Abstract
Background and purpose: Traditionally, elastase has been used to study exocrine activity of the pancreas in patients with chronic pancreatitis and cystic fibrosis, and calprotectin as a marker for gut-wall inflammation in patients with inflammatory bowel disease. The aim of the study was to find out whether elastase and calprotectin could be used as inflammatory markers for radiation-induced gut wall injury of the distal bowel.
Material and methods: Adult male mice were exposed to two, three, or four fractions of 6 Gy or 8 Gy irradiation to the sigmoid and rectum of the large bowel, using a linear accelerator. Fecal samples were collected from mice at 1, 3, and 6 weeks post-irradiation. The fecal levels of elastase and calprotectin were analyzed using ELISA.
Results: Three and 6 weeks after irradiation, we found a dose–effect relationship between dose of ionizing radiation and the fecal level of elastase; that is significantly higher levels of elastase were observed in mice that had received a high irradiation dose. We also found that irradiated mice hosted in the same cage had a comparable level (either high or low) of elastase. No significant differences were observed from the calprotectin data.
Conclusions: We found a clear association between the dose of ionizing radiation to the distal colon and the level of elastase in the fecal samples.
Introduction
During pelvic radiotherapy, unwanted irradiation to surrounding normal tissue triggers gastrointestinal symptoms that can develop into lifelong survivorship diseases [Citation1]. Worldwide, the consequences of radiotherapy on bowel function affect millions of cancer survivors [Citation2]. One major adverse effect is one that we have named ‘Urgency syndrome’ as a radiation-induced gastrointestinal survivorship disease, with symptoms including unexpected defecation without forewarning and not sensing the need to go to the toilet before it is too late. Experiencing defecation and the accompanying release of blood and mucus into clothing may be embarrassing and lead to social stigma [Citation1,Citation3,Citation4]. Tissue damage during irradiation induces inflammation, which is partly needed to defeat cancer cells. However, for some pelvic cancer patients, exaggerated inflammation can also promote the transition of the gut wall into dysfunctional fibrotic tissue. Hence, non-invasive inflammatory biomarkers to monitor radiation-induced gut-wall injury of the distal bowel are needed.
Elastase is secreted predominantly by neutrophils and macrophages during infection and inflammation [Citation5]. Traditionally, the level of fecal elastase is used as a marker for exocrine activity of the pancreas in patients with cystic fibrosis or chronic pancreatitis [Citation6] but has also been used as marker in patients with inflammatory bowel disease and colon cancer [Citation7]. However, elastase has not been used as marker to monitor radiation-induced bowel injury. In blood, a majority of the circulating white blood cells are neutrophils that serve as a first line of defense against bacterial and viral infection, but since elastase is a protease it also destroys host tissue through degradation of elastin and collagen. Inhibition of neutrophil elastase reduces radiation-induced lung injury in mice [Citation8] and protects against the development of lung fibrosis after irradiation through decreased collagen deposition [Citation9].
Calprotectin is a small calcium and zinc-binding protein belonging to the S100 family (S100 A8/A9) with a molecular weight of 36.5 kDa [Citation10]. The protein is abundant predominantly in neutrophils but also present in monocytes [Citation11]. As a marker of inflammation in the gastrointestinal tract, fecal calprotectin has been established as a useful clinical test [Citation12,Citation13]. Increased fecal calprotectin levels have been found in patients with gastric cancer, colorectal cancer, colonic polyps, and inflammatory bowel disease [Citation7]. Calprotectin has been used as a biomarker for acute intestinal toxicity in patients undergoing pelvic radiation therapy [Citation14] and acute radiation proctitis in prostate cancer treatment [Citation15].
Elastase and calprotectin are very stable enzymes and can be extracted from fecal samples and can even be detected from several-days-old stool samples without significant changes in levels [Citation11,Citation16]. Both elastase and calprotectin could possibly be useful as long-term non-invasive inflammatory markers. The aim of this study was to find out whether elastase and calprotectin could be used as markers for radiation-induced gut wall injury of the distal bowel in an experimental mouse model.
Material and methods
Mice and experimental set up
Eight to 9 week old adult male C57BL/6 mice (Charles River Breeding Laboratories, Germany) were used for all experiments. All animals were housed at a constant temperature (20 °C), with 42% relative humidity. A 12-h dark/light cycle was maintained with lights on from 07:00 am to 07:00 pm, and food and water were available ad libitum. All animal experimental procedures were approved by the Gothenburg Committee of the Swedish Animal Welfare Agency (application number 22-2015).
