Abstract
Background: The α/β values for prostate cancer (PCa) are usually assumed to be low (1.0–1.8 Gy). This study estimated the α/β values of PCa from phase III randomized trials of conventional (CRT) versus hypofractionated (HRT) external beam radiotherapy (RT), reported as isoeffective in terms of their 5-year biochemical (BF) or biochemical and/or clinical failure (BCF) rates.
Material and methods: The α/β for each trial was estimated from the equivalent biological effective doses using the linear-quadratic model for each of their HRT and CRT schedules. The cumulative outcomes of these trials were evaluated by meta-analysis for odds ratio (OR), risk ratio (RR) and risk difference (RD).
Results: Eight trials from seven studies, randomized 6993 patients between CRT (n = 2941) and HRT (n = 4052). RT treatment varied between the two treatment groups in terms of dose/fraction, total dose, overall treatment time and %patients on androgen deprivation therapy (ADT). Differences in OR, RR, and RD for both BF and BCF were nonsignificant. The computed α/β ranged from 1.3 to 11.1 Gy (4.9 ± 3.9 Gy; 95% CI: 1.6–8.2). On multivariate regression, %ADT was the sole determinant of computed α/β (model R2: 0.98, p < .001).
Conclusions: Clinically estimated α/β for PCa from isoeffective randomized trials using known variables in the linear-quadratic expression ranged between 1.3 and 11.1 Gy. The estimated α/β values were inversely related to %ADT usage, which should be considered when planning future RT dose-fractionation schedules.
Introduction
Prostate cancers (PCa) are reported to exhibit high-fractionation sensitivity to radiotherapy (RT) with low α/β values similar to those of late-responding normal tissues [Citation1–3]. This observation has led to a number of phase III trials that compared conventional radiotherapy (CRT) with hypofractionated radiotherapy (HRT) in PCa [Citation4–10]. Several authors report α/β values for PCa in the range of 1–1.8 Gy, which are even lower than the adjacent late-responding normal structures such as rectum (α/β ≈ 3–5 Gy) [Citation3,Citation11–15]. Moreover unlike head and neck cancers, PCa are generally regarded as slow growing tumors without significant tumor repopulation [Citation3,Citation13–15]. Thus, it is expected that HRT would improve both the therapeutic outcome and patients’ quality of life through the consequent reduction in the number of hospital visits [Citation14,Citation15].
The design of HRT schedules depends heavily on the selection of an appropriate α/β value. A survey of the literature indicates a wide range of suggested α/β values in PCa [Citation11,Citation16–18] (0.65–50.3 Gy [Citation17]). The α/β value of PCa has been debated since 1999 when Brenner and Hall [Citation11] first proposed an α/β value of 1.5 Gy. Despite the uncertainty, a ‘generic’ α/β value of 1.5 Gy for PCa is usually incorporated in the linear-quadratic (LQ) model to biologically equivalent HRT regimens [Citation11,Citation15].
According to the reviews by Dasu et al. [Citation18] and Oliveira et al. [Citation17], prior to 2007 the mean α/β was estimated to be 2.88 Gy [standard deviation (SD) = ±2.15] whilst from 2007 onwards, the mean α/β was computed as 2.48 Gy (SD = ±0.74). The assumptions made to derive these α/β values included (i) variable source data (trials with external RT alone, variable brachytherapy dose rates, CRT or HRT), (ii) choice of radiosensitivity parameters from prostate cell lines, (iii) assumptions in tumor repair kinetics and (iv) radiobiological parameters including potential doubling time, kickoff time, radiobiological effectiveness, α- and β-values from prostate cell lines and clonogen numbers. These assumptions indicated an inherent uncertainty in the α/β values when applied in the clinic [Citation16,Citation17]. Furthermore, the likely impact of tumor oxygenation, hormonal interventions, tumor heterogeneity and risk categorization could add further ambiguity to the choice of α/β value while designing HRT schedules [Citation14,Citation15,Citation19,Citation20]. With the recent publications of several large phase III trials randomized between HRT and CRT, the general assumption of a low α/β value for PCa has been questioned [Citation21,Citation22].
To avoid such ambiguities, α/β values in this study were individually estimated, by equating the biological effective doses (BED) using the LQ expression [Citation23], from clinically isoeffective randomized trials. These studies had reported non-significant differences in 5-year biochemical failure (BF) or biochemical and/or clinical failure (BCF) free rates. The estimated α/β values and their implications in designing RT dose-fractionation schedules were examined.
Material and methods
Selection of clinical trials
A PubMed search was conducted using the term ‘Prostate hypofractionated trial’ with no filters or restrictions. The search was last updated on 23 March 2017. Articles were shortlisted and full papers reviewed as per the PRISMA guidelines [Citation24], if they were (i) randomized trials with HRT versus CRT with external beam RT only (ii) BF or BCF rates at 5-years were scored using only Phoenix criteria [Citation25] and (iii) no significant difference was reported in BF or BCF at 5-years between the two arms. Along with this, relevant abstracts and publications at major oncology/radiation oncology conferences were hand-searched.
Computation of α/β values, BED and normalized total dose
As the outcomes of the two treatment arms of the trials [Citation4–10] were stated to be non-inferior or without significant differences with regard to BF or BCF, the HRT and CRT treatment arms within each trial were considered to be clinically isoeffective. Thus, assuming BEDCRT = BEDHRT, the RT dose parameters were used to derive the α/β value for each study using the BED expression of equality [Citation23],
(1)
where d and D indicate the RT dose/fraction and the total RT dose respectively, of the corresponding HRT and CRT schedules (represented by subscripts). As PCa is a slow growing tumor [Citation15,Citation18,Citation26], a time factor was not included in the calculations. Using the α/β values derived from each study and n as the number of RT fractions, the BED was computed for the corresponding HRT (BEDHRT) and CRT (BEDCRT) schedules [Citation15]:
(2)
BED for the HRT and CRT schedules were computed using the d and D of the two study arms assuming an α/β of 1.5 Gy [Citation4–6,Citation8,Citation10,Citation11] and were denoted as BEDHRT(α/β = 1.5) and BEDCRT(α/β = 1.5), respectively. The %difference in the two BEDs was computed as {(BEDHRT(α/β = 1.5)–BEDCRT(α/β = 1.5))/BEDCRT(α/β = 1.5)} × 100. To further estimate the equivalent total doses for HRT given in 2 Gy per fraction, the normalized total dose (NTD) for each study was estimated using α/β = 1.5 Gy [Citation15]:
(3)
Statistical analysis
A one-sample t-test was performed for the estimated α/β value while paired-sample t-tests were undertaken for the BED, overall treatment time (OTT) and NTD of the corresponding HRT and CRT schedules. A p value of ≤.05 was considered to be statistically significant. Scatterplots with a linear regression line and R2 with 95% confidence intervals (CI) were plotted wherever indicated.
