Abstract
Background: Proton therapy can reduce the low and intermediate radiation dose to uninvolved brain tissue in children with intracranial ependymomas, which may improve functional outcomes and reduce second malignancies in survivors. Accordingly, ependymoma has become the most common pediatric tumor treated with proton therapy, yet data on efficacy and toxicity are limited.
Material and methods: Between June 2007 and February 2017, 179 children (≤21 years old) with nonmetastatic grade II/III intracranial ependymoma received proton therapy at our institution. Median age, 3.5 years (range, 0.7–21); 58% were male. Most (66%) tumors were in the posterior fossa and classified as WHO grade III (67%). 27% underwent multiple operations to maximize the extent of resection; ultimately 85% had a gross total or near total tumor resection before radiotherapy. 33% received preradiation chemotherapy. Median radiation dose in children ≤3 years old, 54 Gy(RBE). Most (>90%) children over 3 years old received 59.4 Gy(RBE). Patient and treatment variables were assessed for correlation with disease control.
Results: Median follow-up, 3.2 years. 3-year local control, progression-free survival, and overall survival rates were 85%, 76%, and 90%, respectively. First site of progression was local, metastatic, or simultaneous in 14, 17 and 6 patients, respectively. On multivariate analysis, subtotal resection was associated with inferior local control (67% vs. 88%; p ≤ .01) and progression-free survival (59% vs. 79%; p < .05). Male sex was associated with inferior progression-free (67% vs. 87%; p< .05) and overall survival (84% vs. 99%; p < .01). The 3-year CTCAE grade 2 + brainstem toxicity rate was 5.5% (95% CI: 2.9–10.2), including 1 grade 5 toxicity.
Conclusions: This series of proton therapy for pediatric intracranial ependymoma demonstrates disease control comparable to photon series without unexpected toxicity. Subtotal resection and male sex were associated with inferior disease control. Additional follow-up to quantify the expected reductions in late toxicity with proton therapy is ongoing.
Introduction
Ependymomas are one of the most common malignant brain tumors diagnosed in children under 10 years of age [Citation1]. Over the past four decades, cure rates have steadily improved [Citation1], largely attributable to advances in surgery and radiation in patients with nonmetastatic disease. However, the combination of young patient age and aggressive local therapy puts children with ependymoma at risk of significant late effects such as neurocognitive impairment, neurologic deficits, neuroendocrine deficiency and second malignancies. Several studies have attempted to mitigate the impact of late radiation toxicity through selective radiation avoidance, systematic reduction in the size of target volumes, and the use of advanced radiation techniques. Proton therapy is particularly promising since it allows for reductions in the low and intermediate radiation dose to normal tissue outside of the target volume [Citation2]. Accordingly, ependymomas have become the most common pediatric tumor treated with proton therapy in the United States [Citation3], and small heterogeneous studies have recently been published describing early patient outcomes [Citation4–6]. Given the low probability of a randomized study examining radiation modality in pediatric ependymoma, outcome studies continue to help characterize the therapeutic ratio of proton therapy relative to other techniques. To contribute to such data on treatment efficacy and toxicity, we analyzed a cohort of intracranial pediatric ependymoma patients treated at the University of Florida enrolled on an institutional prospective outcomes study. The purpose of the study was to address three main questions:
Does proton therapy offer comparable disease control relative to contemporary photon therapy techniques?
Does proton therapy introduce unique toxicity patterns relative to contemporary photon therapy techniques?
Independent of radiation modality, what does this large patient cohort teach us about risk factors, surgical approach and treatment technique?
Material and methods
Between June 2007 and February 2017, 1178 pediatric patients (age ≤21 years) were treated with proton therapy at the University of Florida. Under an institutional review board-approved prospective outcome study, 179 of these pediatric patients were identified with intracranial ependymoma that fit the following criteria: (a) nonmetastatic disease, (b) WHO grade 2 or 3 tumors, and (c) no prior radiation. As part of the study, acute and late treatment toxicity information was collected and graded according to the National Cancer Institute’s Common Terminology Criteria for Adverse Events, during weekly on-treatment and follow-up visits.
