Introduction
Existing evidence shows that biochemical control rates are improved if the delivered dose to the prostate gland is increased [Citation1]. Brachytherapy (BT) proved to be an effective and safe way for dose escalation in combination with external-beam radiotherapy (EBRT) [Citation1–6].
The main rationale of using magnetic resonance imaging (MRI) across prostate BT workflow was the superior prostate gland and organ at risk (OAR) definition compared to transrectal ultrasound (TRUS). Furthermore, multi-parametric MRI (spectroscopy, dynamic contrast-enhanced-, diffusion weighted images) provides the possibility to visualize the highest risk of local relapse (i.e., intraprostatic tumor), allowing focal dose escalation that might improve clinical outcome [Citation7–12].
The clinical efficacy of MR-guided BT has been well demonstrated in gynecologic tumors [Citation13,Citation14], however, only few long-term clinical results are available for MR-guided high-dose-rate prostate BT (HDRBT) [Citation12,Citation15–21]. We introduced MR-only prostate HDRBT boost in 2007 [Citation18,Citation19] and now we report the five-year clinical efficacy, late toxicity and quality of life (QoL).
Material and methods
From October 2007 to September 2015, 52 patients with intermediate- to high-risk prostate cancer (National Comprehensive Cancer Network criteria, IRPC, HRPC) were treated with 46–60 Gray (Gy) of three-dimensional conformal EBRT preceded and/or followed by a single dose of 8–10 Gy HDRBT. Almost all patients (96%) received androgen deprivation therapy and all HRPC patients received pelvic irradiation. Staging examinations included prostate specific antigen (PSA) measurement, digital rectal examination, histopathology review, 1.5 T prostate MRI and bone scan in HRPC. Exclusion criteria were contraindication for epidural anesthesia or MRI, transurethral resection of the prostate (TURP) within 6 months, large TURP defect, high (>18) International Prostate Symptom Score (IPSS), maximum uroflow rate <10 ml/s, prostate volume >60 cm3 or presence of pubic arch interference. The prospective study protocol was approved by the national ethical committee (reference number: 84-261/2008-1018EKU). Patient-, tumor- and treatment characteristics are presented in Supplementary Tables 1 and 2.
Table 1. Disease control: actuarial and crude rates for all patients.
Table 2. Frequency of late toxicities for all patients with baseline values.
Interventions were performed in a 0.35 T MRI scanner (Signa Ovation; General Electric Healthcare, Milwaukee, WI, USA), initially in the right lateral decubitus position using the manufacturer’s general purpose surface coil for imaging. From 2009, patients were placed in supine position in combination with a pelvic coil, allowing more comfortable interventions (Supplementary Figure 1). Navigation, contouring and planning were exclusively based on MR images. Details of the procedure have been previously described [Citation19]. The prostate was implanted via a peripheral loading technique with extra effort to insert catheters directly into any visualized tumor or tumor-bearing region(s) previously defined on diagnostic MRI. Clinical target volume (CTV) included the prostate gland ± extraprostatic extension or root of seminal vesicles without margin. The urethra and pre-urethral strip of the central gland (if no tumor present) were excluded from CTV (Supplementary Figure 2) [Citation19]. Anterior rectal wall, intraprostatic-, membranous urethra and bladder were delineated as OARs. Dose-point optimization (n = 7, Theraplan Plus vs.3.8; MDS Nordion, Quebec, Canada) and inverse planning optimization were performed (n = 45, Brachyvision vs.8.1-10; Varian Medical Systems, Palo Alto, CA, USA). The initial planning aim was 8 Gy, which migrated to 9–10 Gy during the course of the study providing a total (EBRT + BT) 2 Gy-biological equivalent doses of 81.7–97.4 Gy assuming an α/β = 1.5 (Supplementary Table 2). Where it was possible, we tried to maximally cover high-risk areas and concentrate the hot spots within this site (Supplementary Figure 2). Treatment was delivered using multichannel HDR afterloading system (Gammamed plus, Varian, Palo Alto, CA, USA) with in vivo rectal dosimetry.
Figure 2. Changes in GI/GU EPIC domain scores from baseline over time. EPIC = Expanded Prostate Cancer Index Composite. Arrows indicate significant (p < .05) changes.