To investigate the effects of fractionated irradiation on the colorectal epithelium inflammation, a total of 12 groups of adult mice (a minimum of eight adult mice in each group) were used in this study. Three groups of animals received 6 Gray (Gy) irradiation; each group received either two (6 Gy × 2), three (6 Gy × 3), or four fractions (6 Gy × 4). Another three groups of animals received 8 Gy irradiation; each group received either two (8 Gy × 2), three (8 Gy × 3), or four fractions (8 Gy × 4). Six groups of animals served as controls to each of the 6 Gy and 8 Gy irradiation groups; these animals were anesthetized only (Sham-IRR).
Irradiation procedure
The irradiation methodology was developed by Bull et al and detailed information can be found in our previous report [Citation17]. In brief, adult male C57BL/6 mice were anesthetized with isoflurane (Sigma, Stockholm, Sweden) and exposed to irradiation with the goal of targeting sigmoid and rectum of the large bowel, using a linear accelerator (Varian Clinac 600 CD; Radiation Oncology Systems LLC, San Diego, CA). The radiation source was placed 100 cm away from the target, and the body was covered with 1 cm thick bolus material to obtain even irradiation throughout the underlying tissue. The radiation field was 3 × 3 cm2, the fractions were given with a dose-rate of 3.2 Gy/min, with 12 h between. Dose variation within the target volume was estimated to be ±5%. To verify correct dosimetry, we measured the delivered dose using an in vivo diode with a polystyrene phantom. The expected value for the diode was 5.98 Gy; the measured was 6.17 Gy. After irradiation, the mice were returned to their cages.
Fecal sample collection
Calprotectin and elastase are very stable proteins, but to assure the collected feces were no older than 24 h, cages were changed the day before collection. Each treatment group consisted of two cages housing four animals each. Thirty fecal pellets were collected from each cage at 1, 3, and 6 weeks post irradiation, and stored in −80 °C previous to analysis.
Measurement of fecal calprotectin level by ELISA
Calprotectin in fecal extracts was detected using the S100A8/S100A9 ELISA Kit (calprotectin enzyme-linked immunosorbent assay), according to the instructions from the manufacturer (Immundiagnostik AG, Bensheim, Germany). In brief, fecal samples from each cage were kept on ice and were subsequently divided into four piles and weighed, approximately 40 mg of fecal sample from each of the four piles was diluted in 1:25 in the supplied buffer, mixed for 30 s, and homogenized for 30 min at 1200 rpm (IKA Vibrax Shaker; Labortechnik, Wasserburg, Germany). Supernatant from the homogenate was centrifuged at 3000g for 10 min at 4 °C. The supernatants (100 µL) were added to the pre-coated and washed ELISA plates (96 well), together with standards (provided in the kit, the concentrations are 0.0, 0.25, 0.98, 3.9, and 15.6 ng/mL) and blank. The plates were incubated for 1 h at 37 °C on a horizontal shaker; the contents of each well were discarded. The plate was washed five times and 100 µL/well of lyophilized detection antibody were added and incubated for one hour at 37 °C. After washing, 100 µL/well of conjugate substrate was added and the plates were incubated for 1 h at 37 °C. The plate was washed five times and 100 µL/well of substrate were added and incubated for 5–15 min in dark. The reaction was stopped by stop solution, and detected at 405 nm on an Elisa-reader (Molecular Devices Emax, Sunnyvale, CA). The concentration of Calprotectin was expressed as ng/mL feces.
