Abstract
Introduction: Previous studies revealed that dose escalated radiotherapy for prostate cancer patients leads to higher tumor control probabilities (TCP) but also to higher rectal toxicities. An isotoxic model was developed to maximize the given dose while controlling the toxicity level. This was applied to analyze the effect of an implantable rectum spacer (IRS) and extended with a genetic test of normal tissue radio-sensitivity. A virtual IRS (V-IRS) was tested using this method. We hypothesized that the patients with increased risk of toxicity would benefit more from an IRS.
Material and methods: Sixteen localized prostate cancer patients implanted with an IRS were included in the study. Treatment planning was performed on computed tomography (CT) images before and after the placement of the IRS and with a V-IRS. The normal tissue complication probability (NTCP) was calculated using a QUANTEC reviewed model for Grade > =2 late rectal bleeding and the number of fractions of the plans were adjusted until the NTCP value was under 5%. The resulting treatment plans were used to calculate the TCP before and after placement of an IRS. This was extended by adding the effect of two published genetic single nucleotide polymorphisms (SNP’s) for late rectal bleeding.
Results: The median TCP resulting from the optimized plans in patients before the IRS was 75.1% [32.6–90.5%]. With IRS, the median TCP is significantly higher: 98.9% [80.8–99.9%] (p < .01). The difference in TCP between the V-IRS and the real IRS was 1.8% [0.0–18.0%]. Placing an IRS in the patients with SNP’s improved the TCP from 49.0% [16.1–80.8%] and 48.9% [16.0–72.8%] to 96.3% [67.0–99.5%] and 90.1% [49.0–99.5%] (p < .01) respectively for either SNP.
Conclusion: This study was a proof-of-concept for an isotoxic model with genetic biomarkers with a V-IRS as a multifactorial decision support system for the decision of a placement of an IRS.
Introduction
Intensity-modulated radiotherapy (IMRT) is one of the primary curative treatments for patients with prostate cancer. Studies have revealed that dose escalation leads to better biochemical progression free survival [Citation1,Citation2]. However, the proximity between the anterior rectal wall and the prostate allows for limited dose escalation without resulting in severe gastro-intestinal (GI) toxicity [Citation3–5].
The implantable rectum spacer (IRS) is a device developed to spare the rectum by increasing the distance between the anterior rectal wall and the prostate, thus reducing the overlap between the rectal wall and the high-dose region [Citation6–9]. Recent studies have shown that this device significantly reduces the dose in the rectum, thus reducing the risk of complications, for approximately 20% of the patients [Citation5]. For these patients, the IRS would allow for same radiation dose and cure rate with decreased rectal toxicity rates. There is increasing evidence supporting the role of several genetic variants in the development of radiation-induced toxicity to distinguish the patients having increased risk factors for severe rectal and urinary toxicity [Citation10,Citation11]. We hypothesize that the patients with increased risk of radiation-induced rectal toxicity would benefit more from an IRS. Furthermore, the use of IRS in prostate cancer patients allows for more dose escalation, with consequently improving their prospective therapeutic ratio.
In this proof-of-concept study, we developed a method to determine the amount of possible dose escalation with the resulting tumor control probability (TCP) without exceeding the set of boundaries for the complication risks: the so-called ‘isotoxic dose escalation’ approach [Citation12–14]. This method is applied to compare the possible dose escalation on patients with plans available before and after the placement of an IRS. This model is combined with a so-called ‘virtual IRS’ (V-IRS) [Citation15]. This method uses image deformation to obtain CT images of patients with a predicted IRS position without having to place one (watch the animation: https://www.youtube.com/watch?v=tDlagSXMKqw). Furthermore, this method helps identify the patients for whom significantly higher TCP is possible by placing an IRS.
To further individualize the predictions done for patients, the patients radio-sensitivity needs to be taken into account. Studies have shown that the difference in radio-sensitivity between patients are likely due to common genetic variants, such as single nucleotide polymorphisms (SNPs). We applied the developed method to compare the results for patients using the model including and not including the genetic biomarker.
