Abstract
Introduction: The aim of the present study was to examine the RBE for early damage in an in vivo mouse model, and the effect of the increased linear energy transfer (LET) towards the distal edge of the spread-out Bragg peak (SOBP).
Method: The lower part of the right hind limb of CDF1 mice was irradiated with single fractions of either 6 MV photons, 240 kV photons or scanning beam protons and graded doses were applied. For the proton irradiation, the leg was either placed in the middle of a 30-mm SOBP, or to assess the effect in different positions, irradiated in 4 mm intervals from the middle of the SOBP to behind the distal dose fall-off. Irradiations were performed with the same dose plan at all positions, corresponding to a dose of 31.25 Gy in the middle of the SOBP. Endpoint of the study was early skin damage of the foot, assessed by a mouse foot skin scoring system.
Results: The MDD50 values with 95% confidence intervals were 36.1 (34.2–38.1) Gy for protons in the middle of the SOBP for score 3.5. For 6 MV photons, it was 35.9 (34.5–37.5) Gy and 32.6 (30.7–34.7) Gy for 240 kV photons for score 3.5. The corresponding RBE was 1.00 (0.94–1.05), relative to 6 MV photons and 0.9 (0.85–0.97) relative to 240 kV photons. In the mice group positioned at the SOBP distal dose fall-off, 25% of the mice developed early skin damage compared with 0–8% in other groups. LETd,z = 1 was 8.4 keV/μm at the distal dose fall-off and the physical dose delivered was 7% lower than in the central SOBP position, where LETd,z =1 was 3.3 keV/μm.
Conclusions: Although there is a need to expand the current study to be able to calculate an exact enhancement ratio, an enhanced biological effect in vivo for early skin damage in the distal edge was demonstrated.
Introduction
Proton therapy as cancer treatment provide a more favorable dose distribution compared to X-rays, effectively reducing unwanted dose to healthy tissue and achieving higher conformal dose distributions [Citation1,Citation2]. However, there is still a range of unresolved radiobiological questions that need to be answered in order to fully exploit the advantages of proton therapy. While the physical characteristics of particle radiation have been the aim of intense research, less focus has been on the actual biological responses to particle irradiation.
Proton radiation produces dense energy deposits leading to an increased efficiency of cell killing [Citation3]. This efficiency is owing to an increased linear energy transfer (LET), a measure of the quality of different types of radiation [Citation3]. The concept of relative biological effectiveness (RBE) has been introduced to account for this increased efficiency. RBE is defined as the ratio of a dose from photons to a dose from any other particle to produce the same biological effect. This is complicated by the fact that RBE is not a constant, but depends, among others, on the specific tissue, on the particle energy, and on the dose [Citation4,Citation5]. Several in vitro experiments have carefully assessed the RBE of protons for a large range of cell lines, as reviewed in [Citation6,Citation7]. Increased RBE not only leads to higher tumor control probability (TCP), but also leads to increased normal tissue complication. Both early and late adverse effects are observed in normal tissue following radiation exposure. Among early effects, early skin reactions, which are mostly reversible, are often seen [Citation8].
Currently, an RBE value of 1.1 is used in clinical situations to calculate the equivalent biologic dose for proton therapy relative to photon therapy [Citation9]. However, the LET increases drastically in the last few millimeters of the particle track [Citation10], which in vitro studies have shown to translate into a higher RBE, both in terms of cell killing [Citation11–13] and DNA double strand breaks [Citation14]. There has been limited in vivo data for this issue, with a few studies demonstrating an increase in RBE towards the distal edge of the SOBP [Citation15–17]. There is an increased concern that the RBE of 1.1 is an oversimplification, due to this distal edge effect, which have shown to have clinical implications [Citation18]. To be able to comply with these issues and avoid side effects, it is crucial to have experimental biological studies to determine the extent and magnitude of these biological effects.
We have in the current study determined the RBE for early damage in an in vivo model, and observed an enlarged biologic effect with the increased LET in the distal edge of the spread-out Bragg peak.
Methods
Animal models and evaluation of radiation effects
All experiments were performed on 10- to 20-week-old female CDF1 mice, with the different ages distributed between the treatment groups. As it is the lower part of the leg and the foot that is the target, there is no age-dependent variation in diameter of that part of the leg. All experiments were performed under the Institutional and National Guidelines for Animal Welfare.