Most patients had historically unfavorable characteristics at diagnosis: 120 (67%) had WHO grade-3 tumors, 119 (66%) had posterior fossa tumors, 103 (58%) were male, and 98 (55%) were ≤3 years old. Complete patient characteristics are listed in . Overall, 152 (85%) patients had a gross total or near total tumor resection (<5 mm residual tumor) at the time of irradiation. To achieve maximum resection, 48 (27%) of the patients underwent more than one operation. The median interval between the last operation and the start of radiation in children who did not receive preradiation chemotherapy was 55 days (range, 12–283 days); 95% of patients started radiation within 109 days. Of the 59 (33%) patients who received preradiation chemotherapy, 36 received high-dose methotrexate.
Table 1. Patient Characteristics.
Patients were treated according to the University of Florida pediatric treatment guidelines for ependymoma (). As an exception, patients between 18 months and 3 years who were enrolled on Children’s Oncology Group (COG) study ACNS0831 (n = 6) underwent treatment in accordance with COG study guidelines and therefore received the second planning target volume (PTV2) boost to 59.4 Gy(RBE). Overall, 83% of children were treated according to the University of Florida guidelines and the median radiation dose prescribed was 59.4 Gy(RBE) for all patients >3 years old and 54 Gy(RBE) for children ≤3 years old.
Table 2. University of Florida Pediatric Treatment Guidelines for Ependymoma.
The primary goal of the radiation plan was to ensure that the entire clinical target volume (CTV) was encompassed by >99% of the nominal dose and the entire planning target volume (PTV) was covered by 95% of the nominal dose. Target coverage was modified on a case-by-case basis to limit the brainstem, spinal cord, or optic chiasm dose. All patients were treated with sequential, double-scattered proton plans. Most patients were treated with three fields daily, most commonly using a mix of posterior beams and out-of-plane superior obliques to avoid multiple beams ending on shared brainstem tissue beyond the CTV. The distal and proximal beam margin in millimeters was calculated though the empirically derived institutional formula of (2.5%×field range) + 1.5 mm. The aperture margin was 4–7 mm from the PTV edge. The typical beam smearing margin, used to mitigate the effect of position variability and image misregistration on the distal range, was 5 mm. The median duration of radiation was 47 days and 43 days in patients who received 59.4 Gy(RBE) and 54 Gy(RBE), respectively. Eleven (6%) patients required one day of treatment with photons due to cyclotron downtime and 121 (68%) patients required anesthesia for immobilization during treatment.
Patient characteristics and treatment variables were examined for association with disease control and toxicity according to study guidelines. In the absence of symptoms, MRI was recommended every 3–4 months for the first 3 years, every 6 months for the next 2 years, and once annually thereafter. Toxicity evaluations were customized for each patient according to cochlea, temporal lobe, pituitary, hypothalamus and optic nerve dose. If externally referred patients were unable to return to our institution for follow-up testing, testing results were collected from local medical teams. All statistical analyses were performed with SAS and JMP software (SAS Institute, Cary, NC). The Kaplan–Meier product limit method provided estimates of local control, progression-free survival, overall survival and freedom from grade 2 + radiation-related toxicity; the log-rank test statistic assessed the level of statistical significance between strata of selected prognostic factors for each endpoint. The impact of tumor grade, extent of resection and patient gender were assessed simultaneously with proportional hazards regression.
