Abstract
Introduction: Density changes occurring during fractionated radiotherapy in the pelvic region may degrade proton dose distributions. The aim of the study was to quantify the dosimetric impact of gas cavities and body outline variations.
Material and methods: Seven patients with locally advanced cervical cancer (LACC) were analyzed through a total of 175 daily cone beam computed tomography (CBCT) scans. Four-beams intensity-modulated proton therapy (IMPT) dose plans were generated targeting the internal target volume (ITV) composed of: primary tumor, elective and pathological nodes. The planned dose was 45 Gy [Relative-Biological-Effectiveness-weighted (RBE)] in 25 fractions and simultaneously integrated boosts of pathologic lymph nodes were 55–57.5 Gy (RBE). In total, 475 modified CTs were generated to evaluate the effect of: 1/gas cavities, 2/outline variations and 3/the two combined. The anatomy of each fraction was simulated by propagating gas cavities contours and body outlines from each daily CBCT to the pCT. Hounsfield units corresponding to gas and fat were assigned to the propagated contours. D98 (least dose received by the hottest 98% of the volume) and D99.9 for targets and V43Gy(RBE) (volume receiving ≥43 Gy(RBE)) for organs at risk (OARs) were recalculated on each modified CT, and total dose was evaluated through dose volume histogram (DVH) addition across all fractions.
Results: Weight changes during radiotherapy were between −3.1% and 1.2%. Gas cavities and outline variations induced a median [range] dose degradation for ITV45 of 1.0% [0.5–3.5%] for D98 and 2.1% [0.8–6.4%] for D99.9. Outline variations had larger dosimetric impact than gas cavities. Worst nodal dose degradation was 2.0% for D98 and 2.3% for D99.9. The impact on bladder, bowel and rectum was limited with V43Gy(RBE) variations ≤3.5 cm3.
Conclusion: Bowel gas cavities and outline variations had minor impact on accumulated dose in targets and OAR of four-field IMPT in a LACC population of moderate weight changes.
Introduction
The range of protons is mainly determined by their initial kinetic energy and the stopping power of the matter crossed on their paths [Citation1]. Therefore, when anatomical variations occur in between the acquisition of the planning computed tomography (pCT) and the subsequent treatment fractions, the path length of protons will be modified resulting in under/overshooting. Indeed, in this scenario, the conformality of dose distributions achieved on the pCT may be compromised and result in reduced target coverage and/or increased dose to organs at risk (OARs) [Citation2] which may again affect clinical outcome [Citation3].
Standard treatment of locally advanced cervical cancer (LACC) patients includes a combination of external beam radiation therapy (EBRT), concomitant chemotherapy and brachytherapy. The EBRT covers the primary tumor-related target and elective pelvic ± para-aortic lymph node targets to 45–50 Gy. Furthermore, pathologic lymph nodes may be boosted to for example 55–60 Gy [Citation4]. Cervix cancer patients are possible candidates for proton therapy combined with brachytherapy. The idea would be to replace the pelvic photon EBRT with proton therapy aiming to spare OARs [Citation5–11] and reduce consequently the adverse side effects [Citation12] while maintaining the brachytherapy boost which is essential for reaching high tumor doses and excellent local control [Citation13,Citation14]. In the pelvic region, substantial anatomical day-to-day variations occur due to the presence of organs with variable filling (bladder, bowel and rectum). The possible presence of gas in the bowel/rectum is of concern because variations in location and volume of such cavities induce substantial density changes that might result in suboptimal dose deposition. In addition, patients may experience changes in body weight and bowel volume during a course of RT. A variation in the thickness of the layer of fat as well as volume of bowel contents will also have impact on the depth of target and OARs and therefore on the dose deposition. Previous studies have shown for head and neck [Citation15] and lung [Citation16] the need to adapt the plan with intensity-modulated proton therapy (IMPT) when interfractional anatomical changes occur. In the pelvis region, Thörnqvist et al. and Soukup et al. have proven the impact of the latter for the irradiation of the prostate with two lateral opposed beams [Citation2,Citation17]. The study by Soukup et al. also showed the potential improvement when gas cavities on planning CT are overwritten with water Hounsfield Unit for irradiation of the prostate alone. The purpose of our study was to quantify the specific impact of gas cavities and body outline variation, with the proposed mitigating strategy for a LACC-elective target with four-field IMPT.