The procedure for radiation treatment has previously been described [Citation19]. Irradiations were performed locally to the right hind leg, of non-anesthetized mice restrained in a Lucite jig. The leg was fixed in the correct position for treatment was achieved by applying a small drop of histoacrylic glue to the restraining jig in the region of the uppermost part of the leg. The leg was then firmly compressed against the jig with tape for a 5-min period, after which time the tape was loosened and left for 10 min prior to any treatment. The expected response was based on the known response for X-rays for this mouse strain [Citation19,Citation20]. After treatment the leg was easily detached from the jig. For scoring the skin damage, a previous published skin score table [Citation21] was used, as described in Ref. [Citation19]. The skin damage is scored in steps of 0.5, with 3.5 being the maximum value. Mice were observed on a daily basis between 7 and 40 days following treatment, and the percentage of animals in each treatment group with a score of 2.5 (moist desquamation of 50% of the skin area) and 3.5 (moist desquamation of entire skin area) was determined.
Dose delivery
All proton treatments were performed at the clinical gantry at the Cyclotron Centre Bronowice, Institute of Nuclear Physics in Krakow, Poland, using their scanned beam. All animals were irradiated using a 3 cm wide spread-out Bragg peak, centered at 7 cm after the phantom surface. The SOBP location and the holder fixation equipment were checked using both a Markus ionization chamber, a slanted Gafchromic EBT3 film and in-room X-ray verification, and all agreed with sub-mm accuracy. The systematic uncertainty in the dose delivery is assumed to be 2.5% (95% CI). The field size was 10 × 4 cm to cover three legs simultaneously. Overall, the thickness of the mouse leg varied from 2.3 to 2.7 mm, and to calculate the average dose and LET in each position, a diameter of 2.5 mm was assumed. The mouse leg was placed vertically, so the entire target was in the same position.
Full dose response curves were obtained with the target placed in the middle of the SOBP (Position 2, ), with 9 mice per dose group. To assess the effect in different positions of the SOBP, mouse legs were irradiated using the same dose plan (31.25 Gy delivered in the middle of the SOBP) in 4 mm intervals towards the distal edge (Position 1–8, ). Groups of 12 mice were irradiated in each position. LET values at the different position are shown in .
Figure 1. Outline of setup and acute skin damage in mice irradiated in different positions of the SOBP. Depth-dose curve for 31.25 Gy dose point. Black line: Physical dose, Green curve: LETd. Position number and the dotted lines indicate the positions of the target. Percentage of mice developing acute skin damage (score 2.5) in each position are shown with red dots. N = 12 mice per group.
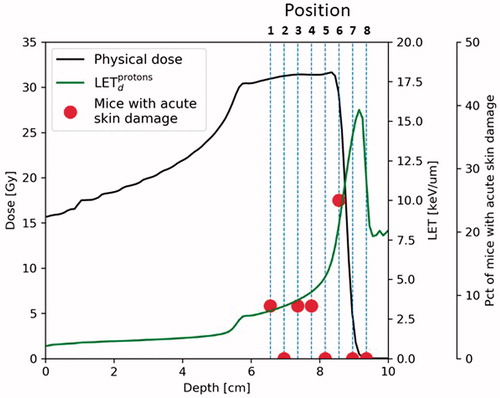
Table 1. Different positions in the SOBP, with the physical dose and calculated LET.
X-ray irradiation of the reference groups was performed using the same water phantom as for the proton irradiation, but with the target located only 2.5 cm from the phantom surface. Orthovoltage X-ray irradiation was performed at the Department of Experimental Clinical Oncology at Aarhus University Hospital, with a therapeutic x-ray machine (Philips 240 kV; 10 mA; 2-mm Al filter; 1.1 mm Cu half-value layer; dose rate 2.3 Gy/min). X-ray dose delivery was monitored with an ionization chamber (TM31010, PTW-Freiburg). MV X-ray irradiation was carried out at Department of Radiation Therapy, Aarhus University Hospital using a clinical linac (Varian Clinac 6 MV, SSD =100 cm, Dose rate at mouse target 6.4 Gy/min).