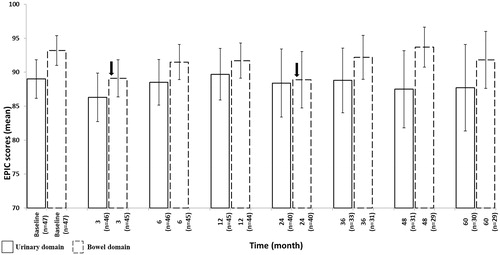
Patients were seen by a radiation oncologist weekly during RT. The follow up schedule included PSA test every three months in the first year, every six months in the second to fifth year, and yearly thereafter. Late morbidity was scored using the Common Terminology Criteria for Adverse Events (CTCAE 4.0) combined with RTOG (Radiation Therapy Oncology Group) scale for dysuria, while urinary and bowel QoL were measured by the Expanded Prostate Cancer Index Composite (EPIC) with IPSS at baseline and at regular intervals up to five years.
Crude and five/seven-year actuarial rates for biochemical relapse-free survival (bRFS, Phoenix definition: PSA nadir +2 ng/ml), local relapse-free survival (LRFS), distant metastasis-free survival (DMFS), cancer-specific survival (CSS) and overall survival (OS) were calculated and described by the Kaplan–Meier method. OS/CSS was defined as the period from end of treatment to date of any death/death by PC. bRFS, LRFS and DMFS were defined as the interval from end of treatment to biochemical, local and distant failure. Changes in EPIC scores relative to baseline were assessed by Paired-Samples t-test, with p < .05 considered as significant. Statistical evaluation was performed with SPSSvs.20 (Chicago, IL, USA).
Results
After a median follow-up of 73 (13–103) months, the overall five-year crude/actuarial rates of bRFS were 92/97%, respectively (, ). Four biochemical relapses occurred exclusively in the HRPC group after 48-, 66-, 78- and 90 months, providing a five-year crude/actuarial biochemical control of 87/90%. Two patients developed subsequently bone metastasis, while two patients have still no evident of clinical disease. One IRPC patient had suspicious tumor propagation on the bladder trigonum after 93 month follow-up. Due to poor general condition (ECOG:4, previous stroke), no PSA test, imaging studies, biopsy was performed, patient went on best supportive care and recorded as a local failure. Two patients (stage T3a,b PC, one refused ADT) developed bone metastasis without local- or other distant failure. The crude/5-year actuarial DMFS was 96/97%, respectively. Forty-seven patients (92%) were alive at last follow up. No PC-related death was recorded, three patients died due to second primary tumor (lung, head and neck, colorectal), and one due to cardiovascular disease, providing a crude and five-year CSS and OS of 100/100% and 92/91%, respectively.
The main late urinary toxicity was dysuria (Grade (Gr) 2 in 24/52 cases), including nine patients using alfa blockers at baseline (). There was a single urinary incontinence (Gr.1) in a patient with past TURP. Three urinary strictures were diagnosed, including one Gr.3 event at the membranous urethra after 23 months providing a five-year actuarial rate of 2.6%. There was no ≥ Gr.3 late GI toxicity. Gr.1 diarrhea (10/51), Gr.1 urgency (9/51), Gr.2 proctitis with Gr.2 bleeding (1/52) (completely resolved after Salazopyrin) and Gr.2 fecal incontinence (1/52) represented the maximum late GI toxicities.
Quality of life data were available for 47/52 (90%) patients. EPIC urinary scores recovered within 6–12 months, followed by a slight decline and stabilized from 4th-year. After a significant decline (p = .005), bowel functions returned to baseline after 3 months and apart from a transient drop at 24 months (p = .04) continued to be around the baseline level ().
Dosimetry results, imaging observations and working time analysis are attached in Supplementary appendix.
Discussion
To date only few long-term clinical results are available on MR guided-HDRBT [Citation12,Citation13,Citation15–17] as reports primarily focus on clinical workflow, feasibility and cohort sizes, follow-up times are too short to address the clinical efficacy. We aimed to report a five-year clinical outcome with MRI-only HDRBT boost for IRPC and HRPC patients. Overall, five-year bRFS, LRFS DMFS and OS of 97.4%, 100%, 96.2% and 91.2% were achieved, which are comparable to combined EBRT + HDR literature with TRUS and MR guidance, including partial gland boost in low-field MR system [Citation1–6,Citation15,Citation16,Citation21]. Our moderate dose escalation protocol with single implants follows the national and Catalan practice [Citation2,Citation6].