Results
Disease control outcomes
With a median follow-up of 3.2 years, the three-year actuarial progression-free survival and overall survival rates were 75.9% and 90.4%, respectively (). The first site of progression was local, metastatic or simultaneous in 14, 17 and 6 patients, respectively. The three-year actuarial local control rate was 85.4% (95% CI, 78.3–90.4). The three-year freedom from isolated distant recurrence was 84.6% (95% CI, 77.8–89.6). The median time to local failure was 1.4 years (range, 0.2–2.5) and the median time to distant failure was 1.0 year (range, 0.2–6.1). Two patients were lost to follow-up at 1 and 4 years following treatment. On univariate analysis (), only extent of resection significantly correlated with local control: Patients who underwent a GTR/NTR experienced a local control rate of 88.4% compared to 66.5% for those who underwent a subtotal resection (p < .005). Of note, higher radiation doses were not associated with improved local control, even when stratified by extent of resection. In terms of progression-free survival, subtotal resection (78.5% vs. 59.4%; p < .05) and male gender (87.3% vs. 67%; p < .05) were associated with inferior outcomes. Subtotal resection (92.8% vs 76.5%; p < .05) and male gender (98.5% vs 84.4%; p < .01) were likewise associated with poorer overall survival. On multivariate analysis including tumor grade, extent of resection and patient gender, patients with subtotal resection maintained significant association with inferior local control (HR, 0.3; range, 0.1–0.7; p < .01) and progression-free survival (HR, 0.5; range, 0.2–1; p < .05). Subtotal resection also demonstrated a borderline association with overall survival (HR, 0.4; range, 0.1–1; p = .057). Male gender patients maintained a significant association with inferior progression-free survival (HR, 0.4; range, 0.2-0.9; p < .05) and overall survival (HR, 0.1; range, 0–0.6; p < .5).
Figure 1. Kaplan–Meier curves for overall survival (OS), progression-free survival (PFS), and local control (LC) rates at 3 years including the number of patients at risk.
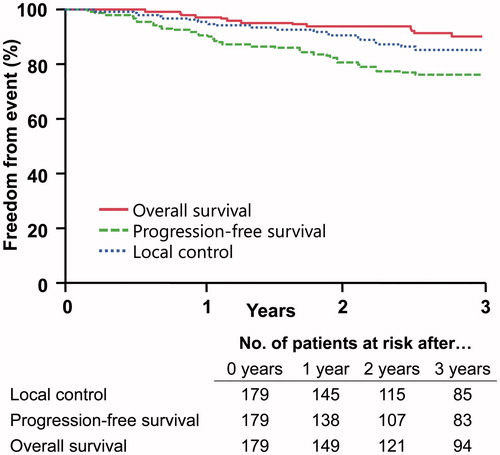
Table 3. Disease control outcomes at 3 years and univariate analysis.
Toxicity outcomes
Grade 2 + acute toxicity attributable to radiotherapy included 18 (10%) patients with nausea or vomiting requiring ondansetron for symptom control. One patient (0.6%) experienced a headache requiring opioid analgesia. No patients required steroids. Grade 2 + late toxicity attributable to radiotherapy included 13 patients (7.3%) who developed hormone deficiency, most commonly growth hormone (11/13). Eleven patients (6.1%) developed new hearing loss requiring hearing aids: seven with bilateral and four with unilateral deficits. Of note, eight of these 11 patients received cisplatin chemotherapy, including six of the seven with bilateral hearing deficits. Six patients (3.4%) developed vasculopathy causing transient ischemic symptoms or stroke at a median 1.2 years (range, 0.8–7.1 years) from the completion of radiotherapy. One of the six patients had a hereditary prothrombin disorder. One patient required vascular surgery bypass, three were started on prophylactic aspirin, and two were observed. Ten patients developed symptomatic brainstem toxicity, corresponding to a 3-year actuarial rate of grade 2 + brainstem toxicity of 5.5% (95% CI: 2.9–10.2). The median duration to toxicity onset was 3 months, and 9 of 10 toxicities occurred within 4 months (the outlier developed toxicity 66 months following radiation). There were eight cases of grade 2 toxicity (4.5%), one case of grade 3 toxicity (0.5%), and one case (0.5%) of grade-5 brainstem toxicity. All living patients have recovered with a median KPS of 80 (range, 70–90). There were no radiation-induced second tumors or cases of cervical subluxation.