All irradiations were performed at the same time interval of the day to ensure no response differences due to the circadian rhythm of the mice.
LET values are here reported in terms of track-average (LETt) and dose-averaged LET (LETd). LET values are calculated following the algorithm C in [Citation22] for protons only (LETd,z = 1) and the full secondary particle spectrum (LETd,z ≥ 1) [Citation23] using the Monte Carlo particle Transport code SHIELD-HIT12A [Citation24].
Data analysis
Dose–response curves were fitted to data using logit analysis. RBE is calculated as the ratio of the MDD50 (dose to produce moist desquamation in 50% of mice) for photons to the MDD50 for protons.
Results
RBE in the middle of SOBP
In order to compare the effect of proton and x-ray irradiation on early (acute) skin reactions, mice legs were irradiated with a range of doses in each modality. shows the dose–response curves for early skin reaction for X-rays and protons in the middle of the SOBP (Position 2, ). Data presented is the percentage of animals in each treatment group showing a severe reaction (score 3.5, moist desquamation of entire skin area) () or a more moderate reaction (score 2.5, moist desquamation of 50% of skin area) () of the treated foot, at any time between Day 7 and 40. The MDD50 values for protons with 95% confidence interval were 36.1 (34.2–38.1) Gy for score 3.5. and 31.2 (29.4–33.2) Gy for score 2.5. As differences were expected, reference curves were produced at both 240 kV and 6 MV radiation. For 240 kV radiation the MDD50 values with 95% confidence interval were 32.6 (30.7–34.7) Gy for score 3.5 and 28.5 (27.1–29.9) Gy for score 2.5. For 6 MV radiation, the MDD50 values with 95% confidence interval were 35.9 (34.5–37.1) Gy for score 3.5. and 33.8 (31.5–34.9) Gy for score 2.5. The obtained RBE values were 0.91 and 0.90 for skin score 2.5 and 3.5, respectively, relative to 240 kV, and 1.06 and 1.00 for skin score 2.5 and 3.5, respectively, relative to 6 MV.
Figure 2. Acute skin score. Effect of proton or photon irradiation on the radiation response of normal mouse foot skin. Tissue response was assessed by the percentage of mice showing moist desquamation between 7 and 40 days after treatment. (A) Score 3.5 (moist desquamation of entire skin area). (B) Score 2.5 (moist desquamation of 50% of skin area). N = 9–12 mice per group; the error bars show 95% confidence intervals on the MDD50 values. RBE values with 95% confidence intervals.
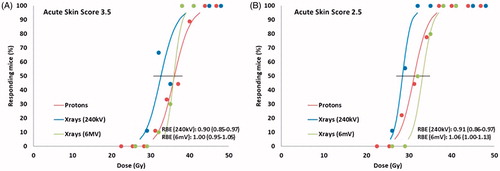
Effect in the distal part of the SOBP
To evaluate the effect of different depths in the SOBP, mice were irradiated with the same dose plan (31.25 Gy in the middle of the SOBP) in different positions with 4 mm apart. This setup was used to ensures a high enough resolution to see the changes induced by an increase of LET that occurs within very few millimeters (see outline of setup in ). Groups of 12 mice were irradiated in each position. In position 1–5, 0 or 1 mouse/group was found to have an score 2.5 early skin reaction (). An increased effect was observed in position 6, were 3/12 mice developed an early skin reaction. Position 6 is in the very distal part of the SOBP/the beginning of the distal dose drop of, and the physical dose was on average 7% lower in this position (29.04 Gy). In mice irradiated in position 7 and 8, 0 mouse/group was found to have an early skin reaction. In position 8, immediately behind the SOBP, the mice showed no signs of irradiation (Score 0 in all mice).
Discussion
RBE for early skin reactions in mice has been determined in several studies, with a mid-SOBP RBE in the range of 1.1–1.15 when measured relative to 60Co [Citation25–27], and 0.74–0.89 when measured relative to orthovoltage X-rays [Citation28,Citation29]. As we normally use 240 keV for our experimental photon radiation, we produced a reference curve with this modality. Aware that there are biological differences from orthovoltage to megavoltage, we decided to also produce a reference curve at 6 MV. The obtained RBE values are in agreement with the previous reported RBE values.