A stepwise multivariate linear regression was performed including the constant for the estimated α/β value using independent variables of %patients on ADT and %patients in different risk categories. DHRT, DCRT, dHRT and dCRT were not incorporated in the regression model as these variables were used to estimate the α/β value. Probability of ‘F’ to enter the model was kept at ≤0.05 and removal at ≥0.10. The regression coefficient, 95% CI, model R2, adjusted model R2 and Durbin–Watson test were estimated. Regression plots for normal probability-plot (P-P) of regression standardized residual, and ZPRED (the standardized predicted values of the dependent variable based on model) versus ZRESID (the standardized residuals or errors) were computed to test for homoscedasticity. Statistical computations were conducted using IBM SPSS version 24 software (IBM Corporation, New York, NY, USA).
To further ascertain the collective impact of HRT versus CRT across the trials, a meta-analysis for the BF, BCF, overall mortality (OM) and PCa-specific mortality (PCaSM) was carried out using Comprehensive Meta-analysis Software (version 3.0) (Comprehensive-Meta-Analysis, Englewood, NJ, USA) [Citation27]. The odds ratio (OR), risk ratio (RR) and risk difference (RD) for each of the chosen endpoints were computed using the random-effects model and results are given as a point estimate, 95% CI, Z and p value. Heterogeneity was assessed using the I2 statistic while publication bias was evaluated by funnel plots and rank correlation test using Kendall’s tau [Citation28].
Results
Shortlisted isoeffective phase III randomized studies
One hundred and fifty six citations were identified, of which seven randomized studies fulfilled the selection criteria and were shortlisted for further analysis [Citation4–10]. Of these, one was available only in abstract form [Citation10]. However, additional study details were mined from other publications pertaining to the same patient cohort [Citation29]. Of the seven randomized studies, three were designed as non-inferiority while the remaining four were superiority trials (). The non-inferiority trials had varying non-inferiority margins (5–7.65%) and different hazard ratios (1.21–1.52) [Citation4,Citation6,Citation7]. Thus, the eligibility criterion for inclusion in our study was not based on the non-inferiority or superiority design of the trials but on non-significant differences in BF or BCF at 5-years between CRT and HRT.
Table 1. Summary of the patient characteristics and outcomes of the eight randomized trial arms from seven clinically isoeffectiveTable Footnote† studies included in the study.
Dearnaley et al. [Citation7] reported that the 60 Gy HRT arm was non-inferior to the 74 Gy CRT arm. The authors concluded that non-inferiority of the 57 Gy HRT arm could not be claimed. However, as both the 60 and 57 Gy HRT arms of the above trial showed no significant difference in BCF to the CRT arm, these were considered separately in the present study. The studies by Yeoh et al. [Citation30] and Lukka et al. [Citation31] were not considered as they did not meet the inclusion criteria. The former trial was reported to be isoeffective at 5-years for HRT versus CRT according to the American Society for Therapeutic Radiology and Oncology (ASTRO) rather than the Phoenix criteria and was thereby excluded [Citation30]. The updated outcome at 7.5 years was based on Phoenix criteria but showed a therapeutic advantage for HRT [Citation32]. Lukka et al. [Citation31] reported BCF to be significantly better with CRT as per the ASTRO criteria and consequently this study was also omitted. Eight comparative trial arms from seven studies were finally included in this study.
A total of 6993 patients were treated by CRT (n = 2941) or HRT (n = 4052) in these eight trials. The trials reported by Catton et al. [Citation4], Lee et al. [Citation6], Dearnaley et al. [Citation7] and Incrocci et al. [Citation8] are referred to in the text with acronyms PROFIT, RTOG 0415, CHHiP and HYPRO, respectively.
All patients were treated by either intensity-modulated radiation therapy or 3D RT to the prostate with varying volumes of seminal vesicle (Supplementary Table S1). Studies were categorized into various risk groups based on T stage, Gleason score and prostate-specific antigen (PSA) levels. PROFIT [Citation4], RTOG 0415 [Citation6], CHHiP [Citation7] and Kuban et al. [Citation10,Citation29] defined risk groups as per the National Comprehensive Cancer Network (NCCN) guidelines. HYPRO [Citation8] allocated risk according to the single or double-factor high-risk model proposed by Chism et al. [Citation33]. Arcangeli et al. [Citation5] and Pollack et al. [Citation9] described the criteria for patient risk classification without citing specific guidelines ().
The total RT dose used in the CRT arms ranged from 73.8 to 80 Gy (mean ± SD: 76.2 ± 2.3, p < .001) at a dose per fraction of 1.8–2 Gy (1.9 ± 0.1, p < .001) while the corresponding RT doses and dose per fraction for HRT were 57–72 Gy (64.5 ± 5.6, p < .001) and 2.4–3.4 Gy (2.9 ± 0.3, p < .001) (). The dose per fraction and the total RT dose for both CRT and HRT were significantly different (dose per fraction, p < .001; total RT dose, p = .001). The OTT varied between 25 and 43 days (33.6 ± 7.3, p < .001) for HRT and ranged from 51 to 58 days (53.6 ± 2.6, p < .001) for CRT. The difference in OTT between HRT and CRT was also significant (p < .001).