Discussion
The management of pediatric ependymoma is a challenge due to both patient and tumor characteristics. However, prospective and retrospective studies indicate that radiation therapy plays an important role in reducing the risk of local recurrence. Additional dosimetric and retrospective studies suggest that proton therapy can reduce the low and intermediate radiation dose to developing brain tissue without compromising disease control. Independent of radiation modality, as the largest single-institution report of children treated for ependymoma, this study contributes valuable data to address general management of ependymoma as well as the specific role of proton therapy.
In this analysis, three questions were addressed:
1. Does proton therapy offer comparable disease control relative to contemporary photon therapy techniques?
Modern multi-institution studies from the United States and Europe involving almost exclusively photon therapy report local control rates between 75% and 79%, progression-free survival rates between 50% and 65%, and overall survival rates between 71% and 86% [Citation7–10]. Single-institution photon studies published since 2000, including many with longer follow-up [Citation11], demonstrate local control rates between 31% and 87%, progression-free survival rates between 25% and 69%, and overall survival rates between 35% and 86% [Citation12–18]. Our patient cohort demonstrated 3-year local control, progression-free survival and overall survival rates of 85%, 76% and 90%, respectively, suggesting comparable outcomes with proton therapy. These estimates of disease control reflect other contemporary series of pediatric ependymoma patients treated with proton therapy [Citation4,Citation5].
2. Does proton therapy introduce unique toxicity patterns relative to contemporary photon therapy techniques?
No modern series has reported acute toxicity during radiotherapy for intracranial ependymoma in children; therefore, data herein can serve as a benchmark for future research. In comparison to other pediatric brain tumor patients treated with photon therapy, the low rate of nausea, vomiting and headache compare favorably [Citation19]. Our findings are also consistent with data demonstrating a comparative reduction in nausea and vomiting in adult brain tumor patients treated with proton therapy [Citation20]. As with acute toxicity, very few modern photon series report intermediate interval toxicity, like hearing loss and endocrinopathy that can occur in children within the first 5 years of treatment. The lack of data exists despite the widespread enthusiasm and usage of intensity-modulated photon radiotherapy in children over the past decade, a technique intended to reduce the side effects reported in historic series utilizing nonconformal or 3D conformal approaches [Citation11]. Based on dosimetric modeling, proton therapy may be able to further reduce the radiation dose to tissue beyond the PTV. Although the rate of hearing loss and endocrinopathy is low in the present proton series (particularly in children who did not receive chemotherapy), it is hoped that more data from contemporary photon series emerge that allow meaningful comparison across these domains. One toxicity not lacking comparative data is radiation-related brainstem injury. Combining data from 3 recent photon series, symptomatic brainstem toxicity was observed in 11 of 207 children with ependymoma for a rate of approximately 5.3% [Citation6,Citation15,Citation21]. In three contemporaneous proton series, symptomatic brainstem toxicity was observed in 14 of 340 children with ependymoma for a rate of approximately 4.1% [Citation4–6], which is consistent with the 5.5% rate observed in this series. The similar rate of brainstem toxicity observed between modern proton and photon series is to be expected following a treatment in which the target volume often overlaps the organ at risk (i.e., the PTV encompasses part of the brainstem).
3. Independent of radiation modality, what does this large patient cohort teach us about risk factors, surgical approach, and treatment technique?
Beyond the value that this dataset offers regarding particle therapy, the large cohort permits a robust multivariate analysis that informs patient risk factors and optimal treatment parameters across modalities. Most notably, these outcomes confirm the importance of complete resection, suggesting it has an impact on not just disease control but also early survival endpoints. This insight, which has been previously identified by others [Citation8,Citation10,Citation11,Citation15,Citation16,Citation18], is critical when counseling families on the risk/benefit ratio of aggressive and often neurologically morbid attempts at tumor resection. Likewise, the adverse outcome observed in male patients with ependymoma has also been reported in other large multivariate analyzes [Citation13,Citation15,Citation22], which may be related to the higher propensity of the EPN-PFA molecular subtype in males [Citation23]; further research is needed in this area.