Material and methods
Patient characteristics
For this study, 16 patients with localized prostate cancer who had signed an informed consent were included (see table in Supplementary Appendix I). Two different commercial types of IRS’s are used: 8 had received a rectum balloon implant (RBI) (BioProtect Ltd, Israel) [Citation6] and eight had a hydrogel spacer (HGS) (SpaceOARTM System, Augmenix Inc., Waltham, MA) placed. The hydrogel and RBI patients included in this study were consecutively selected in 2011 and 2015, respectively. Both studies were approved by their local ethics committees [Citation7]. The RBI patients were included in a clinical trial between 2015 and 2016 (registration number: 14-38-03/09-internal-6335) [Citation9]. As this is a consecutive case study, it is assumed these patients are representative of average prostate cancer patients.
Imaging and treatment planning
Standard computed tomography (CT) imaging was performed twice on all patients while they were in supine position: once before and once after placement of an IRS. These images had a slice thickness of 5 mm for the patients with a HGS, and 3 mm for those with a RBI. The pixel size was 1 mm for all images
The clinical target volume (CTV) was defined as the prostate for eight patients, and the prostate including seminal vesicles for the rest and the planning target volume (PTV) was obtained by extending the CTV with 6 mm in all directions to account for uncertainties in planning a treatment delivery. The rectum volumes were delineated as a solid organ, including the rectum as well as the anal canal. The same experienced radiation oncologist (BV) did all delineations.
Plans were performed by a certified technician on all CT images, yielding two treatment plans for each patient (one without IRS, one with IRS). The treatment plans were designed for dose delivery with a knowledge-based volumetric modulated arc technique with 10 MV photon beams (Eclipse Version ICD-10, Varian Medical Systems Inc., Palo Alto). Dose delivery was planned to be done in 28 fractions of 2.5 Gray (Gy) [Citation16]. Dose constraints held during treatment planning are shown in Supplementary Appendix II.
Isotoxic model
Dose calculations
Treatment planning was performed in fractions of 2.5 Gy instead of 2 Gy, which is the case for the treatment plans on which the NTCP model was based. For this reason, the equivalent 2 Gy dose (EQD2) in the rectum resulting from the treatment plan is calculated and used as input for the model. This is done using the Withers Equation [Citation17] (1):
(1)
Here is the total dose,
is the fraction dose and
represent the fractionation sensitivity of the organ. For late rectal toxicity, the
ratio has been estimated to be 3 Gy [Citation18].
NTCP model
The NTCP model used is the Lyman–Kutcher–Burman (LKB) model [Citation19] that was reviewed by the QUANTEC group (quantitative analysis of normal tissue effects in the clinic). The LKB model is a dose response curve that uses the generalized equivalent uniform dose () as a dose parameter, which is obtained using the DVH, and a parameter
, which determines which part of the DVH is statistically most important. The curve is dependent on the dose required for an NTCP of 50% (
) and the steepness of the curve (
). The QUANTEC-recommended parameters are
76.9,
= 0.09 and
= 0.13 for grade> =2 late rectal toxicity. For more details see Supplementary Appendix III.
Single nucleotide polymorphisms
This NTCP model was expanded as a proof-of-concept with genetic markers of rectal toxicity, for which we used published results of a meta-analysis of GWASs (Genome Wide Association Study) for genetic markers of late toxicity, in prostate cancer patients treated with radiotherapy [Citation11]. The two SNPs most associated with rectal bleeding, that approach meta-analysis significance, were selected for the initial proof-of-concept: rs141044160 (SNP 1) with an odds ratio of 2.68 (P = 2.26 × 10−6) and rs7432328 (SNP 2), with an odds ratio of 3.36 (P = 3.32 × 10−6). The SNP’s were incorporated into the dose response curve using a published mathematical method [Citation20]. In this method, clinical parameters are incorporated into a dose response by separating the curve for the overall population into two curves: one for the population with the SNP risk allele and one for the population without. This is done using the odds ratio and the proportion between the population with and without the risk factor. The proportion of the population with the SNP risk allele can be calculated using the minor allele frequency (MAF). For this study, we demonstrated the effects of the SNPs on the NTCP model by applying it on the patient cohort, assuming they were carriers for either SNP risk allele were considered (Supplementary Appendix IV).