Material and methods
Patient data and contouring
Seven consecutive LACC patients who received photon pelvic irradiation at Aarhus University Hospital from March 2016 until June 2016 were included in this study. Five patients were diagnosed with FIGO (International Federation of Gynaecology and Obstetrics) stage IIB tumor and the remaining two patients had stage-IIIB disease. Two patients had each two pelvic lymph node metastases, while the remaining five patients were node negative. The median age was 69 years with range [38, 83 (years)].
Planning MR and CT images were available for all patients as well as daily cone beam computed tomography (CBCT) acquired prior to each radiotherapy fraction for the purpose of daily image guidance with setup according to bony registration. Target definition and contouring was conducted according to the Embrace II protocol (www.Embrace.dk) [Citation18]: initial GTV-T was defined as the extension of the primary gross tumour volume. Initial CTV-T HR (clinical target volume high risk) encompassed GTV-T and any remaining part of the cervix. Initial CTV-T LR (low risk) included CTV-T HR (with a maximum anterior–posterior margin of 5 mm while excluding bladder and rectum), the complete parametria bilaterally, the entire uterus and part of the vagina with a 20 mm margin from the most inferior part of CTV-T HR along the vaginal axis. To take into account the motion of OARs, the ITV-T LR (internal target volume) was defined from the CTV-T LR taking the anatomy on the MR planning scan as well as on CT scans with empty and full bladder into account. The CTV-E included elective pelvic nodal regions with/without the para-aortic region depending on the level of lymph node involvement. The ITV45 included the CTV-E and the ITV-T LR. Prescribed dose to the ITV45 was 45 Gy [Relative-Biological-Effectiveness-weighted (RBE)]/25fx. Lymph node metastases (CTV-Nx) were boosted with a simultaneous integrated boost to either 55 or 57.5 Gy(RBE) for lymph nodes inside and outside the pelvis, respectively. Bladder, bowel and rectum were delineated on all pCTs.
Proton treatment planning
Proton plans were simulated according to the target and OARs that were previously contoured, as well the dose and fractionation that was used clinically for photon therapy within the EMBRACE II protocol. The dose constraint for the ITV45 was D99.99%>42.75 Gy(RBE) (least dose received by the hottest 99.99% of the volume) and for PTV45 (planning target volume) D95%>42.75 Gy(RBE). This PTV constraint allowed an under-dosage of 5% of the PTV to reduce doses to the OARs, while the ITV45 constraint ensures coverage. Four beams, two lateral opposed and two posterior oblique (30, 90, 270, 330°) were used. This beam configuration was found to be optimal in terms of robustness, target coverage and OAR sparing in a previous study by Van de Schoot et al [Citation19]. IMPT treatment plans were generated using Eclipse version 13.7 (Varian Medical Systems, Palo Alto, CA). A spacing of 4 mm was used and the spot size (sigma) at isocenter in air was 4 mm for maximum energy (245 MeV). To secure the coverage of the ITV45, similarly to the photon planning, an ITV-to-PTV margin was set to 5 mm isotropically. No robustness feature was used during dose optimization. On pCT all gas pockets were virtually filled with mean bowel/feces Hounsfield Unit (44HU) assuming that gas pockets are not appearing reproducibly during radiotherapy. This is assumed to lead to more robust dose plans, as discussed by Soukup et al. for prostate [Citation2].
Calculation of fractional and accumulated dose
Gas cavities and body outlines were delineated on all 25 daily CBCTs for each patient (total 175 CBCTs). These contours were propagated to pCT through a bony match to reflect the treatment position of the patient. For each patient, 25 modified CT images were generated from the pCT anatomy on which the gas cavities of each fraction were overwritten with air HU (−992.8). Furthermore, 25 modified CT images were created to represent the body outline on CBCT by adding air HU when the CBCT body outline was inside the pCT outline or fat HU (−121.6) when the CBCT outline was outside the pCT outline. In this way, the resulting body reflected the actual body outline at each fraction. Modifications were not done outside the CBCT Field of View. In another 25 CT images, gas pockets and body outlines were combined. On each of these 75 CT images per patient, the beams were applied and the dose recalculated. Total accumulated dose was assessed by dose volume histogram (DVH) addition across all fractions for the following scenarios: (1) Body outline identical to pCT, but inclusion of CBCT bowel gas for all fractions, (2) Body outline according to each CBCT, but disregarding bowel gas, (3) Body outline and bowel gas according to each CBCT. Concretely, for each patient, D98, D99.9 and V43Gy(RBE) of the 25 modified CTs, after recalculation of the dose were added and divided by the total number of modified CTs. DVH addition has been termed ‘worst case scenario’, because it tends to under/over-estimate the delivered dose in low-/high-dose regions [Citation20]. The accumulated D98/D99.9 was evaluated for ITV45 and CTV-Ns and accumulated V43Gy(RBE) for bladder, bowel and rectum. The accumulated dose was divided by the pCT dose to assess the accumulated dose relative to the planned dose.