Additionally, our findings reveal that neither young age nor high tumor grade demonstrate significant statistical association with disease recurrence. Also noteworthy is that radiation doses >55.8 Gy, even when stratified by extent of resection, did not demonstrate a clear association with disease control. The data related to disease control and dose escalation is mixed [Citation7,Citation12,Citation15,Citation24] and the lack of correlation may simply reflect the poor sensitivity of a subset analysis with too few patients. Importantly, our results did not suggest an increase in “marginal misses” and local recurrences despite the conservative CTV1 margin (5 mm) and the high conformality of proton therapy. These data are consistent with an earlier series published by Ares et al [Citation5]. Finally, there was no detriment to outcomes across the range of time intervals observed between last surgery and the commencement of radiation. Patient outcomes were similar regardless of the length of time between definitive resection and the start of radiotherapy (≤ 8 vs. > 8 weeks). Other studies have likewise demonstrated that a median interval between surgery and radiation extending beyond 6 to 7 weeks is not associated with inferior outcomes [Citation8,Citation15]. Nevertheless, the current SIOP and COG ependymoma studies require that patients begin radiation within 6 weeks of surgery or 3 weeks of study enrollment, respectively. This policy deserves reexamination as it may unnecessarily rush patients recovering from surgery and preclude subjects from enrollment [Citation25].
This analysis is limited by its modest follow-up duration, and further data from this prospective outcome study are critical to confirming the early findings. The absence of molecular subtyping, which informs prognosis and may advance future risk stratification [Citation23], is another limitation of this series. Nevertheless, these findings contribute valuable outcome data on the most common pediatric tumor treated with proton therapy in the United States [Citation3] and suggests that proton therapy affords comparable disease control without producing unexpected toxicity. Quantifying the magnitude in reduction of late toxicity with proton therapy will require continued follow-up as well as more publications sharing equivalent photon data. Independent of radiation modality, however, this series represents the largest single-institution report of intracranial pediatric ependymoma outcomes and in that capacity contributes useful information for overall disease management, patient and family counseling, technical radiotherapy considerations and surveillance guidance.
Disclosure statement
Dr. Indelicato and Dr. Bradley have received prior funding from an unrestricted educational grant from IBA. All other authors report no conflicts of interest.
References
- Ostrom QT, de Blank PM, Kruchko C, et al. Alex's lemonade stand foundation infant and childhood primary brain and central nervous system tumors diagnosed in the United States in 2007-2011. Neuro Oncol. 2015; 16:x1–x36.
- MacDonald SM, Safai S, Trofimov A, et al. Proton radiotherapy for childhood ependymoma: initial clinical outcomes and dose comparisons. Int J Radiat Oncol Biol Phys. 2008;71:979–986.
- Chang AL, Yock TI, Mahajan A, et al. Pediatric proton therapy: patterns of care across the United States. Int J Particle Ther. 2014;1:357–367.
- Macdonald SM, Sethi R, Lavally B, et al. Proton radiotherapy for pediatric central nervous system ependymoma: clinical outcomes for 70 patients. Neuro Oncol. 2013;15:1552–1559.
- Ares C, Albertini F, Frei-Welte M, et al. Pencil beam scanning proton therapy for pediatric intracranial ependymoma. J Neurooncol. 2016;128:137–145.
- Sato M, Gunther JR, Mahajan A, et al. Progression-free survival of children with localized ependymoma treated with intensity-modulated radiation therapy or proton-beam radiation therapy. Cancer. 2017;123:2570–2578.