TCP model
The used TCP model [Citation21] was built to predict the clinical response to external beam radiotherapy in patients with low-, intermediate- and high-risk prostate cancer and is available on http://predictcancer.org/Main.php?page=TCPprostate-Model. This model utilizes realistic radio biologically input parameters and works for a wide range of treatment strategies [Citation22].
The inputs to the TCP model are the number of fractions, the fraction dose, the treatment modality and the risk group of the patient. The prostate-specific antigen (PSA), TNM and Gleason score are the input factors to the classification of the risk group: low, medium or high [Citation23]. To evaluate the result of dose escalation, only the fraction dose is varied in this study to test the amount of dose escalation possible without exceeding NTCP limits.
Isotoxic method
The proposed model uses an optimized treatment plan for a patient, calculates the DVH in the organs at risk and scales this DVH by changing the fraction dose used during treatment planning. The fraction dose is varied in order to obtain a maximum TCP value without exceeding the cutoff value set for the NTCP value, which, for this paper, is arbitrarily chosen to be 5% (). The fraction dose for a plan with 28 fractions advised by the model can vary between 1.5 and 3.5 Gy. These limits are chosen because a plan with 28 fractions with less than 1.5 Gy, resulting TCP was modeled to always be lower than 25%, and more than 3.5 fractions was to always result in a TCP higher than 95%. In reality 1.5 Gy in 28 fractions would not be for curative treatment, but in this study, these plans are calculated for theoretical comparison.
Figure 1. A flowchart of the isotoxic model. The model loads a treatment plan made by an expert and increases or decreases the fraction dose. Each time fraction dose is increased or decreased, the normal tissue complication probability (NTCP) is recalculated until a set cutoff value is reached. The tumor control probability (TCP) is hen calculated.
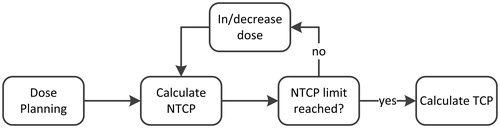
The method only takes into account rectal toxicity, but as the bladder is also an organ at risk, we added a constraint to the bladder dose [Citation24], as is shown in Supplementary Appendix V.
To use the method to select patients for whom the placement of an IRS is beneficial, a minimum increase in TCP should be chosen. For this study, two cutoff values were used for the increase in TCP as a result of the placement of an IRS: 25% and 50%.
Virtual spacer
In order to use the isotoxic model to predict the improvement of the IRS in a specified patient, CT images of a patient are needed before the placement of the IRS. To this end, a previously developed V-IRS was used [Citation15]. This V-IRS uses a model IRS derived from 7 delineations of a RBI and uses image deformation to insert the model into a CT image of a patient without an IRS, thus creating a virtual image of the patient with an IRS. This virtual image is then subjected to treatment planning, and the isotoxic model is used to calculate the highest possible TCP without exceeding the NTCP limit. In this study we test the V-IRS by comparing the results of the isotoxic model to those of the actual IRS.
Statistics
We used two-tailed Wilcoxon signed rank tests to determine whether the increase in optimal TCP for the plans without IRS and with IRS was significant. The test was also applied to test if the difference between the NTCP values before and after placement was significant. p-values of less than 0.05 were considered significant.