Table 2. Details of the eight randomized trial arms from seven studies between CRT and HRT along with their computed α/β values and the corresponding BED.
All studies included used the Phoenix criteria for assessment of BF at 5-years [Citation25]. They reported non-significant differences in BF or BCF or both for CRT versus HRT. Five trials had similar BF [Citation4–6,Citation8,Citation10] while six reported comparable BCF rates (includes both arms of the CHHiP trial) [Citation4,Citation7–10] between the HRT and CRT arms. Both BF and BCF rates were reported in three trials [Citation4,Citation8,Citation10]. Thus, all studies were individually clinically isoeffective for the endpoint of BF and/or BCF. This allowed computation of the α/β of each study using the EquationEquation (1)(2) .
Furthermore, the meta-analysis revealed no significant differences in RD for BF (RD: −0.020, p: ns), BCF (RD: −0.006, p: ns), OM (RD: −0.008, p: ns) and PCaSM (RD: −0.002, p: ns) (Supplementary Figures S1(a–d)). The same was also observed for OR and RR (Supplementary Table S2) and no significant heterogeneity or publication bias was evident.
Computation of α/β values, BED and NTD (2 Gy)
The estimated α/β from these studies ranged between 1.3 and 11.1 Gy (4.9 ± 3.9 Gy, p = .010). The weighted mean of the estimated α/β was 3.7 Gy. There was a significant difference (δ) in the %BEDs of the BEDCRT(α/β = 1.5) and BEDHRT(α/β = 1.5) (δ range = −1.1 to 15.9%, 7.3 ± 7.1%, p = .023, ). BEDCRT(α/β = 1.5) and BEDHRT(α/β = 1.5) displayed no correlation (Pearson r = 0.33, p: ns, ). Only in PROFIT [Citation4] were the BED values for HRT and CRT protocols nearly identical (BEDHRT(α/β = 1.5) = 180 Gy and BEDCRT(α/β = 1.5) = 182 Gy). Using the derived α/β values, the computed BEDs were similar for both HRT and CRT for all trials and varied between 85.7 Gy(estimated α/β) and 195 Gy(estimated α/β) (133.9 ± 43.9 Gy(estimated α/β), ).
Figure 1. No correlation is evident between BED for CRT and HRT schedules from the isoeffective trials. BED has been estimated assuming α/β = 1.5 Gy (Pearson correlation, r = 0.33, p: ns).
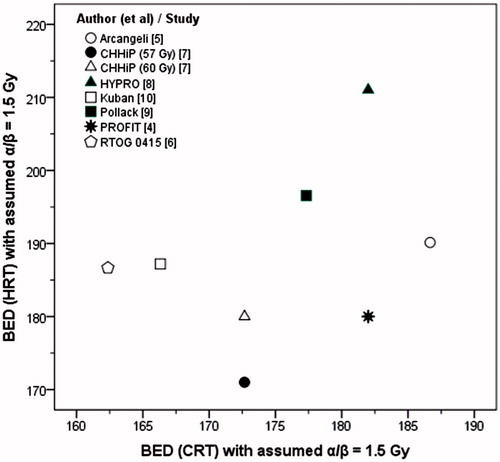
The NTD (2 Gy) for HRT schedules computed using the α/β value of 1.5 Gy, ranged between 73.4 and 90.6 Gy1.5 (80.6 ± 5.2). As two of the CRT protocols [Citation6,Citation10] used 1.8 Gy/fraction, the corrected corresponding CRT doses at 2 Gy per fraction were between 69.6 and 80 Gy (75.1 ± 3.5). The differences between NTD (2 Gy) of HRT and the CRT were statistically significant (p = .021).
Estimated α/β values and %patients on ADT
The %patients on ADT varied between 0 and 100% in these trials. One study in low-risk patients did not prescribe ADT [Citation6] whilst a variable number of patients received ADT in the other studies ( and ). The PROFIT study [Citation4] did not permit ADT concurrently with RT, but patients who received up to 12 weeks of hormone therapy prior to RT were included in the study. CHHiP [Citation7] prescribed three to 6 months short course ADT before and during RT and around 97% of the patients in each of the three arms (CRT, HRT 57 Gy and HRT 60 Gy) received ADT, which was optional for low-risk patients. In HYPRO [Citation8], around two-thirds of patients in each group were prescribed ADT at the discretion of the investigators. 39.8% of the patients received ADT for at least 25 months. Nearly half of the patients (CRT: 46.7%; HRT: 45%) treated by Pollack et al. [Citation9] received ADT. This was either short-term up to 4 months for the intermediate-risk group (13.2% CRT; 9.9% HRT) or long-term (2-years) for the high-risk group (33.5% CRT; 35.1% HRT). All patients in the randomized trial reported by Arcangeli et al. [Citation5] received ADT which was started before the onset of RT (Day 0–270 with bicalutamide, RT from Day 67) and continued with luteinizing hormone-releasing hormone analog depot at Days 7, 97 and 187. Kuban et al. [Citation10] reported that 22.5 and 19.6% of the CRT and HRT patients received 4 months of ADT.
The estimated α/β values were lower in trials that had higher %patients on ADT. In the PROFIT study [Citation4], with 5.6% patients on ADT, the estimated α/β value was 1.3 Gy, well below the 95% CI (1.6–8.2) with R2 of 0.42 (Pearson r = −0.66, p: ns) (). This appeared to be in discordance with the data from other studies [Citation5–10] and the possible reasons for this observation are discussed in the next section. A linear regression of the remaining seven trial arms showed an improved line fit of R2 of 0.98 (Pearson r = −0.99, p < .001) (Supplementary Figure S2).
Multivariate regression for estimated α/β
When all eight trials were included, only ‘%patients in the low-risk category’ was retained by the model for the dependent variable ‘estimated α/β value’ (B = 0.08, p = .05, model R2 = 0.51) (Supplementary Table S3). The derived model estimated the α/β value as 3.25 + 0.08x (%low-risk patients). The residuals ranged from −3.15 to +4.53 (mean ± SD: 0.00 ± 2.78). For the CHHiP HRT 57 Gy arm [Citation7], the predicted α/β with the above model was 4.5 Gy compared to the estimated value of 1.3 Gy. At the other extreme, the model predicted and estimated α/β value for Kuban et al. [Citation10] of 5.7 and 10.2 Gy, respectively.