Measurement of fecal elastase level by ELISA
Elastase in fecal extracts was detected using the PMN-Elastase ELISA kit, mostly according to the manufacturer’s instructions (Immundiagnostik AG, Bensheim, Germany) but with some modifications. In brief, fecal samples from each cage were divided into four piles and weighed, 40 mg of fecal sample was diluted in 1:100 in supplied buffer, mixed for 30 s, and homogenized for 30 min at 1200 rpm (IKA Vibrax Shaker; Labortechnik, Wasserburg, Germany). Supernatant from the homogenate was centrifuged at 3000g for 10 min at 4 °C. The supernatants (100 µL), standards (ranging from 0.0, 0.04, 0.12, 0.37, 1.1–3.3 ng/mL) and blank, were added to the pre-coated and washed ELISA wells. The wells were incubated for one hour at 37 °C on a horizontal shaker the contents of each well were discarded, washed five times, and 100 µL/well of lyophilized detection antibody was added and incubated for 1 h at 37 °C. After washing, 100 µL/well of anti-mouse IgG donkey biotinylated (R&D Systems, Minneapolis, MN) were added and the plates were incubated for 1 h at 37 °C. The plate was washed five times and anti-mouse IgG donkey biotinylated (R&D Systems, Minneapolis, MN) was added and incubated for 30 min, and then washed five times. The reaction was stopped by Stop Solution and detected at 450 nm on an Elisa-reader (Molecular Devices Emax, Sunnyvale, CA). The concentration of elastase was expressed as ng/mL feces.
Statistical analysis
The different levels of elastase and calprotectin between irradiated mice and sham-irradiation were calculated using the non-parametric Kruskal–Wallis test. The different levels of elastase between cages were calculated by using Mann–Whitney test. Both tests were conducted by using the Prism 5 software (Prism GraphPad, San Diego, CA).
Results
The effects of fractionated irradiation on the inflammation of colorectal epithelium were investigated using adult mice to evaluate whether elastase or calprotectin could be used as fecal bio-markers of radiation-induced bowel injury. Irradiations were given at 6 Gy or 8 Gy in a total of 2, 3, or 4 fractions. Fecal calprotectin and elastase levels were detected by ELISA and the results were compared with sham-irradiated mice.
Calprotectin levels following 6 Gy irradiation
Fecal calprotectin levels at 1, 3, and 6 weeks after 6 Gy irradiation, given in 2, 3, and 4 fractions are presented in . No significant differences were observed in any of the groups between the irradiated and sham-irradiated mice.
Figure 1. (a–c) Calprotectin level in fecal samples at 1, 3, and 6 weeks after 6 Gy irradiation with 2, 3, or 4 fractions compared with sham-irradiated mice. (d and e), Elastase level in fecal samples at 1 and 6 weeks after 6 Gy irradiation with 2, 3, or 4 fractions compared with sham-irradiated mice. Error bars are shown as mean ± SEM, *p < .05 (Kruskal–Wallis test).
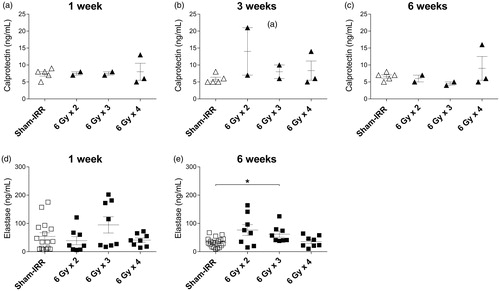
Elastase levels following 6 Gy irradiation
Fecal elastase levels at 1 week and 6 weeks following 6 Gy irradiation are shown in . Six weeks after 6 Gy × 3 fractions of irradiation, a significantly higher level of fecal elastase was observed when compared with sham-irradiated mice (p = .0479, Kruskal–Wallis test).
Calprotectin levels following 8 Gy irradiation
presents fecal calprotectin level following 8 Gy irradiation given in 2, 3, and 4 fractions (). There were no differences between the irradiated and sham-irradiated mice, consistent with the results acquired from fecal samples collected from animals receiving 6 Gy irradiation. However, the levels of calprotectin were slightly higher after 8 Gy irradiation compared to 6 Gy irradiation.
Figure 2. (a–c) Calprotectin level in fecal samples at 1, 3, and 6 weeks after 8 Gy irradiation with 2, 3, or 4 fractions compared to sham-irradiated mice. (d–f) Elastase level in fecal samples at 1, 3, and 6 weeks after 8 Gy irradiation with 2, 3, or 4 fractions compared to sham-irradiated mice. Error bars are shown as mean ± SEM, *p < .05; **p < .01; ***p < .001 (Kruskal–Wallis test).