Results
Dose escalation
Giving more dose per fraction increases both the TCP and the NTCP predictions for a given patient (). The original plans (before applying the isotoxic method) consisted of 28 fractions of 2.5 Gy, which, for this set of patients corresponds to a median TCP of 80.8% with a range of [51.8–92.0%]. The median fraction dose allowed by the model without exceeding the NTCP constraint before the placement of an IRS was 2.5 Gy [2.4–2.7 Gy] (), and corresponds to 75.1% [32.6–90.5%] TCP. The TCP for the patients when given a conformal treatment plan of 2 Gy in 35 fractions corresponds to a TCP of 60.8% [26.3–80.9%]. shows that to obtain a median TCP of at least 90%, the dose needs to be escalated further to 2.7 Gy per fraction for a treatment plan with 28 fractions, which was possible for 2 of the 16 patients while holding to the pre-set accepted toxicity rates. For 5 of the patients, 2.6 Gy per fraction could be given, and for the remaining patients, no dose escalation was possible.
Figure 2. The median tumor control probability (TCP) and the median normal tissue complication probability (NTCP) is plotted against the fraction dose for the patients without implantable rectum spacer (IRS) and with IRS.
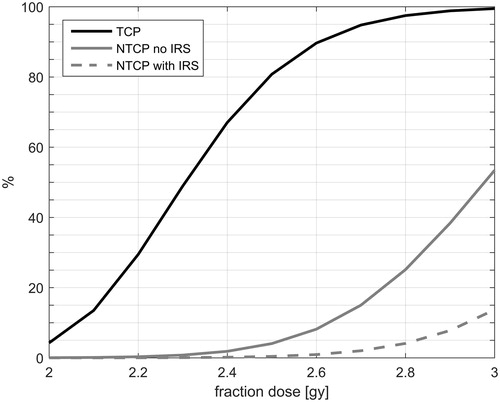
Table 1. An overview of the fraction dose, the NTCP grade> =2 late rectal bleeding and the TCP results.
IRS gain
The placement of an IRS resulted in an increase in advised fraction dose of 0.4 Gy [−0.1–0.9 Gy] on average for all patients. Note that the minimum increase is negative, which means this is a decrease. This results in an absolute increase in TCP of 21.1% [−8.9–57.9%] which is statistically significant (p < .01) (). Patient-specific outcomes have been plotted in a similar manner in Supplementary Appendix VI. In only one patient, the TCP after the placement of an IRS is lower than before placement. For this patient, the rectum volume had decreased after the placement of an IRS (from 268.9 cm3 to 156.1 cm3).
Figure 3. The median tumor control probability (TCP) and range, as well as the median normal tissue complication probability (NTCP) and range, for the patients without implantable rectum spacer (IRS), with IRS and with the virtual IRS (V-IRS) is shown.
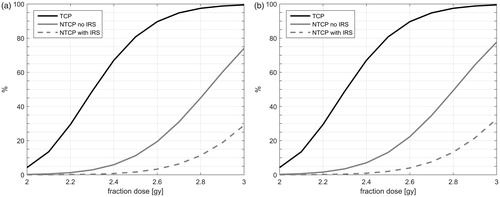
If an increase in TCP of 25% is taken as a threshold for the decision to place an IRS, then 7 of the 16 patients would be selected by the model for the placement of an IRS. Two patients even had an increased TCP of 50% resulting from the IRS.
The difference in the NTCP resulting from the optimized plan for the patients without IRS and with IRS is 0.15% and is not significant (p = .47). We performed an analyses for different NTCP limits in Supplementary Appendix VII.
Performance V-IRS
The difference in fractionation result between the V-IRS and the real IRS was a median of 0.3 Gy [0–0.8 Gy], which translates to a median TCP difference of 1.8% [0.0–18.0%]. The difference between the optimized TCP for the real IRS and the V-IRS was not significant (p = .2). There were two patients for whom the difference in TCP between the V-IRS plan and the IRS plan was 18%, and in both patients the rectum volume had changed significantly (57.9% and 58.1% volume decrease in the image after the IRS).