As the estimated α/β value of 1.3 Gy for the PROFIT study was outside the 95% CI and discordant with values from other trials with respect to ‘% patients on ADT’, the multivariate regression was repeated excluding this study [Citation4]. With the seven remaining trial arms, the model retained only ‘%patients on ADT’ as the sole predictor (B = −0.10, p < .001, model R2 = 0.98) (). The revised model estimated the α/β value to be 11.45–0.10x (%patients on ADT) with much lower residuals of −0.53 to 0.81 (mean ± SD: 0.00 ± 0.50). Thus using this model, the predicted and estimated α/β values for CHHiP HRT 57 Gy [Citation7] were 1.9 and 1.4 Gy, respectively. The same was also evident for Kuban et al. [Citation10] (predicted versus estimated α/β as 9.4 versus 10.2 Gy). The ZPRED versus ZRESID plots for residuals indicated that the assumptions of random errors and homoscedasticity had been satisfactorily met with this revised model [Supplementary Figure S3(a,b)].
Table 3. Stepwise multivariate regression for the computed α/β values (dependent variable) for the randomized trials between conventional fractionated and hypofractionated RT.
Discussion
External beam RT, brachytherapy and, more recently, stereotactic body RT are used to irradiate localized or locally advanced PCa. With the widespread acceptance of α/β of 1.5 Gy for PCa, a number of studies have explored HRT to improve the therapeutic outcome and reduce OTT. The computation of an HRT schedule with the same biological effectiveness as the CRT arm requires the best estimate of the true α/β value. In this study, the LQ expression was used to compute α/β values from eight isoeffective trial arms with two different total RT doses and dose per fraction and avoided the assumption of values for other radiobiological parameters.
For the computation of NTD (2 Gy), only α/β is assumed as dHRT and DHRT are known. Fowler et al. [Citation15] derived various equivalent NTD values for HRT which could be considered equivalent to a 2 Gy per fraction CRT regimen in PCa, assuming α/β to be 1.5 Gy. However, they did caution that these were based on theoretical LQ modeling for existing clinical data for intermediate-risk PCa patients [Citation15]. They also stated that the generic use of α/β = 1.5 Gy for PCa did not take into account other fractionation-related phenomena that might play a part in the clinical setting. Neither was the possible impact of ADT on α/β values considered.
On computing the individual NTD values for each of these isoeffective studies as described above, using α/β of 1.5 Gy, the NTD (2 Gy) of HRT and the total dose of CRT were significantly different (p = .021) and no correlation was observed (r = 0.33, p: ns, , R2 = 0.11). This amounts to a contradiction of the very basis of the NTD concept. Of the eight trial arms, only three had a NTD (2 Gy) for HRT similar to that of the corresponding CRT when computed with an α/β of 1.5 Gy: PROFIT [Citation4], Arcangeli et al. [Citation5] and 57 Gy HRT arm of CHHiP [Citation7] (). The estimated α/β in these were 1.3 [Citation4], 1.8 [Citation5] and 1.4 Gy [Citation7]. The lack of overall correlation between the NTD values of HRT and CRT computed with α/β = 1.5 Gy, further indicates that use of a generic α/β of 1.5 Gy for PCa needs reconsideration when applied in clinical situations.
Figure 3. NTD at 2 Gy for HRT versus the NTD at 2 Gy for CRT regimes. The NTDs have been computed using α/β = 1.5 Gy.
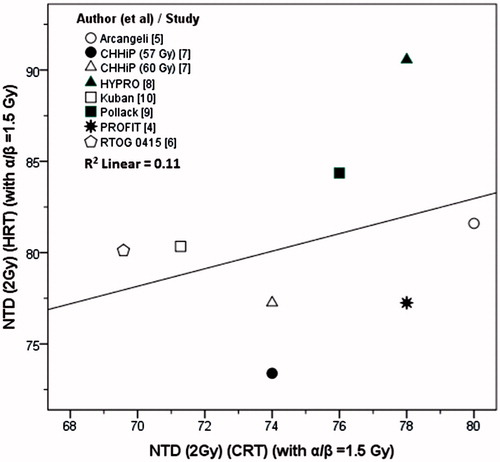
A higher BED would be expected to result in a higher %freedom from BF. In their meta-analysis of 12,756 PCa patients across all risk categories, Zaorsky et al. [Citation34] showed that between 140 and 200 Gy, an increment of 10 Gy in BED (α/β = 1.5 Gy) was associated with a five unit improvement in 5-year freedom from BF. A closer perusal of the BF and BCF rates at 5-years shows that the BF or BCF achieved with HRT or CRT in most of these eight trials was high, in the range of at least 80–90% and even beyond. For instance, RTOG 0415 [Citation6] reported the 5-year BF with CRT as 91.9 versus 93.7% with HRT. Thus, the higher BED of 14.9% (+24.3 Gy1.5 in absolute terms) with BEDHRT(α/β = 1.5) in RTOG 0415 cannot realistically result in an improvement in freedom from BF by more than 12% beyond 93.7% in the HRT arm. Similarly, Kuban et al. [Citation10] reported a 5-year BCF in the range of 94–97% in the CRT and HRT treatment arms. So, even if the BEDHRT(α/β = 1.5) was higher in the HRT arm by 12.5% in Kuban et al. [Citation10], a further gain in terms of BCF cannot be expected in the HRT arm. These studies raise further questions about the assumption of a constant α/β of 1.5 Gy in all PCa tumors.
To further explore this point, we examined the curves from Zaorsky et al. [Citation34] plotted between the saturation BED and the corresponding α/β values (ranging from 1.5 to 10 Gy) to achieve the optimal freedom from BF. Since our estimated α/β varied from 1.3 to 11.1 Gy, it provided us with an opportunity to compare the impact of BED computed using our estimated α/β value with the corresponding saturation BED values for the identical α/β computed by Zaorsky et al. [Citation34].
