstrated rapid growth when compared to imaging obtained one month before the radiation consultation. Radiation was ultimately initiated 7.2 years after the initial diagnosis ().
Figure 1. (A) Sagittal view of a computed tomography simulation scan with pretreatment gross tumor volume (GTV; red, inside line) and the 5-mm clinical target volume (CTV) expansion (yellow, outside line). (B) Mid-treatment balanced fast field-echo MRI with the pretreatment GTV (red, inside line), pretreatment 5-mm CTV expansion (yellow, middle line), and mid-treatment GTV contour (green, outside line) extending beyond the pretreatment CTV.
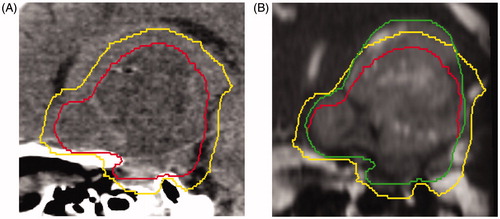
Discussion
Pediatric LGG represents a heterogeneous set of tumors of different histologic subtypes for which radiotherapy is often indicated, either as primary treatment at the time of diagnosis or following progression after prior treatment including surgery, chemotherapy or both. The decision to initiate radiotherapy is often influenced by the fear of late effects, such as a potential impact on growth and development, neurocognitive decline, endocrine deficiencies, hearing loss, cerebral vasculopathy and secondary malignancies. Since the advent of improved diagnostic imaging to define targets and the introduction of highly conformal radiation therapy techniques, the focus has been on reducing target volumes with the aim of minimizing late effects while maintaining a high rate of disease control. However, shrinking target volumes and highly conformal radiotherapy are less forgiving and may be more susceptible to a geographical miss should there be tumor changes during the treatment course. Therefore, greater vigilance and surveillance of target volume changes and adaptive replanning may be necessary.
Craniopharyngioma is a pediatric brain tumor whose volume is prone to change during radiation therapy and for which surveillance and adaptive replanning has become essential with the decrease in target expansion. For instance, Winkfield et al. [Citation18] evaluated the effect of decreasing CTV expansion from 10 mm to 5 mm on craniopharyngiomas treated with proton beam radiation. MRIs were obtained during weeks 1, 3 and 4 of radiation treatment for 17 pediatric patients. In this series, 35% of patients required adaptive replanning or cyst drainage during or after treatment, and 24% of patients experienced tumor growth outside of the original treatment field, requiring adaptive replanning to ensure appropriate coverage of the target volume.
Similarly, Beltran et al. [Citation17] reported the observed dosimetric effect of target expansion and setup uncertainty during radiation therapy in 15 pediatric patients treated for craniopharyngioma. MRI was performed before and a median of six times during treatment to assess changes in the tumor volume. The patients were treated with three-dimensional conformal radiotherapy plans; however, highly conformal intensity-modulated radiation therapy comparison plans were generated and the role of adaptive therapy was assessed as a function of GTV changes during treatment. The mean of the maximal growth change in the GTV was 28.5% [range, –20.7% to 82%], again demonstrating that the GTV of pediatric craniopharyngioma patients changes size during therapy, for which adaptive replanning of the conformal plans is critical.
In a phase II prospective trial, Merchant et al. [Citation10] reported outcomes on 78 pediatric patients with LGG treated with conformal radiation therapy and a 10-mm CTV margin. With a median follow-up of 89 months, 13 patients experienced disease progression. One patient developed a marginal recurrence, with 8 patients experiencing local recurrences and 4 patients experiencing metastatic failure. The mean and standard error 5- and 10-year overall survival rates were 98.5% ± 1.6% and 95.9% ± 5.8%, respectively. On-treatment MRI imaging at weeks 3 and 5 of radiotherapy demonstrated active cyst expansion in 19 (24%) of 78 patients, suggesting that changes in the PTV for LGG during treatment are common. Two patients had a clinically significant increase in target volume requiring modification of their treatment plans.
In our series of 55 pediatric patients treated with proton therapy for localized intracranial LGG, only one patient (1.8%) experienced an increase in GTV that was noted on a mid-treatment MRI, which was also deemed to be clinically significant, requiring adaptive replanning. Although the number of events (n = 1) were too small to permit further statistical analysis to determine predictive clinicopathologic factors, some notable features of this case include tumor progression through 6 lines of chemotherapy and evidence of rapid tumor growth on a pretreatment diagnostic MRI performed 1 month before patient evaluation for radiotherapy. The interval between consultation and the initiation of radiotherapy was 14 days, within the standard of care. Therefore, it would appear that routine MRI during radiotherapy for pediatric intracranial LGG is not warranted; however, increased vigilance and MRI surveillance may be warranted for tumors with significant cystic components and for tumors that exhibit rapid tumor progression immediately preceding the initiation of radiotherapy. In such cases, increases in GTV during treatment may be clinically significant and adaptive replanning may be critical to ensure adequate radiation dose coverage and minimize the risk of a geographical tumor miss leading to an increased risk of tumor progression. This strategy is particularly important in proton therapy patients given the rapid dose falloff beyond the target.