Generally, a mild long-term GU/GI toxicity was observed. The dominant urinary symptom was dysuria as 17% of the patient developed transitory or permanently alpha blocker dependence. A low Gr.3 stricture rate of 2.6% was observed, which compares favorably to published results of 3–12% [Citation1–6,Citation22,Citation23]. Despite the lack of clear correlation between dose-volume, non-dosimetry factors and stricture incidence [Citation23,Citation24], we believe that MR-based definition of membranous urethra might improve dose delivery and eventually decrease toxicity. GI toxicity was generally mild with only one Gr.2 proctitis. The observed Gr.1 diarrheas/urgencies and fecal incontinence mainly attributed to EBRT.
EPIC scores revealed mainly urinary baseline symptoms and showed transient changes in both domains over time similarly to literature findings [Citation21,Citation22,Citation25]. Apart from a post-treatment decline, we noted a transient drop in GI QoL at 24 months as well. The main reason is the patient with manifested proctitis suffered from a bowel perforation during the explorative colonoscopy. He reported an almost 50% decrease in total GI score compared to baseline. It should be noted that treatment-induced alpha blocker dependence could mask urinary QoL and despite a questionnaire return rate of 90%, some sub-score questions were incomplete.
The limited number of cases with heterogeneous radiation treatment across the cohort represents the main limitation of our paper. However, the follow-up is long enough to draw a definitive conclusion on clinical outcome. It would be excessive and premature to state that these results are directly related to MRI guidance as several patients – tumor – and treatment related factors may contribute to clinical outcome [Citation26]. Although, its influence on targeted catheter placement, target and OARs definition throughout the course of the procedure could not be ignored either.
In conclusion, MRI-only HDR-BT boost provides excellent five-year disease control and QoL for IRPC and HRPC patients, building up further evidence for inclusion of MRI across the BT workflow.
IONC_A_1349333_Supplementary_Information.zip
Download Zip (4 MB)Acknowledgments
The authors thank Andrea Farkas for the registration of QoL scores and for the Urology and Anesthesiology team at the Kaposi Mor Teaching Hospital for their input and support.
Disclosure statement
The authors report no conflicts of interest.
Funding
There was no funding source for this study.
References
- Moon DH, Efstathiou JA, Chen RC. What is the best way to radiate the prostate in 2016? Urol Oncol Semin Orig Investig. 2016;35:1–10.
- Ágoston P, Major T, Fröhlich G, et al. Moderate dose escalation with single-fraction high-dose-rate brachytherapy boost for clinically localized intermediate- and high-risk prostate cancer: 5-year outcome of the first 100 consecutively treated patients. Brachytherapy. 2011;10:376–384.
- Hoskin PJ, Rojas AM, Bownes PJ, et al. Randomised trial of external beam radiotherapy alone or combined with high-dose-rate brachytherapy boost for localised prostate cancer. Radiother Oncol. 2012;103:217–222.
- Martínez-Monge R, Moreno M, Ciérvide R, et al. External-beam radiation therapy and high-dose rate brachytherapy combined with long-term androgen deprivation therapy in high and very high prostate cancer: preliminary data on clinical outcome. Int J Radiat Oncol Biol Phys. 2012;82:469–476.
- Sathya JR, Davis IR, Julian JA, et al. Randomized trial comparing iridium implant plus external-beam radiation therapy with external-beam radiation therapy alone in node-negative locally advanced cancer of the prostate. JCO. 2005;23:1192–1199.
- Pistis F, Guedea F, Pera J, et al. External beam radiotherapy plus high-dose-rate brachytherapy for treatment of locally advanced prostate cancer: the initial experience of the Catalan Institute of Oncology. Brachytherapy. 2010;9:15–22.
- Ahmed HU, El-Shater Bosaily A, Brown LC, et al. Diagnostic accuracy of multi-parametric MRI and TRUS biopsy in prostate cancer (PROMIS): a paired validating confirmatory study. Lancet. 2017;389:815–822.
- Simmons LAM, Kanthabalan A, Arya M, et al. The PICTURE study: diagnostic accuracy of multiparametric MRI in men requiring a repeat prostate biopsy. Br J Cancer. 2017;116:1159–1165.