The BEDs for both CRT and HRT of these studies ranged between 85.7 and 195 Gy (). Extrapolating the two ends of the plots for ‘HFRT monotherapy’ and ‘DE-CFRT monotherapy’ ( from Zaorsky et al. [Citation34]) for α/β from 1.3 to 1.5 Gy and 10 to 11 Gy, the BED values computed using the estimated α/β (varying from 1.3 to 11.1 Gy) showed a close correlation with those plotted by Zaorsky et al. (r = 0.99, p < .001). A scatter plot yielded an R2 of 0.99 between the two BEDs (). This indicated that, although there was a high likelihood of saturation in the BF or BCF being reached by increasing the BED, the corresponding saturated BED depended on the actual α/β values, which were different from 1.5 Gy. The saturation value of a BED of 200 Gy, as proposed by Zaorsky et al. [Citation34] for nearly all risk categories, would be applicable only if the α/β for all PCa is assumed to be 1.5 Gy. Our study showed the α/β to vary between 1.3 and 11.1 Gy and hence the saturation BED value would be different for each case. This corroborated our concern of assuming a uniform α/β of 1.5 Gy for all patients with PCa.
Figure 4. Plots between the BED computed using the estimated α/β for prostate in the present studies and the BED computed by Zaorsky et al. [Citation34] for a range of α/β values to indicate the saturation values of BED for RT dose escalation and altered fractionation regimes. The 95% CI is shown in dotted lines.
![Figure 4. Plots between the BED computed using the estimated α/β for prostate in the present studies and the BED computed by Zaorsky et al. [Citation34] for a range of α/β values to indicate the saturation values of BED for RT dose escalation and altered fractionation regimes. The 95% CI is shown in dotted lines.](/cms/asset/6e558656-c8c3-4c68-95db-facb0d523298/ionc_a_1433874_f0004_c.jpg)
Gulliford et al. [Citation35] have recently estimated the α/β values for PCa using the data from four of the seven randomized trials—PROFIT [Citation4], RTOG 0415 [Citation6], CHHiP [Citation7] and HYPRO [Citation8] and their findings are presently available only in abstract form. Their estimated α/β values, using the best fit parameters with a time factor correction, ranged between 3.8 and 31.5 Gy. Without consideration of the time factor, α/β varied from 1.3 to 6.9 Gy. In agreement with our findings, the lowest values obtained were from PROFIT [Citation4] (1.3 Gy exactly matched our estimate) while the highest values were derived from RTOG 0415 [Citation6]. The trend was similar to that observed in the present study where α/β ranged between 1.3 and 11.1 Gy. We did not assume values for any variables but instead used the isoeffective concept with given values of the variables in the LQ equation. They used a grid search approach and repopulation was included in their model, assuming a proliferation rate of 0.31 Gy/day. The exact α/β values were dissimilar due to a difference in the computation methods. Nonetheless both studies highlighted that the assumption of a universal α/β value of 1.5 Gy in PCa may need reconsideration.
These eight isoeffective trial arms included patients in different risk categories and the %patients receiving ADT varied from 0 to 100%. The value of estimated α/β reduced as the proportion of patients on ADT increased. This trend was apparent when all studies were included and was even more marked by excluding the PROFIT study [Citation4]. On including the PROFIT study, the regression line was less steep and the R2 dropped from 0.98 to 0.42 ( and Supplementary Figure S2). PROFIT was the only study where all patients were in the intermediate-risk group. Although synchronous ADT with RT was not permitted, only those with more than 12 weeks of ADT prior to RT were considered ineligible for the PROFIT study. The estimated α/β of 1.3 Gy was discordant with the data from the remaining seven trial arms with respect to corresponding %patients on ADT. Possible reasons include the heterogeneity of the intermediate-risk group and the consequent range in prognostic outcomes in their various subsets, which could influence the impact of ADT [Citation36,Citation37]. Furthermore, as the study spanned 27 centers across three continents and permitted ADT up to 12 weeks before RT, it could be possible that some additional patients might have been inadvertently initiated on ADT prior to enrollment in the study. Either or both of these mitigating factors could have contributed to the low estimated α/β value of 1.3 Gy despite only 5.6% patients having formally received ADT. This value was incongruous with the observations from other trials. For example, in the 57 Gy HRT arm of CHHiP trial, the estimated α/β value of 1.3 Gy was derived with 97% patients on ADT.
Our estimated α/β of 1.3 Gy for the PROFIT study matches exactly with the estimates without a time factor reported by Gulliford et al. [Citation35]. All computations with the estimated α/β of 1.3 Gy for the PROFIT study are in line with the NTD (2 Gy) and the saturation BED for HRT as proposed by Zaorsky et al. [Citation34] and discussed earlier. The mismatch was only with respect to the relationship between %patients on ADT and the estimated α/β of the PROFIT study, the likely reasons for which have been stated above.
The regression model for estimated α/β including the PROFIT study demonstrated a poor fit (Supplementary Table S3). Only ‘% patients in the low-risk category’ emerged as a predictive variable. The resultant model had high residuals. However, the model improved considerably on excluding the PROFIT study, indicating that only %patients on ADT predicted the estimated α/β values, with a lower α/β from studies where most patients received ADT (, Supplementary Figure S2). This indicated a possible influence of ADT on prostate tumor cytokinetics resulting in a reduction of the α/β value.
Androgen receptor-mediated signaling has been shown to induce androgen-dependent cell proliferation by acting as a key regulator of G1–S cell cycle phase progression, promoting G1 cycle-dependent kinase activity and inducing phosphorylation and thus inactivation of the retinoblastoma tumor suppressor [Citation38]. ADT thus induces tumor growth arrest which has been shown through the reduction in Ki-67 proliferative index, a fall in cyclin D1 expression, a reduction of cells in S-phase, G1/G0 phase arrest, an increase in p53 and p21 levels and consequent increase in apoptosis [Citation38–41]. The above processes could sequentially inhibit tumor growth kinetics of androgen-dependent tumors and cause them to mimic radiobiologically slow growing, late-responding normal tissues with low α/β values (). This is a compelling explanation of the apparent influence of ADT on lowering α/β values in PCa patients.