In order to quantify the missing information due to limited CBCT field of view (FOV), the ratio of the intersection of the ITV45 and all 25 CBCTs over the volume of the ITV45 was calculated. An index of 1 indicated that the ITV45 is entirely encompassed in all CBCTs FOV. Measuring D98/D99.9 on the entire ITV volume did not reflect the real D98/D99.9 value since the ITV was only modified within the CBCT FOV. To compensate for this lack of information, our results were linearly adjusted with the previously mentioned ratio. More explicitly, the change for instance in D98 (from pCT to modified CTs) observed on 50% of the ITV45 volume (because the other 50% are outside the CBCT FOV) was assumed to reflect only the half of the actual variation and was therefore in this case doubled. In this scenario, it was assumed that the nonmodified part of the ITV would be equivalently subject to dose degradation as the part of the ITV within the CBCT FOV. The indices used for the linear adjustments are shown for ITV45, bowel, bladder and rectum in Supplementary Table 1.
Patient 5 had a girth exceeding the lateral CBCT FOV and the full-body outline was not included on CBCT. This patient was therefore excluded for body outline variations analysis.
The volume of gas contained in the bowel within the CBCT FOV was measured for all patients in each fraction. The thickness (anterior–posterior (AP)) and width (right–left (RL)) of the body contour were measured on pCT and for all fractions on one transversal slice chosen according to an anatomical point of reference (promontorium when possible). The variations of gas volume and patient thickness/width were evaluated during the five weeks of treatment, and it was evaluated whether changes in dose correlated with these factors. Using a Pearson correlation test, we measured the linear correlation between gas volume, patient thickness and width at each fraction and dose degradation as well as the significance of the correlation with a p value threshold of .05.
Results
A significant correlation was found in 6/7 patients between the volume of gas per fraction and the relative ITV45 D98 dose degradation (, panel B). No correlation was found for patient 3. The Pearson correlation coefficient ranged from −0.78 to −0.48 for D98 in the six patients with significant correlation. The median [range] volume of gas contained in the bowel within the CBCT FOV was 27.4 cm3 [0.1 cm3 to 142 cm3] in all patients.
Figure 1. The relative ITV45 dose degradation (D98/D98pCT) on each treatment fraction induced by variations in body outline (panels A, C, D) and bowel gas (panel B), respectively. The dosimetric changes are shown in relation to changes in measured body outline and variation in bowel gas cavity volumes on daily CBCT.
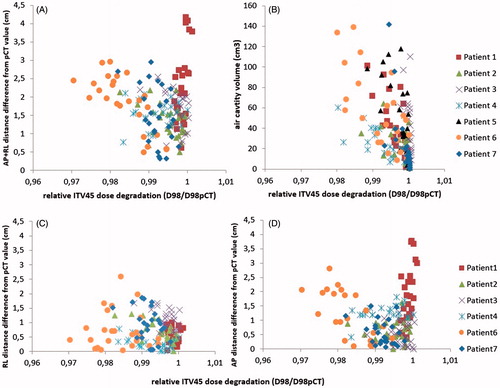
The changes in AP and RL distances did not correlate with the variations in body weight in the patients during EBRT (). In 5/6 patients, the dose degradation (D98) was significantly correlated with RL variation (three patients), AP variation (one patient) and/or the sum of RL and AP variation (three patients) (, panel A, C and D). The Pearson correlation coefficient for D98 ranged from −0.76 to −0.43 in the patients with significant correlation. Patient 1 did not show correlation with width or thickness while substantial AP distance differences from pCT occurred.
Table 1. Changes in body weight, anterior–posterior distance and right–left distance for all patients in cm after linear regression during external beam radiotherapy.
When gas cavities and body outline variations were combined, ITV45 D98 had a median [range] dose degradation of 1.0% [0.5–3.5%] after modification due to lack of CBCT FOV. Median [range] dose degradation of ITV45 D98 when gas cavities only were simulated was 0.3% [0.1–1.3%] and when only body outline variations were simulated was 0.7% [0.1–2.6%], after adjustment for lack of cranio-caudal length of CBCT ().