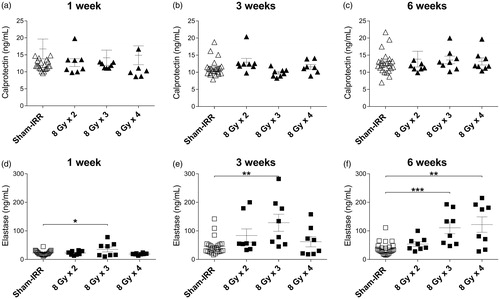
Elastase levels following 8 Gy irradiation
For fecal elastase, higher levels were observed at 8 Gy × 3 fractions (p = .0479, Kruskal–Wallis test) at 1 week after treatment when compared with sham-irradiated mice (). At 3 weeks after irradiation, higher levels of elastase were again detected in mice having received 8 Gy × 3 (p = .0038). At 6 weeks post-irradiation, both groups that had received 8 Gy × 3 fractions (p = .0009, Kruskal–Wallis test) and 8 Gy × 4 fractions (p = .0033, Kruskal–Wallis test) displayed significantly higher levels of fecal elastase than sham-irradiated animals. At 6 weeks after irradiation, a dose–effect correlation was observed between the abundance of elastase and the number of fractions.
Elevated elastase level in the irradiated mice was cage dependent
In our animal facility, four mice are hosted in each cage and each experiment used mice from two cages. Similar levels of elastase were observed in mice hosted in the same cage ( and ). On seven occasions, the differences between levels of elastase in mice in different cages within the same treatment group reached statistical significance (*p < .05, Mann–Whitney test).
Discussion
In the present study, we used a novel mouse model of radiation-induced colorectal injury that was established by us with the purpose to study underlying mechanism of radiation-induced bowel injury [Citation17]. Our model is especially suited for the long-term evaluation of radiation-induced gut injury, since animal survival is maintained despite the administration of high doses. The survival can be explained by the restricted target field, which is achieved by using linear accelerators, normally employed during human cancer radiotherapy. Using linear accelerators and a small target field, we do not have to shield the rest of the mouse to avoid causing immune system failure or lethal gastrointestinal toxicity. Both factors normally restrict other commonly used models to a follow-up time of a few days to a week [Citation18]. With our model, we were able to follow the levels of fecal elastase and calprotectin over time after fractionated radiation towards the colorectal of adult C57Bl/6 mice. We found a strong dose–effect relationship between dose of ionizing radiation and the fecal level of elastase, but not calprotectin. In addition, we found that irradiated mice hosted in the same cage had a comparable level of elastase, while cage sharing had no effect on the fecal levels of calprotectin.
We observed increasing levels of elastase with increasing fractions and irradiation dose. The abundancy of fecal elastase likely corresponds to the degree of radiation injury to the gut wall. We previously observed increasing number of macrophages 6 weeks after 8 Gy irradiation which also reflected that the inflammatory activity were still remained at this time point [Citation17]. However, chronic secretion of elastase could exacerbate the development fibrosis in the gut. One common treatment-related side effect that pelvic cancer survivor suffer from is radiation-induced intestinal fibrosis. Studies on radiation-induced lung disease showed that inhibition of neutrophil elastase reduced lung fibrosis and prolonged survival of the irradiated mice [Citation19]. Moreover, multiple administration of low-dose elastase could reduce elastic fiber content and increase collagen fiber in mouse lung tissue [Citation20]. Elastase has the ability to disrupt tight junctions of the lung epithelial lining and increase epithelial permeability, cause proteolytic damage to tissue, and break down cytokines [Citation21,Citation22]. These processes prompt a further release of cytokines and recruitment of pro-inflammatory cells, and they induce a subsequent vicious circle of inflammatory responses. In the gut, the tight junctions connecting the epithelial cells are an indispensable component of the gut barrier, preventing the translocation of luminal contents into the body. The increased levels of elastase in our model could thus be an important underlying mechanism of the inflammation and fibrosis that develops as a response to radiation.