When considering a minimum increase in TCP of 25% for the placement of an IRS, then 6 of the 16 patients would be selected by the model using the V-IRS, in contrary to the 7 selected using the actual IRS. The increase predicted by the V-IRS was underestimated compared to the real IRS in one patient, with an increase of 13.7% versus 27.7%. The two patients who had an increased TCP of 50% resulting from the real IRS, had the same increase predicted by the V-IRS.
SNP results
shows the TCP and the NTCP as function of the fraction dose, and it can be observed that though the TCP is the same as for the patients without SNPs, the NTCP curve is much steeper for both SNPs.
Figure 4. The median tumor control probability (TCP) and the median normal tissue complication probability (NTCP) is plotted against the fraction dose for the patients with SNP’s (a: rs141044160 and b: rs7432328) without implantable rectum spacer (IRS) and with IRS.
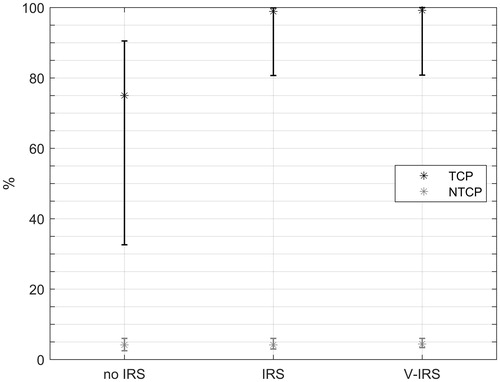
The median NTCP for the original plan (28 fractions of 2.5 Gy) was 11.2% [2.2–19.6%] and 13.1% [2.7–22.5%] for SNP 1 and SNP 2, respectively, which is reduced significantly (P < .01) when using the isotoxic method. The results show () that if the patients are carriers of the chosen SNPs, the TCP while restraining the NTCP would be 49.0% for SNP 1, and 48.9% for SNP 2. The placement of an IRS resulted in significantly better TCP values (p < .01), with a median of 96.3% [67.0–99.5%] and 90.1% [49.0–99.5%] for SNP 1 and SNP 2, respectively, which was significantly higher than without IRS (p < .01) for both SNP’s. Four other SNP’s were found to be near significant for late rectal bleeding; results for these SNP’s are shown in Supplementary Appendix VIII. We performed a sensitivity analyses on a range of odds ratios to show the possible effects of different SNP’s for late rectal bleeding in Supplementary Appendix IX.
Table 2. An overview of the hypothetical fraction dose, the NTCP grade ≥2 late rectal bleeding and the TCP results if the patients were carrier of the risk alleles for either of the selected SNP’s.
Discussion
In this study, we have developed an isotoxic method integrating genetic markers of rectal radio-sensitivity combined with a V-IRS which can be used to support the decision for the implantation of an IRS for a specified patient or not. The method applies image deformation to a CT image of a patient without IRS and creates a virtual CT image with IRS. The method calculates the maximum dose per fraction that can be given without exceeding an upfront determined limit for the NTCP value for late rectal bleeding.
The results show that a higher prescribed dose should be given to improve TCP, and can be safely administered when combined with an IRS. This method can be used as the basis of a multifactorial DSS [Citation25], which can be used as a meta-treatment planning system [Citation26] to decide in which patients to place an IRS, as well as advise on how much dose escalation is possible to apply for a specified patient [Citation27,Citation28]. This method also allows for shared decision making, taking into account the patients priorities, such as cure rate or risk of toxicity.
Our method has two main strengths. Firstly, the method uses NTCP prediction values to predict the amount of dose escalation possible while keeping rectal toxicities under control. Applying this method in the clinic would allow dose escalation to be used more safely and improve outcomes for patients [Citation29]. Secondly, treatment planning with an IRS can be applied to a CT of a patient without the real implantation of the IRS itself. This means that the increase in TCP with the IRS for the specified patient can be estimated without having to put the patient through the discomfort of the implantation, and without the additional costs of the placement of the IRS, before it has been established that an IRS will be beneficial.