- Tensaouti F, Ducassou A, Chaltiel L, et al. Patterns of failure after radiotherapy for pediatric patients with intracranial ependymoma. Radiother Oncol. 2017;122:362–367.
- Massimino M, Miceli R, Giangaspero F, et al. Final results of the second prospective AIEOP protocol for pediatric intracranial ependymoma. Neuonc. 2016;18:1451–1460.
- Garvin JH Jr, Selch MT, Holmes E, et al. Phase II study of pre-irradiation chemotherapy for childhood intracranial ependymoma. Children's Cancer Group protocol 9942: a report from the Children's Oncology Group. Pediatr Blood Cancer. 2012;59:1183–1189.
- Ducassou A, Murraciole X, Chaltiel L, et al. OC-0309: Role of age, grade and RT dose on outcome of 177 ependymoma - 13 years experience of Child's cancer French Society. Radiother Oncol. 2015;115:S155.
- Swanson EL, Amdur RJ, Morris CG, et al. Intracranial ependymomas treated with radiotherapy: long-term results from a single institution. J Neurooncol. 2011;102:451–457.
- Mansur DB, Perry A, Rajaram V, et al. Postoperative radiation therapy for grade II and III intracranial ependymoma. Int J Radiat Oncol Biol Phys. 2005;61:387–391.
- Oya N, Shibamoto Y, Nagata Y, et al. Postoperative radiotherapy for intracranial ependymoma: analysis of prognostic factors and patterns of failure. J Neurooncol. 2002;56:87–94.
- van Veelen-Vincent ML, Pierre-Kahn A, Kalifa C, et al. Ependymoma in childhood: prognostic factors, extent of surgery, and adjuvant therapy. J Neurosurg. 2002;97:827–835.
- Merchant TE, Li C, Xiong X, et al. Conformal radiotherapy after surgery for paediatric ependymoma: a prospective study. Lancet Oncol. 2009;10:258–266.
- Shu HK, Sall WF, Maity A, et al. Childhood intracranial ependymoma: twenty-year experience from a single institution. Cancer. 2007;110:432–441.
- Jaing TH, Wang HS, Tsay PK, et al. Multivariate analysis of clinical prognostic factors in children with intracranial ependymomas. J Neurooncol. 2004;68:255–261.
- Paulino AC, Wen BC, Buatti JM, et al. Intracranial ependymomas: an analysis of prognostic factors and patterns of failure. Am J Clin Oncol. 2002;25:117–122.
- Merchant TE, Kun LE, Wu S, et al. Phase II trial of conformal radiation therapy for pediatric low-grade glioma. J Clin Oncol. 2009;27:3598–3604.
- Brown AP, Barney CL, Grosshans DR, et al. Proton beam craniospinal irradiation reduces acute toxicity for adults with medulloblastoma. Int J Radiat Oncol Biol Phys. 2013;86:277–284.
- Nanda RH, Ganju RG, Schreibmann E, et al. Correlation of acute and late brainstem toxicities with dose-volume data for pediatric patients with posterior fossa malignancies. Int J Radiat Oncol Biol Phys. 2017;98:360–366.
- Ramaswamy V, Hielscher T, Mack SC, et al. Therapeutic impact of cytoreductive surgery and irradiation of posterior fossa ependymoma in the molecular era: a retrospective multicohort analysis. JCO. 2016;34:2468–2477.
- Pajtler KW, Witt H, Sill M, et al. Molecular classification of ependymal tumors across all CNS compartments, histopathological grades, and age groups. Cancer Cell. 2015;27:728–743.
- Ducassou A, Muracciole X, Chaltiel L, et al. PD-017: survival and prognostic factors in 211 intracranial ependymomas – 13 years experience of Child's Cancer French Society. Pediatr Blood Cancer. 2015;62:S214.
- Merchant TE. Current clinical challenges in childhood ependymoma: a focused review. J Clin Oncol. 2017;35:2364–2369.