Conclusions
This study evaluates the utility of mid-treatment MRI in pediatric patients treated for LGG with proton beam therapy. We demonstrate that the incidence of clinically significant target volume change during treatment is very low (1.8%). Routine mid-treatment MRIs in this patient population may not be clinically warranted but should be considered for patients whose tumors have a significant cystic component or for those experiencing rapid tumor progression on diagnostic imaging immediately preceding the initiation of radiotherapy.
Disclosure statement
The authors report no conflicts of interest.
References
- Ward E, DeSantis C, Robbins A, et al. Childhood and adolescent cancer statistics, 2014. CA Cancer J Clin. 2014;64:83–103.
- Watson GA, Kadota RP, Wisoff JH. Multidisciplinary management of pediatric low-grade gliomas. Semin Radiat Oncol. 2001;11:152–162.
- Oh KS, Hung J, Robertson PL, et al. Outcomes of multidisciplinary management in pediatric low-grade gliomas. Int J Radiat Oncol Biol Phys. 2011;81:e481–e488.
- Brower JV, Indelicato DJ, Aldana PR, et al. A treatment planning comparison of highly conformal radiation therapy for pediatric low-grade brainstem gliomas. Acta Oncol. 2013;52:594–599.
- Greenberger BA, Pulsifer MB, Ebb DH, et al. Clinical outcomes and late endocrine, neurocognitive, and visual profiles of proton radiation for pediatric low-grade gliomas. Int J Radiat Oncol Biol Phys. 2014;89:1060–1068.
- Hua C, Bass JK, Khan R, et al. Hearing loss after radiotherapy for pediatric brain tumors: effect of cochlear dose. Int J Radiat Oncol Biol Phys. 2008;72:892–899.
- Merchant TE, Conklin HM, Wu S, et al. Late effects of conformal radiation therapy for pediatric patients with low-grade glioma: prospective evaluation of cognitive, endocrine, and hearing deficits. JCO. 2009;27:3691–3697.
- Grill J, Couanet D, Cappelli C, et al. Radiation-induced cerebral vasculopathy in children with neurofibromatosis and optic pathway glioma. Ann Neurol. 1999;45:393–396.
- Marcus KJ, Goumnerova L, Billett AL, et al. Stereotactic radiotherapy for localized low-grade gliomas in children: final results of a prospective trial. Int J Radiat Oncol Biol Phys. 2005;61:374–379.
- Merchant TE, Kun LE, Wu S, et al. Phase II trial of conformal radiation therapy for pediatric low-grade glioma. J Clin Oncol. 2009;27:3598–3604.
- Saran FH, Baumert BG, Khoo VS, et al. Stereotactically guided conformal radiotherapy for progressive low-grade gliomas of childhood. Int J Radiat Oncol Biol Phys. 2002;53:43–51.
- Hug EB, Muenter MW, Archambeau JO, et al. Conformal proton radiation therapy for pediatric low-grade astrocytomas. Strahlenther Onkol. 2002;178:10–17.
- Raikar SS, Halloran DR, Elliot M, et al. Outcomes of pediatric low-grade gliomas treated with radiation therapy: a single-institution study. J Pediatr Hematol Oncol.2014;36:e366–e370.
- Merchant TE, Hua CH, Shukla H, et al. Proton versus photon radiotherapy for common pediatric brain tumors: comparison of models of dose characteristics and their relationship to cognitive function. Pediatr Blood Cancer. 2008;51:110–117.
- Beltran C, Trussell J, Merchant TE. Dosimetric impact of intrafractional patient motion in pediatric brain tumor patients. Med Dosim. 2010;35:43–48.
- Beltran C, Naik M, Merchant TE. Dosimetric effect of setup motion and target volume margin reduction in pediatric ependymoma. Radiother Oncol. 2010;96:216–222.
- Beltran C, Naik M, Merchant TE. Dosimetric effect of target expansion and setup uncertainty during radiation therapy in pediatric craniopharyngioma. Radiother Oncol. 2010;97:399–403.
- Winkfield KM, Linsenmeier C, Yock TI, et al. Surveillance of craniopharyngioma cyst growth in children treated with proton radiotherapy. Int J Radiat Oncol Biol Phys. 2009;73:716–721.