The results showed that the system would advise further dose escalation even without the placement of an IRS, which would result in better outcomes for these patients. The system foresees a gain in TCP for 7 of the 16 patients, showing the model can be used as to identify patients for whom an IRS is beneficial. Using the V-IRS, 6 of the 7 patients for whom the spacer would be significantly effective could be identified. The patient not identified had very different rectal fillings before and after IRS placement. This demonstrates that this DSS has the potential to identify patients who benefit most from an IRS. This improves the cost-effectiveness of the IRS by only placing it in patients who would benefit from it, while also improving their survival and quality of life.
During this study, SNP’s were incorporated into the NTCP model and tested on the patient cohort, assuming all were heterogeneous for either SNP. Because the toxicity risk of a carrier is larger than for a non-carrier, these patients could normally be considered for alternative treatments, such as surgery. However, the method presented here has the potential to identify patients with this SNP who could be treated safely with radiotherapy after placement of an IRS. This would be beneficial, since placing an IRS is less invasive and has a very low to no impact on the quality of life, especially in comparison with surgery [Citation8]. This would also be beneficial for patients who don’t want an operation or who are not candidates for surgery.
One of the main limitations of the study was the small amount of patients included. Though they were sufficient for a first proof-of-concept, a more elaborate study including a larger amount of patients is required for internal validation of the method, and additional data sets are required for external validation.
Furthermore, the LKB model uses the entire DVH as a predictor for late rectal bleeding, which is partly effected by the rectal volume, a factor which varies strongly over time [Citation30]. The model cannot predict these changes in rectal volume, and for some patients this may influence the outcome. For two patients, this difference in volume was as much as 150 cm3, and for one patient, this resulted in a large overestimation of the NTCP by the V-IRS. For another patient, the decrease in rectal volume even resulted in an increased NTCP after the placement of the IRS. A possible solution to this problem is to submit patients with very full rectums to rescanning, or for patients to be given a laxative prior to scanning. For future validation of the V-IRS, only CT scans with similar rectal filling before and after IRS placement should be used, as only then a fair comparison can be made. Finally, concerning optimizing the treatment planning technique: it is possible to reduce intermediate dose levels (30−50 Gy) in the rectum region by an arc-therapy with an avoidance-region near the rectum.
Another possible error in both the TCP and the NTCP model is the α/β ratio, which varies between prostate, rectum and bladder. In order to fully understand the effect possible errors in the α/β ratio have on the calculated outcome, a sensitivity analyses should be done. However, considering the uncertainty in estimates for α/β ratio’s results for these kind of analysis should always be interpreted carefully.
The V-IRS used in this study was based on a RBI, a closed system which has a predictable shape, while 8 of the patients were implanted with a HGS, which has a highly variable shape [Citation31]. However, patients with a large increase in TCP resulting from a hydrogel spacer can still be identified using the V-IRS, and therefore, it still has potential as the basis of the DSS, even for the hydrogel spacer. The V-IRS has been published in a proof-of-concept study but requires validation. The V-IRS performance is promising; however, after validation, it is expected to improve and consequently leading to better outcome results.
The integration of the SNPs is theoretical in this study, as none of the patients were sequenced and tested for the SNPs, and as the MAF is low (0.05 and 0.06), it is not likely any of them would be carriers. An improved method would be to build an NTCP model on a large patient cohort for whom sequencing has been done and for whom outcome data has been collected. Also, though SNPs have been identified that are significant for rectal toxicity in general, currently no SNPs have been found to be significant for late rectal bleeding specifically [Citation11]. To date, six SNPs have been associated with rectal bleeding (11), of which two were used in this study. These SNPs approaching statistical significance in a meta-analysis (p < 5 × 10−6). Further validation in larger studies are needed before incorporate these SNPs in NTCP models. Once significant SNPs are identified, these can be incorporated into NTCP models.