Figure 5. A possible mechanism to explain the impact of ADT on androgen-dependent PCa cells. The resulting alteration in PCa cell kinetics mimics late reacting tissue with a lower α/β.
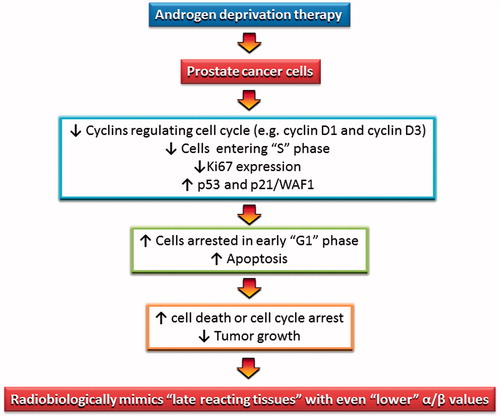
Miralbell et al. [Citation14] reported a α/β of 1.4 Gy for all risk categories of PCa, independent of ADT use. This is in contrast to our observation where use of ADT strongly influenced α/β. This could be ascribed to differences in the methodology of α/β estimations. They used tumor control probabilities and considered different models with the key variables of natural log of effective target cell number, α and β/α which were allowed to vary between risk categories and ADT status to select the most ‘optimum’ predictive model. Thus, the α/β was estimated by varying the above variables to select the most optimal model according to the Akaike information criteria and model fitting [Citation14]. Unlike some of the parameters used in the above model, the α/β values estimated in our study are based on given EBRT parameters of the HRT and CRT arms from the isoeffective randomized trials. Our approach eliminated the need to assume values of unknown radiobiological parameters.
Following the publication of these seven large studies randomized between HRT and CRT, Høyer [Citation21] expressed concern regarding the uniform assumption of low α/β values in PCa. It was argued that the earlier trials were carried out in accordance with modeling studies which had indicated high-fractionation sensitivity, whilst the results from the recent large trials suggested that the α/β value could be moderately or considerably higher than the assumed value of 1.5 Gy [Citation21]. Høyer [Citation21] noted that these inconsistencies were unexplained and could be attributed to the differences in risk groups, Gleason score and use of ADT in these studies. Our computations indicate a variable α/β (1.3–11.1, 95% CI: 1.7–9.1) according to the ADT usage. The wide 95% CI of the estimated α/β values could also reflect the pattern of %ADT usage in these studies, which also varied from 0 to 100%.
PCa has been considered to have negligible repopulation and hence an insignificant time factor is assumed during the design of RT protocols [Citation15,Citation18,Citation26]. This is consistent with the assumption of a low α/β value of 1.5 Gy, typical of late-responding tissues. However, if the α/β was as high as 11.1 Gy, as estimated for the RTOG 0415 study [Citation6] (), one could expect an influence of OTT similar to other tumors with similar high α/β values (e.g., head and neck cancers). All patients included in the above study were in a low-risk category and none had received ADT. Gulliford et al. [Citation35] reported the highest α/β of 31.5 Gy for RTOG 0415 [Citation6] using best fit parameters and 6.9 Gy when no time factor was incorporated. Both our estimates and those from Gulliford et al. [Citation35] pointed towards the highest α/β values in the low-risk group who are irradiated without ADT. If these low-risk tumors indeed have higher α/β values without ADT, the impact of OTT would need to be considered as in the case of head and neck cancer.
In a large retrospective analysis of 4338 patients treated in nine leading US cancer centers, Thames et al. [Citation26] reported a significant influence of OTT in patients receiving ≥70 Gy in low and intermediate-risk groups but not in high-risk patients. The significance of a time factor might have been evident in this cohort of patients because none of the patients received neoadjuvant or adjuvant androgen suppression. Thus, although Thames et al. [Citation26] indicated a time factor effect in a selected patient population, this may not be applicable to the patients in our analysis as they belonged to all risk groups and had varying use of ADT. The only comparable group was the patients treated in RTOG 0415 [Citation6].
The dose equivalence of proliferation estimated by Thames et al. [Citation26] was 0.20 Gy/day for the low-risk group and 0.35 Gy/day for the intermediate-risk group. Although this was much less than 0.80 Gy/day for head and neck cancers [Citation42], a statistically significant difference in the BF failure rates was only evident in low (p = .11) and intermediate-risk group (p = .0005) patients for OTT of < 52 versus ≥52 days [Citation26]. Since these estimates were from a retrospective analysis, they need cautious interpretation. There is some similarity with the observation reported earlier by Hamsen et al. [Citation43] in head and neck cancer, where the impact of prolongation of OTT was only seen in well to moderately differentiated tumors. This was ascribed to the loss of accelerated repopulation in dedifferentiated tumors [Citation43]. Whether a similar phenomenon also exists in PCa requires further evaluation and is beyond the scope of the present study.
As the α/β values were computed from the reported summary data of these trials, it was not possible to exactly assess the duration of ADT in individual cases. Nevertheless, these isoeffective studies provided a unique opportunity to quantify directly the α/β values for each study using the widely accepted concept of BED (EquationEquation (1)(2) ) without making any assumptions as to various parameters (radiosensitivity, tumor repair kinetics, radiobiological parameters). Ideally, one would look at individual patient data along with the exact details of ADT administration, risk categories, Gleason scores, PSA levels and OTT to consolidate the impression of the impact of ADT on α/β values.