Table 2. Median, minimum and maximum accumulated D98 and D99.9 relative to planned for the ITV45 with gas inclusion only (Gas), body outline variations only (body) and the two combined (Gas + body) for our cohort studied, before and after modification for lack of CBCT FOV.
After modification for limited CBCT FOV, absolute accumulated ITV45 D98 dropped at worst by 0.6 Gy(RBE) when only gas was simulated and by 1.2 Gy(RBE) when only body outline variations were taken into consideration. When both were combined ITV45 D98 dropped by 1.6 Gy(RBE) at worst ().
Figure 2. Accumulated ITV45 D99.9 and D98 for seven LACC patients during external beam proton therapy were simulated: 1. Bowel gas cavities only, 2. Body contour variations only, 3. Bowel gas cavities and body contour variations combined, before and after adjustment for limited CBCT FOV.
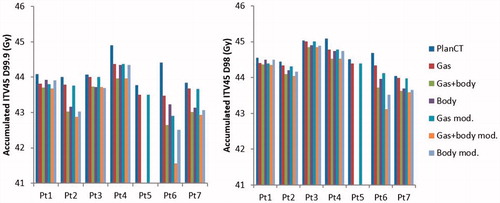
In , bladder, bowel and rectum increase/decrease in V43Gy(RBE) are shown according to the presence of gas cavities and body outline variation combined after adjustment for the lack of FOV of CBCTs. For patient 6, the bladder was sometimes entirely outside the CBCT FOV. For bladder, one patient had an increase in V43Gy(RBE) (0.7%) relative to V43Gy(RBE) on pCT. The greatest decrease in bladder V43Gy(RBE) was 6.1%. For bowel, four patients had a V43Gy(RBE) increase which reached 7.5% at its highest. For rectum, four patients had an increase in V43Gy(RBE) with a maximum of 4.5%. The maximum absolute increase in V43Gy(RBE) across all organs was 3.5 cm3.
Figure 3. Variation of accumulated V43Gy(RBE) for bladder bowel and rectum when bowel gas cavities and body outline variations on daily CBCT is combined in seven patients with LACC during proton therapy after adjustment for lack of CBCT FOV. (No information on bladder parameters for patient 6).
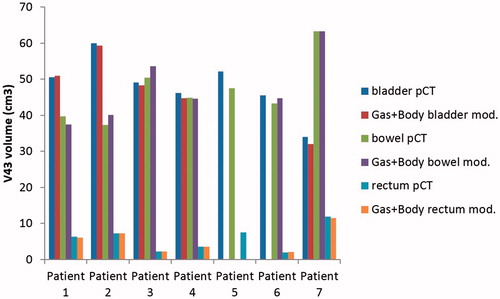
The CTV N1 and CTV N2 of patient 6 and patient 7 were for all fractions entirely encompassed in the CBCT FOV. Therefore, no adjustment was needed for the nodal dosimetric parameters. As shown in , accumulated D98 was degraded at worst by 2.0% when gas cavities and body outline variations were combined. As of accumulated D99.9, it was degraded by 2.3% at worst in the same scenario. The presence of gas cavities caused dose degradation of 0.4% for the worst case for both D98 and D99.9. The body outline variations had a bigger impact on nodal coverage with a dose degradation of 2.0% for the worst case for both D98 and D99.9 on CTV-Nx.
Table 3. Accumulated D98 and D99.9 relative to planned for the nodal CTVs of patient 6 and 7 with gas inclusion only, body outline variations only and the two combined.
Discussion
Most studies on proton therapy for LACC are dosimetric studies that compare proton plans with highly conformal photon plans. In general, it has been found that proton plans reduce the doses to OAR and at the same time maintain adequate dose to the target volume [Citation5–9] and could therefore contribute to a significant reduction in acute as well as in late morbidity following radiotherapy. However, there are only limited data on the dosimetric impact of uncertainties induced by organ motion and changes of filling of OARs on target coverage in proton therapy to pelvic tumors. This study showed that with a four-field dose-planning approach, the varying presence of gas cavities had limited dosimetric impact on the accumulated dose to the ITV45. Body outline variations had more impact on the accumulated dose to ITV45 compared with gas cavities. However, when gas cavities and body outlines were combined and analyzed together, which is the real scenario, the D98 and D99.9 degradations were median 1–2% and always less than 3.5% and 6.4%, respectively. The impact of gas cavities and body outline variations on nodal coverage for the anatomies studied was very limited in the two patients with positive lymph nodes with a worst dose degradation of 2.0% for D98 and 2.3% for D99.9. Gas cavities and body outline variations combined induced small variations (<3.5 cm3) in V43 for bladder, bowel and rectum.