Interestingly, we also found that the abundancy of fecal elastase is strongly influenced by the surrounding environment, since irradiated mice hosted in the same cage had comparable levels (either high or low) of fecal elastase. This co-variation could be due to the fact that mice who share the same cage harbor a distinct gut microbiota that could facilitate inflammation or has anti-inflammatory effects [Citation23]. We could not rule out that the differences could also be due to potential infections of mice hosted in the same cage. However, our mice appeared to be healthy and gained weight throughout the experiment [Citation17]. We showed in our previous publication that no significant induction of apoptosis was found in the proximal colon [Citation17]. However, we could not completely exclude that the intestinal inflammation in our model only involves the sigmoid colon and rectum. Microbiota before and during irradiation is important for gut health post-irradiation. Patients with a healthy gut flora before radiation therapy have lower post-irradiation side-effects [Citation24]. Interestingly, an exogenously administered microflora stimulates proliferation in the gut epithelial lining as seen in germ-free rats [Citation25]. In addition, the protective mucus barriers of the epithelium are shaped by the microbiota, and the mucus layer could either be impenetrable or be penetrable depending on the distinct microbiota presented in the gut [Citation26]. It would be interesting to compare the gut microbiota of mice from cages with high elastase level with low elastase level cages. However, our fecal collection initially was not aimed for bacterial species-analysis and many gut bacteria are obligate anaerobes. Hence, we expected that many bacterial species would have died during fecal collection and a microbiota analysis would not have resulted in a representative picture. For future experiments, it is of importance to collect fecal samples directly from each mouse and store under proper conditions for microbiota analysis.
Traditionally, calprotectin has been used as a marker for intestinal inflammation in patients with inflammatory bowel disease and acute intestinal toxicity in patients undergoing pelvic radiotherapy [Citation27]. However, all available data from our study pointed out that calprotectin was not a suitable inflammation marker after irradiation of the rectal region. This was confirmed by a previous study, where Varela et al. showed that the fecal calprotectin levels doubled during pelvic radiotherapy of the non-rectal group, whereas no significant changes were found in patients with irradiation of the rectal region [Citation14].
A strength of the present study is that the fractionated irradiation was given to a small concentrated field with a known position i.e., sigmoid and rectum of the large bowel with linear accelerators. This experimental design more closely mimics the radiotherapy given to prostate or gynecological cancer patients than other commonly used animal models of radiation-induced gut injury [Citation18]. One weakness of the study is that the experiment was carried out on animal models. Our model does not reflect all disease aspects of radiation enteritis in humans [Citation28]. However, we do believe that elastase is a more sensitive marker than calprotectin regarding radiation-induced damage to the mucosa in the colorectum, and that it is worthwhile to follow up our finding in human studies.
Conclusion
We need non-invasive and reliable markers to understand why some patients develop radiation-induced gastrointestinal survivorship diseases. To the best of our knowledge, elastase has not been used as marker for radiation-induced intestinal inflammation in patients undergoing pelvic radiotherapy. Future studies will evaluate if this still holds true when re-directing our research from animal model to cancer patients.
Acknowledgments
The authors would like to thank Dr John Swanpalmer for dosimetric calculations, BSc Rita Grandér for her technical expertise concerning animal radiation models, the staffs at Jubileumskliniken, Sahlgrenska University Hospital, MSc Arne Ståhl for assistance with the ELISA analysis and Larry Lundgren for proofreading.
Disclosure statement
The authors report no conflicts of interest.
Additional information
Funding
References
- Steineck G, Skokic V, Sjoberg F, et al. Identifying radiation-induced survivorship syndromes affecting bowel health in a cohort of gynecological cancer survivors. PLoS One. 2017;12:e0171461.
- Andreyev HJ, Wotherspoon A, Denham JW, et al. “Pelvic radiation disease”: new understanding and new solutions for a new disease in the era of cancer survivorship”. Scand J Gastroenterol. 2011;46:389–397.
- Oh JH, Thor M, Olsson C, et al. A factor analysis approach for clustering patient reported outcomes. Methods Inf Med. 2016;55:431–439.
- Steineck G, Schmidt H, Alevronta E, et al. Toward restored bowel health in rectal cancer survivors. Semin Radiat Oncol. 2016;26:236–250.
- Fournier BM, Parkos CA. The role of neutrophils during intestinal inflammation. Mucosal Immunol. 2012;5:354–366.
- Leus J, Van Biervliet S, Robberecht E. Detection and follow up of exocrine pancreatic insufficiency in cystic fibrosis: a review. Eur J Pediatr. 2000;159:563–568.
- Saitoh O, Sugi K, Matsuse R, et al. The forms and the levels of fecal PMN-elastase in patients with colorectal diseases. Am J Gastroenterol. 1995;90:388–393.