RBE is not only dependent on LET, but also on dose and tissue type [Citation30]. Early reacting tissue, as the skin reactions used in the present study, with a high α/β ratio may display a lower RBE than late reacting tissue with a low α/β ratio [Citation5]. Furthermore, studies are pointing towards RBE increasing with decreasing dose, causing fractionation to potentially lead to a higher RBE [Citation31]. Hence, more in vivo data are needed to identify RBE differences in late and early endpoints using clinically relevant doses [Citation32].
The increased LET towards the distal edge of the SOBP has previously been shown in vitro to lead to higher biological effect. An increase in RBE can potentially be taken into account in dose planning systems, using RBE models as proposed by Wedenberg et al. [Citation33], as demonstrated in Ref. [Citation34], but the extent and magnitude of RBE in in vivo systems needs to be charted. Previous in vivo experiments addressing the distal edge issue have irradiated whole body or the mouse thorax, and looked at intestinal crypt regeneration and lung tolerance in the middle of the SOBP or in the distal edge. These studies have found only a small increase in effect in the distal part of the beam, compared to in vitro data [Citation15,Citation16]. Due to the size of a mouse body, this is measured over a large part of the SOPB. In a clinical situation, an enhanced effect even only in a few millimeters might have a severe impact.
In the current study, the effect of proton irradiation on early skin reactions was measured in 4 mm intervals through the SOBP. An increased effect was found in the position covering the very distal edge of the SOBP and the first part of the distal dose drop off. As the same dose plan was used in all positions, the physical dose in this distal edge position was 7% lower than in positions 1–5. Although there is a need to expand the current study to be able to calculate an exact enhancement ratio, it demonstrates an enhanced biological effect in vivo for early skin damage in the SOBP distal edge.
Acknowledgments
The authors would like to thank Inger Marie Horsman and Dorthe Grand for excellent technical help, Maria Beck for animal care and Katarzyna Majcher for assistance. This research was supported in part by PL-Grid Infrastructure.
Disclosure statement
No potential conflict of interest was reported by the authors.
Additional information
Funding
References
- Matsumoto Y, Matsuura T, Wada M, et al. Enhanced radiobiological effects at the distal end of a clinical proton beam: in vitro study. J Radiat Res. 2014;55:816–822.
- Lomax AJ, Bortfeld T, Goitein G, et al. A treatment planning inter-comparison of proton and intensity modulated photon radiotherapy. Radiother Oncol. 1999;51:257–271.
- Goodhead DT. Mechanisms for the biological effectiveness of high-LET radiations. J Radiat Res. 1999;40 Suppl:1–13.
- Jäkel O. The relative biological effectiveness of proton and ion beams. Z Med Phys. 2008;18:276–285.
- Gerweck LE, Kozin SV. Relative biological effectiveness of proton beams in clinical therapy. Radiother Oncol. 1999;50:135–142.
- Sørensen BS, Overgaard J, Bassler N. In vitro RBE-LET dependence for multiple particle types. Acta Oncol. 2011;50:757–762.
- Paganetti H, Niemierko A, Ancukiewicz M, et al. Relative biological effectiveness (RBE) values for proton beam therapy. Int J Radiat Oncol Biol Phys. 2002;53:407–421.
- Brush J, Lipnick SL, Phillips T, et al. Molecular mechanisms of late normal tissue injury. Semin Radiat Oncol. 2007;17:121–130.
- Mahajan A. Normal tissue complications from low-dose proton therapy. Health Phys. 2012;103:586–589.
- Mohan R, Mahajan A, Minsky BD. New strategies in radiation therapy: exploiting the full potential of protons. Clin Cancer Res. 2013;19:6338–6343.
- Britten RA, Nazaryan V, Davis LK, et al. Variations in the RBE for cell killing along the depth-dose profile of a modulated proton therapy beam. Radiat Res. 2013;179:21–28.
- Wouters BGBG, Skarsgard LDLD, Gerweck LELE, et al. Radiobiological intercomparison of the 160 MeV and 230 MeV proton therapy beams at the Harvard Cyclotron Laboratory and at Massachusetts General Hospital. Radiat Res 2015;183:174–187.