- Damkjær S, Thomsen JB, Petersen SI, et al. A modeling study of functional magnetic resonance imaging to individualize target definition of seminal vesicles for external beam radiotherapy. Acta Oncol. 2017;56:799–805.
- Feng Y, Welsh D, McDonald K, et al. Identifying the dominant prostate cancer focal lesion using image analysis and planning of a simultaneous integrated stereotactic boost. Acta Oncol. 2015;54:1543–1550.
- Vigneault E, Mbodji K, Racine LG, et al. Image-guided high-dose-rate brachytherapy boost to the dominant intraprostatic lesion using multiparametric magnetic resonance imaging including spectroscopy: results of a prospective study. Brachytherapy. 2016;15:746–751.
- Gomez-Iturriaga A, Casquero F, Urresola A, et al. Dose escalation to dominant intraprostatic lesions with MRI-transrectal ultrasound fusion high-dose-rate prostate brachytherapy. Prospective phase II trial. Radiother Oncol. 2016;119:91–96.
- Lakosi F, de Cuypere M, Viet Nguyen P, et al. Clinical efficacy and toxicity of radio-chemotherapy and magnetic resonance imaging-guided brachytherapy for locally advanced cervical cancer patients: a mono-institutional experience. Acta Oncol. 2015;54:1558–1566.
- Lindegaard JC, Fokdal LU, Nielsen SK, et al. MRI-guided adaptive radiotherapy in locally advanced cervical cancer from a Nordic perspective. Acta Oncol. 2013;52:1510–1519.
- Ares C, Popowski Y, Pampallona S, et al. Hypofractionated boost with high-dose-rate brachytherapy and open magnetic resonance imaging-guided implants for locally aggressive prostate cancer: a sequential dose-escalation pilot study. Int J Radiat Oncol Biol Phys. 2009;75:656–663.
- Blanchard P, Ménard C, Frank SJ. Clinical use of magnetic resonance imaging across the prostate brachytherapy workflow. Brachytherapy. 2017. DOI:10.1016/j.brachy.2016.11.012
- Buus S, Rylander S, Hokland S, et al. Learning curve of MRI-based planning for high-dose-rate brachytherapy for prostate cancer. Brachytherapy. 2016;15:426–434.
- Lakosi F, Antal G, Vandulek C, et al. Technical feasibility of transperineal MR-guided prostate interventions in a low-field open MRI unit: Canine study. Pathol Oncol Res. 2009;15:315–322.
- Lakosi F, Antal G, Vandulek C, et al. Open MR-guided high-dose-rate (HDR) prostate brachytherapy: feasibility and initial experiences open MR-guided high-dose-rate (HDR) prostate brachytherapy. Pathol Oncol Res. 2011;17:315–324.
- Ménard C, Susil RC, Choyke P, et al. MRI-guided HDR prostate brachytherapy in standard 1.5 T scanner. Int J Radiat Oncol Biol Phys. 2004;59:1414–1423.
- Murgic J, Chung P, Berlin A, et al. Lessons learned using an MRI-only workflow during high-dose-rate brachytherapy for prostate cancer. Brachytherapy. 2016;15:147–155.
- Choudhury A, Arthur C, Malik J, et al. Patient-reported outcomes and health-related quality of life in prostate cancer treated with a single fraction of high dose rate brachytherapy combined with hypofractionated external beam radiotherapy. Clin Oncol. 2014;26:661–667.
- Díez P, Mullassery V, Dankulchai P, et al. Dosimetric analysis of urethral strictures following HDR 192Ir brachytherapy as monotherapy for intermediate- and high-risk prostate cancer. Radiother Oncol. 2014;113:410–413.
- Hindson BR, Millar JL, Matheson B. Urethral strictures following high-dose-rate brachytherapy for prostate cancer: analysis of risk factors. Brachytherapy. 2013;12:50–55.
- Hoskin PJ, Rojas AM, Ostler PJ, et al. Quality of life after radical radiotherapy for prostate cancer: longitudinal study from a randomised trial of external beam radiotherapy alone or in combination with high dose rate brachytherapy. Clin Oncol (R Coll Radiol). 2013;25:321–327.
- Hjälm-Eriksson M, Ullén A, Johansson H, et al. Comorbidity as a predictor of overall survival in prostate cancer patients treated with external beam radiotherapy combined with HDR brachytherapy boosts. Acta Oncol. 2017;56:21–26.