- Yoshikawa N, Inomata T, Okada Y, et al. Sivelestat sodium hydrate reduces radiation-induced lung injury in mice by inhibiting neutrophil elastase. Mol Med Rep. 2013;7:1091–1095.
- Fujino N, Kubo H, Suzuki T, et al. Administration of a specific inhibitor of neutrophil elastase attenuates pulmonary fibrosis after acute lung injury in mice. Exp Lung Res. 2012;38:28–36.
- Alibrahim B, Aljasser MI, Salh B. Fecal calprotectin use in inflammatory bowel disease and beyond: a mini-review. Can J Gastroenterol Hepatol. 2015;29:157–163.
- Johne B, Fagerhol MK, Lyberg T, et al. Functional and clinical aspects of the myelomonocyte protein calprotectin. Mol Pathol. 1997;50:113–123.
- Caviglia GP, Pantaleoni S, Touscoz GA, et al. Fecal calprotectin is an effective diagnostic tool that differentiates inflammatory from functional intestinal disorders. Scand J Gastroenterol. 2014;49:1419–1424.
- Kopylov U, Rosenfeld G, Bressler B, et al. Clinical utility of fecal biomarkers for the diagnosis and management of inflammatory bowel disease. Inflamm Bowel Dis. 2014;20:742–756.
- Varela E, Antolin M, Guarner F, et al. Faecal DNA and calprotectin as biomarkers of acute intestinal toxicity in patients undergoing pelvic radiotherapy. Aliment Pharmacol Ther. 2009;30:175–185.
- Hille A, Schmidt-Giese E, Hermann RM, et al. A prospective study of faecal calprotectin and lactoferrin in the monitoring of acute radiation proctitis in prostate cancer treatment. Scand J Gastroenterol. 2008;43:52–58.
- Uchida K, Matsuse R, Tomita S, et al. Immunochemical detection of human lactoferrin in feces as a new marker for inflammatory gastrointestinal disorders and colon cancer. Clin Biochem. 1994;27:259–264.
- Bull C, Malipatlolla D, Kalm M, et al. A novel mouse model of radiation-induced cancer survivorship diseases of the gut. Am J Physiol Gastrointest Liver Physiol. 2017;313:G456–GG66.
- Paris F, Fuks Z, Kang A, et al. Endothelial apoptosis as the primary lesion initiating intestinal radiation damage in mice. Science. 2001;293:293–297.
- Fox J, Haston CK. CXC receptor 1 and 2 and neutrophil elastase inhibitors alter radiation-induced lung disease in the mouse. Int J Radiat Oncol Biol Phys. 2013;85:215–222.
- Oliveira MV, Abreu SC, Padilha GA, et al. Characterization of a mouse model of emphysema induced by multiple instillations of low-dose elastase. Front Physiol. 2016;7:457.
- Azghani AO, Connelly JC, Peterson BT, et al. Effects of Pseudomonas aeruginosa elastase on alveolar epithelial permeability in guinea pigs. Infect Immun. 1990;58:433–438.
- Azghani AO. Pseudomonas aeruginosa and epithelial permeability: role of virulence factors elastase and exotoxin A. Am J Respir Cell Mol Biol. 1996;15:132–140.
- Kostic AD, Xavier RJ, Gevers D. The microbiome in inflammatory bowel disease: current status and the future ahead. Gastroenterology. 2014;146:1489–1499.
- Manichanh C, Varela E, Martinez C, et al. The gut microbiota predispose to the pathophysiology of acute postradiotherapy diarrhea. Am J Gastroenterol. 2008;103:1754–1761.
- Carter PB, Pollard M. Host responses to “normal” microbial flora in germ-free mice. J Reticuloendothel Soc. 1971;9:580–587.
- Jakobsson HE, Rodriguez-Pineiro AM, Schutte A, et al. The composition of the gut microbiota shapes the colon mucus barrier. EMBO Rep. 2015;16:164–177.
- Larsen A, Hovdenak N, Karlsdottir A, et al. Faecal calprotectin and lactoferrin as markers of acute radiation proctitis: a pilot study of eight stool markers. Scand J Gastroenterol. 2004;39:1113–1118.
- Andreyev HJ, Vlavianos P, Blake P, et al. Gastrointestinal symptoms after pelvic radiotherapy: role for the gastroenterologist? Int J Radiat Oncol Biol Phys. 2005;62:1464–1471.