The potential influence of ADT on the radiation sensitivity of PCa should be considered when computing equivalent BEDs in future clinical trials. ADT reduces the α/β values in PCa and thereby could improve therapeutic outcomes by potentially reducing the α/β value below that of the rectum. Additionally, as evident on a recent meta-analysis, neoadjuvant ADT could also minimize the RT target volume by inducing prostate shrinkage, especially in the high-risk group, and thus mitigate the incidence of acute gastro-intestinal toxicities [Citation44]. Although ADT is not usually advocated for low-risk patients or routinely for all intermediate-risk group patients, the possibility of short course neoadjuvant and concurrent ADT could be explored with RT in these risk groups too. This could not only lower the α/β value, but may help to counter the tumor repopulation as reported in low- and intermediate-risk groups [Citation26]. Furthermore, a relatively short course of ADT would minimize the side effects associated with long-term ADT administration. These should be evaluated in well-designed randomized studies for such patients to further optimize treatment strategies.
Currently, extreme HRT consisting of four-to-five sessions of 6–10 Gy fractions are being explored in PCa [Citation2,Citation45]. The outcomes of these trials will help address concerns as to the validity of the present LQ model for such extreme HRT schedules [Citation22,Citation46,Citation47].
In conclusion, the computed α/β values from isoeffective trials using the LQ expression from eight trials totaling 6993 patients demonstrates a need to move from a ‘generic’ value of 1.5 Gy to a ‘clinically derived realistic’ estimate in PCa. The prevailing ‘one-size-fits-all’ assumption of α/β of 1.5 Gy in PCa may need reconsideration in clinical situations and the impact of ADT on α/β values needs closer evaluation. Lennernäs et al. [Citation48] suggested a growing need to emphasize the importance of radiobiology in addition to the existing four-dimensional (4D) RT to move towards a comprehensive five-dimensional (5D) RT. To realize truly a 5D-RT in PCa, the use of ADT with its potential to impact radiobiology, may need to be taken into account.
Niloy_R._Datta_et_al._Supplementary_Tables_and_Figures.pdf
Download PDF (670.3 KB)Disclosure statement
No potential conflict of interest was reported by the authors.
Additional information
Funding
References
- Hocht S, Aebersold DM, Albrecht C, et al. Hypofractionated radiotherapy for localized prostate cancer. Strahlenther Onkol. 2017;193:1–12.
- Arcangeli S, Greco C. Hypofractionated radiotherapy for organ-confined prostate cancer: is less more? Nat Rev Urol. 2016;13:400–408.
- Dasu A, Toma-Dasu I. Prostate alpha/beta revisited – an analysis of clinical results from 14 168 patients. Acta Oncol. 2012;51:963–974.
- Catton CN, Lukka H, Gu CS, et al. Randomized trial of a hypofractionated radiation regimen for the treatment of localized prostate cancer. J Clin Oncol. 2017;35:1884–1890.
- Arcangeli G, Saracino B, Arcangeli S, et al. Moderate hypofractionation in high-risk, organ-confined prostate cancer: final results of a phase III randomized trial. J Clin Oncol. 2017;35:1891–1897.
- Lee WR, Dignam JJ, Amin MB, et al. Randomized phase III noninferiority study comparing two radiotherapy fractionation schedules in patients with low-risk prostate cancer. JCO. 2016;34:2325–2332.
- Dearnaley D, Syndikus I, Mossop H, et al. Conventional versus hypofractionated high-dose intensity-modulated radiotherapy for prostate cancer: 5-year outcomes of the randomised, non-inferiority, phase 3 CHHiP trial. Lancet Oncol. 2016;17:1047–1060.
- Incrocci L, Wortel RC, Alemayehu WG, et al. Hypofractionated versus conventionally fractionated radiotherapy for patients with localised prostate cancer (HYPRO): final efficacy results from a randomised, multicentre, open-label, phase 3 trial. Lancet Oncol. 2016;17:1061–1069.
- Pollack A, Walker G, Horwitz EM, et al. Randomized trial of hypofractionated external-beam radiotherapy for prostate cancer. J Clin Oncol. 2013;31:3860–3868.
- Kuban DA, Nogueras-Gonzalez GM, Hamblin L, et al. Preliminary report of a randomized dose escalation trial for prostate cancer using hypofractionation. Int J Radiat Oncol Biol Phys. 2010;78:S58–S59.
- Brenner DJ, Hall EJ. Fractionation and protraction for radiotherapy of prostate carcinoma. Int J Radiat Oncol Biol Phys. 1999;43:1095–1101.
- Bentzen SM, Ritter MA. The alpha/beta ratio for prostate cancer: what is it, really? Radiother Oncol. 2005;76:1–3.
- Fowler JF. The radiobiology of prostate cancer including new aspects of fractionated radiotherapy. Acta Oncol. 2005;44:265–276.
- Miralbell R, Roberts SA, Zubizarreta E, et al. Dose-fractionation sensitivity of prostate cancer deduced from radiotherapy outcomes of 5,969 patients in seven international institutional datasets: α/β = 1.4 (0.9–2.2) Gy. Int J Radiat Oncol Biol Phys. 2012;82:e17–e24.
- Fowler JF, Ritter MA, Chappell RJ, et al. What hypofractionated protocols should be tested for prostate cancer? Int J Radiat Oncol Biol Phys. 2003;56:1093–1104.
- Shaffer R, Pickles T, Lee R, et al. Deriving prostate alpha-beta ratio using carefully matched groups, long follow-up and the phoenix definition of biochemical failure. Int J Radiat Oncol Biol Phys. 2011;79:1029–1036.
- Oliveira SM, Teixeira NJ, Fernandes L. What do we know about the alpha/beta for prostate cancer? Med Phys. 2012;39:3189–3201.
- Dasu A. Is the alpha/beta value for prostate tumours low enough to be safely used in clinical trials? Clin Oncol (R Coll Radiol). 2007;19:289–301.
- Ray KJ, Sibson NR, Kiltie AE. Treatment of breast and prostate cancer by hypofractionated radiotherapy: potential risks and benefits. Clin Oncol (R Coll Radiol). 2015;27:420–426.
- Lennernas B, Nilsson S, Levitt S. Hypofractionation for radiotherapy of prostate cancer using a low alfa/beta ratio–possible reasons for concerns? An example of five dimensional radiotherapy. Acta Oncol. 2011;50(Suppl. 1):111–115.