The volume of gas as well as width and thickness was generally found to correlate with dose degradations. However, D98 dose degradation did not correlate with gas for patient 3 and with AP and RL variation for patient 1. For both cases, it is possibly explained by the fact that the variations occurred in the anterior part of the abdomen, away from the proton paths. In addition, overall weight variations were not found to correlate with ITV45 dose degradation. Therefore, weight variations of the present order of magnitude is not an indicator of dose degradation and cannot be used alone as a monitoring method. Patients with larger weight variations might be subject to larger dose degradation.
In the present study, a 5 mm isotropic ITV to PTV margin was used and robust dose optimization was not applied. Likely, the dosimetric impact of changes in gas cavities and body outline could be further reduced if the dose plans had been optimized robustly (by compensating for simulated range and setup errors) instead of using an isotropic ITV-to-PTV margin [Citation21]. Further robustness would probably also be possible with the use of Single Field Uniform Dose (SFUD), or setting the weights of the different beams in order to obtain a homogeneous coverage of the PTV from each field separately. This has been shown to result into more robust dose-plans for prostate patients [Citation2].
The impact of density changes depend on the beam configuration, as the beam directions determine which organs are crossed by the protons paths [Citation22,Citation23]. Organs which are subject to day-to-day variations will, if crossed, result in less robust dose plans. Also, the number of beams used influences the robustness as an additional beam equates to more distributed protons trajectories which, makes the plan less sensitive to occasional anatomical variations.
The method used in this study, implied overwriting with gas and fat HU the cavities and body contours. This approach is an approximation as anatomical changes occurring when the body outline is modified from day-to-day is not as simple as a displacement of the layer of fat. However, given that our results showed a limited effect of adding/removing tissue, the variations due to additional HU uncertainties in these subvolumes are expected to be minor. In addition, the linear correction applied to our raw results to compensate for the lack of CBCT FOV is an estimation based on assumptions (e.g., the missing part of the ITV would be subject to a dose degradation proportional to the one of the volume within CBCT FOV). For six patients out of seven, less than 13% of the ITV45 was outside the evaluation volume and the CBCTs were reproducibly centered which induces that the uncertainty of dose degradation estimates is expected to be limited. For one patient, 38% of the ITV45 was outside the CBCT intersection, mainly due to a few decentered CBCTs. We expect the correction to represent a worst case estimate as gas cavities and outline variations were not cropped to the intersection.
Our study simulates a course of RT in terms of gas cavities and body outline variations only. There are other factors such as setup errors [Citation24], contouring uncertainties [Citation25,Citation26], and interfractional motion of the target which have impact on the dose distribution. A major challenge with proton RT for LACC patients is to account for the interfractional motion. Indeed, for some patients, the primary target is subject to substantial motion from one day to another [Citation27]. In concrete terms, the uterus position depends for some patients on the bladder filling [Citation28], and more importantly, the cervix position (where the primary tumor is located) depends on the rectum filling [Citation29,Citation30]. A number of possible solutions to address the interfractional anatomical changes have been proposed for photons [Citation31] and should be further investigated for protons. The use of larger margins for instance, or the use of an adaptive strategy as demonstrated by Van de Schoot et al. [Citation32], are potential strategies. In the previously mentioned study, they compared image-guided adaptive photon therapy with image-guided adaptive proton therapy for cervix cancer patients based on a plan-library approach. They found an improved OARs sparing and target coverage for protons as compared to photons. They concluded that the adaptive strategy investigated using a plan-library approach is suitable for cervix patients. The utilization of plans optimized on CT scans acquired daily would further reduce uncertainties due to interfractional motion and would allow for the use of tighter margins and better sparing of OARs. Technical challenges have to be solved before this option becomes possibly established. Finally, intrafraction motion may impact the robustness in case of utilization of plan of the day and tight margins.