- Chaudhary P, Marshall TI, Perozziello FM, et al. Relative biological effectiveness variation along monoenergetic and modulated Bragg peaks of a 62-MeV therapeutic proton beam: a preclinical assessment. Int J Radiat Oncol Biol Phys. 2014;90:27–35.
- Cuaron JJ, Chang C, Lovelock M, et al. Exponential increase in relative biological effectiveness along distal edge of a proton bragg peak as measured by deoxyribonucleic acid double-strand breaks. Int J Radiat Oncol Biol Phys. 2016;95:62–69.
- Gueulette J, Slabbert JP, Böhm L, et al. Proton RBE for early intestinal tolerance in mice after fractionated irradiation. Radiother Oncol. 2001;61:177–184.
- Gueulette J, Bohm L, Slabbert JP, et al. Proton relative biological effectiveness (RBE) for survival in mice after thoracic irradiation with fractionated doses. Int J Radiat Oncol Biol Phys. 2000;47:1051–1058.
- Gueulette J, Blattmann H, Pedroni E, et al. Relative biologic effectiveness determination in mouse intestine for scanning proton beam at Paul Scherrer Institute, Switzerland. Influence of motion. Int J Radiat Oncol. 2005;62:838–845.
- Peeler CR, Mirkovic D, Titt U, et al. Clinical evidence of variable proton biological effectiveness in pediatric patients treated for ependymoma. Radiother Oncol. 2016;121:395–401.
- Horsman MR, Chaplin DJ, Overgaard J. Combination of nicotinamide and hyperthermia to eliminate radioresistant chronically and acutely hypoxic tumor cells. Cancer Res. 1990;50:7430–7436.
- Horsman MR, Siemann DW, Chaplin DJ, et al. Nicotinamide as a radiosensitizer in tumours and normal tissues: the importance of drug dose and timing. Radiother Oncol. 1997;45:167–174.
- Von der Maase H. Effect of cancer chemotherapeutic drugs on the radiation-induced skin reactions in mouse feet. Br J Radiol. 1984;57:697–707.
- Cortés-Giraldo MA, Carabe A. A critical study of different Monte Carlo scoring methods of dose average linear-energy-transfer maps calculated in voxelized geometries irradiated with clinical proton beams. Phys Med Biol. 2015;60:2645–2669.
- Grassberger C, Paganetti H. Elevated LET components in clinical proton beams. Phys Med Biol. 2011;56:6677–6691.
- Bassler N, Hansen DC, Lühr A, et al. SHIELD-HIT12A – a Monte Carlo particle transport program for ion therapy research. J Phys Conf Ser. 2014;489:12004.
- Raju MR, Carpenter SG. A heavy particle comparative study. Part IV: acute and late reactions. Br J Radiol. 1978;51:720–727.
- Nemoto K, Pickles T, Minchinton AI, et al. The relative biological effectiveness of the modulated proton beam at TRIUMF. Radiat Med. 1998;16:43–46.
- Tepper J, Verhey L, Goitein M, et al. In vivo determinations of RBE in a high energy modulated proton beam using normal tissue reactions and fractionated dose schedules. Int J Radiat Oncol Biol Phys. 1977;2:1115–1122.
- Tatsuzaki H, Inada T, Shimizu T, et al. Early skin reaction following 250 MeV proton peak irradiation. J Radiat Res. 1987;28:150–155.
- Tatsuzaki H, Arimoto T, Inada T, et al. Early skin reaction following superficial proton irradiation. J Radiat Res. 1991;32:175–180.
- Tommasino F, Durante M. Proton radiobiology. Cancers (Basel). 2015;7:353–381.
- Dasu A, Toma-Dasu I. Impact of variable RBE on proton fractionation. Med Phys. 2012;40:11705.
- Jones B. Why RBE must be a variable and not a constant in proton therapy. Br J Radiol. 2016;89:1–10.
- Wedenberg M, Lind BK, Hårdemark B. A model for the relative biological effectiveness of protons: the tissue specific parameter α/β of photons is a predictor for the sensitivity to LET changes. Acta Oncol. (Madr). 2013;52:580–588.
- Resch AF, Landry G, Kamp F, et al. Quantification of the uncertainties of a biological model and their impact on variable RBE proton treatment plan optimization. Phys Med. 2017;36:91–102.