- Høyer M. What does large randomized trials tell us about the fractionation sensitivity of prostate cancer? J Phys Conf Ser. 2017;777:012024. Available from: http://iopscience.iop.org/article/10.1088/1742-6596/777/1/012024
- Cosset JM. [Hypofractionated irradiation of prostate cancer: what is the radiobiological understanding in 2017?]. Cancer Radiother. 2017;21:447–453.
- Fowler JF, Ritter MA. A rationale for fractionation for slowly proliferating tumors such as prostatic adenocarcinoma. Int J Radiat Oncol Biol Phys. 1995;32:521–529.
- Liberati A, Altman DG, Tetzlaff J, et al. The PRISMA statement for reporting systematic reviews and meta-analyses of studies that evaluate healthcare interventions: explanation and elaboration. BMJ. 2009;339:b2700.
- Roach M III, Hanks G, Thames H Jr, et al. Defining biochemical failure following radiotherapy with or without hormonal therapy in men with clinically localized prostate cancer: recommendations of the RTOG-ASTRO Phoenix Consensus Conference. Int J Radiat Oncol Biol Phys. 2006;65:965–974.
- Thames HD, Kuban D, Levy LB, et al. The role of overall treatment time in the outcome of radiotherapy of prostate cancer: an analysis of biochemical failure in 4839 men treated between 1987 and 1995. Radiother Oncol. 2010;96:6–12.
- Comprehensive Meta-Analysis [Internet]. Comprehensive Meta-Analysis Software, version 3.0. 2016 [Aug 8.]. Englewood, NJ: Biostat. Available from: https://www.meta-analysis.com/
- Begg CB, Mazumdar M. Operating characteristics of a rank correlation test for publication bias. Biometrics. 1994;50:1088–1101.
- Hoffman KE, Voong KR, Pugh TJ, et al. Risk of late toxicity in men receiving dose-escalated hypofractionated intensity modulated prostate radiation therapy: results from a randomized trial. Int J Radiat Oncol Biol Phys. 2014;88:1074–1084.
- Yeoh EE, Holloway RH, Fraser RJ, et al. Hypofractionated versus conventionally fractionated radiation therapy for prostate carcinoma: updated results of a phase III randomized trial. Int J Radiat Oncol Biol Phys. 2006;66:1072–1083.
- Lukka H, Hayter C, Julian JA, et al. Randomized trial comparing two fractionation schedules for patients with localized prostate cancer. JCO. 2005;23:6132–6138.
- Yeoh EE, Botten RJ, Butters J, et al. Hypofractionated versus conventionally fractionated radiotherapy for prostate carcinoma: final results of phase III randomized trial. Int J Radiat Oncol Biol Phys. 2011;81:1271–1278.
- Chism DB, Hanlon AL, Horwitz EM, et al. A comparison of the single and double factor high-risk models for risk assignment of prostate cancer treated with 3D conformal radiotherapy. Int J Radiat Oncol Biol Phys. 2004;59:380–385.
- Zaorsky NG, Palmer JD, Hurwitz MD, et al. What is the ideal radiotherapy dose to treat prostate cancer? A meta-analysis of biologically equivalent dose escalation. Radiother Oncol. 2015;115:295–300.
- Gulliford S, Griffin C, Tree A, et al. Estimates of the α/β ratio for prostate using data from recent hypofractionated RT trials. Radiother Oncol. 2017;123:S871–S872.
- Zumsteg ZS, Zelefsky MJ. Short-term androgen deprivation therapy for patients with intermediate-risk prostate cancer undergoing dose-escalated radiotherapy: the standard of care? Lancet Oncol. 2012;13:e259–e269.
- Castle KO, Hoffman KE, Levy LB, et al. Is androgen deprivation therapy necessary in all intermediate-risk prostate cancer patients treated in the dose escalation era? Int J Radiat Oncol Biol Phys. 2013;85:693–699.
- Balk SP, Knudsen KE. AR, the cell cycle, and prostate cancer. Nucl Recept Signal. 2008;6:e001.
- Munoz-Moreno L, Arenas MI, Carmena MJ, et al. Anti-proliferative and pro-apoptotic effects of GHRH antagonists in prostate cancer. Oncotarget. 2016;7:52195–52206.
- Katzenwadel A, Wolf P. Androgen deprivation of prostate cancer: leading to a therapeutic dead end. Cancer Lett. 2015;367:12–17.
- Ardiani A, Gameiro SR, Kwilas AR, et al. Androgen deprivation therapy sensitizes prostate cancer cells to T-cell killing through androgen receptor dependent modulation of the apoptotic pathway. Oncotarget. 2014;5:9335–9348.
- Roberts SA, Hendry JH, Brewster AE, et al. The influence of radiotherapy treatment time on the control of laryngeal cancer: a direct analysis of data from two British Institute of Radiology trials to calculate the lag period and the time factor. Br J Radiol. 1994;67:790–794.
- Hansen O, Overgaard J, Hansen HS, et al. Importance of overall treatment time for the outcome of radiotherapy of advanced head and neck carcinoma: dependency on tumor differentiation. Radiother Oncol. 1997;43:47–51.
- Datta NR, Stutz E, Rogers S, et al. Conventional versus hypofractionated radiation therapy for localized or locally advanced prostate cancer: a systematic review and meta-analysis along with therapeutic implications. Int J Radiat Oncol Biol Phys. 2017;99:573–589.
- De Bari B, Arcangeli S, Ciardo D, et al. Extreme hypofractionation for early prostate cancer: biology meets technology. Cancer Treat Rev. 2016;50:48–60.
- Nahum AE. The radiobiology of hypofractionation. Clin Oncol (R Coll Radiol). 2015;27:260–269.
- Park C, Papiez L, Zhang S, et al. Universal survival curve and single fraction equivalent dose: useful tools in understanding potency of ablative radiotherapy. Int J Radiat Oncol Biol Phys. 2008;70:847–852.
- Lennernas B, Castellanos E, Nilsson S, et al. Four and five dimensional radiotherapy with reference to prostate cancer–definitions, state of the art and further directions–an overview. Acta Oncol. 2011;50(Suppl. 1):104–110.