Currently, robust planning is commonly based on assumptions of isocenter uncertainties as well as a 3.5% range uncertainty which has been shown sufficient to compensate for the stopping power ratio (SPR)–related errors [Citation33]. This source of error due to HU conversion into SPR is disregarded in our study and its consequences on dose degradation will have to be combined to the results from the present study. Utilization of dual-energy CT has potential to reduce stopping power uncertainties [Citation34,Citation35]. Furthermore, bones have a high stopping power and may have an impact on the dose distribution induced by patient positioning uncertainty as shown previously in prostate cancer [Citation22]. However, in cervix cancer, it is recommended that the positioning of patients is done according to a bony match. This procedure minimizes the mismatch of the bony structures between the pCT and the treatment position [Citation24]. These factors need to be studied and their contribution quantified to draw broader conclusions on the robustness of proton therapy for LACC patients.
In conclusion, the present study found limited dosimetric impact of variations in bowel gas cavities and patient outline. This finding is under the condition of the chosen dose-planning characteristics including four-field technique as well as moderate weight variations. Patients with major weight changes may be subject to larger dose degradation and should be considered for replanning. Utilization of strategies that take inter-fraction motion of cervix and uterus into account are needed before proceeding with clinical implementation of IMPT for cervix cancer patients.
IONC_A_1376753_Supplementary_Information.zip
Download Zip (10.8 KB)Disclosure statement
No potential conflict of interest was reported by the authors.
Additional information
Funding
References
- Newhauser WD, Zhang R. The physics of proton therapy. Phys Med Biol. 2015;60:R155–R209.
- Soukup M, Söhn M, Yan D, et al. Study of robustness of IMPT and IMRT for prostate cancer against organ movement. Int J Radiat Oncol Biol Phys. 2009;75:941–949.
- Nesvacil N, Tanderup K, Lindegaard JC, et al. Can reduction of uncertainties in cervix cancer brachytherapy potentially improve clinical outcome? Radiother Oncol. 2016;120:390–396.
- Ramlov A, Kroon PS, Jürgenliemk-Schulz IM, et al. Impact of radiation dose and standardized uptake value of (18)FDG PET on nodal control in locally advanced cervical cancer. Acta Oncol. 2015;54:1567–1573.
- Georg D, Georg P, Hillbrand M, et al. Assessment of improved organ at risk sparing for advanced cervix carcinoma utilizing precision radiotherapy techniques. Strahlenther Onkol. 2008;184:586–591.
- Marnitz S, Wlodarczyk W, Neumann O, et al. Which technique for radiation is most beneficial for patients with locally advanced cervical cancer? Intensity modulated proton therapy versus intensity modulated photon treatment, helical tomotherapy and volumetric arc therapy for primary radiation – an intraindividual comparison. Radiat Oncol. 2015;10:91.
- van de Sande MAE, Creutzberg CL, van de Water S, et al. Which cervical and endometrial cancer patients will benefit most from intensity-modulated proton therapy? Radiother Oncol. 2016;120:397–403.
- Hashimoto S, Shibamoto Y, Iwata H, et al. Whole-pelvic radiotherapy with spot-scanning proton beams for uterine cervical cancer: a planning study. J Radiat Res. 2016;57:524–532.
- Song WY, Huh SN, Liang Y, et al. Dosimetric comparison study between intensity modulated radiation therapy and three-dimensional conformal proton therapy for pelvic bone marrow sparing in the treatment of cervical cancer. J Appl Clin Med Phys. 2010;11:3255.
- Lin LL, Kirk M, Scholey J, et al. Initial report of pencil beam scanning proton therapy for posthysterectomy patients with gynecologic cancer. Int J Radiat Oncol Biol Phys. 2016;95:181–189.
- Dinges E, Felderman N, McGuire S, et al. Bone marrow sparing in intensity modulated proton therapy for cervical cancer: efficacy and robustness under range and setup uncertainties. Radiother Oncol. 2015;115:373–378.
- Ramlov A, Pedersen EM, Røhl L, et al. Risk factors for pelvic insufficiency fractures in locally advanced cervical cancer following intensity modulated radiation therapy. Int J Radiat Oncol Biol Phys. 2017;97:1032–1039.
- Tanderup K, Fokdal LU, Sturdza A, et al. Effect of tumor dose, volume and overall treatment time on local control after radiochemotherapy including MRI guided brachytherapy of locally advanced cervical cancer. Radiother Oncol. 2016;120:441–446.
- Sturdza A, Pötter R, Fokdal LU, et al. Image guided brachytherapy in locally advanced cervical cancer: improved pelvic control and survival in RetroEMBRACE, a multicenter cohort study. Radiother Oncol. 2016;120:428–433.
- Góra J, Kuess P, Stock M, et al. ART for head and neck patients: on the difference between VMAT and IMPT. Acta Oncol. 2015;54:1166–1174.
- Hoffmann L, Alber M, Jensen MF, et al. Adaptation is mandatory for intensity modulated proton therapy of advanced lung cancer to ensure target coverage. Radiother Oncol. 2017;122:400–405.
- Thörnqvist S, Muren LP, Bentzen L, et al. Degradation of target coverage due to inter-fraction motion during intensity-modulated proton therapy of prostate and elective targets. Acta Oncol. 2013;52:521–527.
- EmbraceStudy [Internet]. 2017. Available from: https://www.embracestudy.dk/
- van de Schoot AJ, Visser J, van Kesteren Z, et al. Beam configuration selection for robust intensity-modulated proton therapy in cervical cancer using Pareto front comparison. Phys Med Biol. 2016;61:1780–1794.
- Andersen ES, Muren LP, Sørensen TS, et al. Bladder dose accumulation based on a biomechanical deformable image registration algorithm in volumetric modulated arc therapy for prostate cancer. Phys Med Biol. 2012;57:7089.
- Liu W, Mohan R, Park P, et al. Dosimetric benefits of robust treatment planning for intensity modulated proton therapy for base-of-skull cancers. Pract Radiat Oncol. 2014;4:384–391.
- Andersen AG, Casares-Magaz O, Petersen J, et al. Beam angle evaluation to improve inter-fraction motion robustness in pelvic lymph node irradiation with proton therapy. Acta Oncol. 2017;56:846–852.
- Andersen AG, Casares-Magaz O, Muren LP, et al. A method for evaluation of proton plan robustness towards inter-fractional motion applied to pelvic lymph node irradiation. Acta Oncol. 2015;54:1643–1650.
- Laursen LV, Elstrøm UV, Vestergaard A, et al. Residual rotational set-up errors after daily cone-beam CT image guided radiotherapy of locally advanced cervical cancer. Radiother Oncol. 2012;105:220–225.
- Eminowicz G, Rompokos V, Stacey C, et al. The dosimetric impact of target volume delineation variation for cervical cancer radiotherapy. Radiother Oncol. 2016;120:493–499.
- Eminowicz G, McCormack M. Variability of clinical target volume delineation for definitive radiotherapy in cervix cancer. Radiother Oncol. 2015;117:542–547.
- Jadon R, Pembroke CA, Hanna CL, et al. A systematic review of organ motion and image-guided strategies in external beam radiotherapy for cervical cancer. Clin Oncol R Coll Radiol. 2014;26:185–196.
- Heijkoop ST, Langerak TR, Quint S, et al. Quantification of intra-fraction changes during radiotherapy of cervical cancer assessed with pre- and post-fraction cone beam CT scans. Radiother Oncol. 2015;117:536–541.
- Taylor A, Powell MEB. An assessment of interfractional uterine and cervical motion: implications for radiotherapy target volume definition in gynaecological cancer. Radiother Oncol. 2008;88:250–257.
- Eminowicz G, Rompokos V, Stacey C, et al. Understanding the impact of pelvic organ motion on dose delivered to target volumes during IMRT for cervical cancer. Radiother Oncol. 2017;122:116–121.
- Thörnqvist S, Hysing LB, Tuomikoski L, et al. Adaptive radiotherapy strategies for pelvic tumors – a systematic review of clinical implementations. Acta Oncol. 2016;55:943–958.
- van de Schoot AJAJ, de Boer P, Crama KF, et al. Dosimetric advantages of proton therapy compared with photon therapy using an adaptive strategy in cervical cancer. Acta Oncol. 2016;55:892–899.
- Yang M, Zhu XR, Park PC, et al. Comprehensive analysis of proton range uncertainties related to patient stopping-power-ratio estimation using the stoichiometric calibration. Phys Med Biol. 2012;57:4095–4115.
- Taasti VT, Petersen JBB, Muren LP, et al. A robust empirical parametrization of proton stopping power using dual energy CT. Med Phys. 2016;43:5547–5560.
- Taasti VT, Høye EM, Hansen DC, et al. Technical Note: Improving proton stopping power ratio determination for a deformable silicone-based 3D dosimeter using dual energy CT. Med Phys. 2016;43:2780–2784.