The NTCP model is dependent on the DVH in the rectum; however, during treatment this, DVH might vary depending on day-to-day variability of rectum filling. This could influence the accuracy of the NTCP model. In order to fully demonstrate the effect this has on the NTCP prediction, the real administered rectal dose should be computed. Also, the NTCP model used in this study only uses the DVH as a predictor for late rectal bleeding, however, studies have shown that several clinical parameters (diabetes, abdominal surgery, etc.) are also strong predictors. In future work, we intend to include NTCP models using clinical predictors into the model as well as validated SNPs. We also want to validate the method of combining published SNP’s with NTCP models on a dataset for which sequencing was done, and for which outcomes were known.
Including different endpoints for the toxicity models, such as incontinence, erectile dysfunction and urinary toxicity would serve to further improve patient specific decision making. Another good expansion of the model would be to add a cost-effectiveness analyses, which would enhance the cost-effectiveness of the IRS, a topic that is becoming increasingly important due to ever-expanding expenses in health care [Citation32,Citation33]. Another development would be to focus on hypofractionation as well. Possibilities for extreme hypofractionation, such as stereotactic radiotherapy, single fraction high-dose rate brachytherapy or single-shot proton therapy could be explored. This would serve to further increase patient prospects as well as cost-effectiveness.
Before application in the clinic is possible, the DSS model needs to be further developed while taking into accounts the limits of treatment planning and application. When the model advices lower dose prescription than the original treatment plan, the V-MAT system might not be able to calculate the treatment plan. Analyses should be done on how easily prescription dose can be adjusted, and whether variation in fraction dose and/or number of fractions would be most effective.
The isotoxic model integrating genetic markers for rectal toxicity developed in this study can be used to evaluate a treatment plan, and test how much dose can be given without causing excessive damage to the rectum. Thus sparing the organs at risk at a chosen described level while optimizing the TCP. In combination with the V-IRS, this method can serve as the basis for a DSS for the implantation of an IRS.
Supplemental Material
Download MS Word (918.7 KB)Disclosure statement
Sean Walsh is a salaried employee of ptTheragnostic B.V. and CSO of OncoRadiomics SPRL. Philippe Lambin is co-inventors of IP related to Decision Support Systems and he is member of the advisory board of ptTheragnostic/DNAmito. Cary Oberije is employee and CEO of ptTheragnostic B.V.
Additional information
Funding
References
- Kalbasi A, Li J, Berman A, et al. Dose-escalated irradiation and overall survival in men with nonmetastatic prostate cancer. JAMA Oncol. 2015;1:897–906.
- Zelefsky MJ, Pei X, Chou JF, et al. Dose escalation for prostate cancer radiotherapy: predictors of long-term biochemical tumor control and distant metastases-free survival outcomes. Eur Urol. 2011;60:1133–1139.
- Glass AS, Cowan JE, Fuldeore MJ, et al. Patient demographics, quality of life, and disease features of men with newly diagnosed prostate cancer: trends in the PSA era. Urology. 2013;82:60–65.
- Vanneste BG, Van De Voorde L, de Ridder RJ, et al. Chronic radiation proctitis: tricks to prevent and treat. Int J Colorectal Dis. 2015;30:1293–1303.
- Vanneste BG, Hoffmann AL, van Lin EN, et al. Who will benefit most from hydrogel rectum spacer implantation in prostate cancer radiotherapy? A model-based approach for patient selection. Radiother Oncol. 2016;121:118–123.
- Gez E, Cytron S, Ben Yosef R, et al. Application of an interstitial and biodegradable balloon system for prostate-rectum separation during prostate cancer radiotherapy: a prospective multi-center study. Radiat Oncol. 2013;8:96.
- Pinkawa M, Corral NE, Caffaro M, et al. Application of a spacer gel to optimize three-dimensional conformal and intensity modulated radiotherapy for prostate cancer. Radiother Oncol. 2011;100:436–441.
- Vanneste BG, van De Beek K, Lutgens L, et al. Implantation of a biodegradable rectum balloon implant: tips, tricks and pitfalls. Int Braz J Urol. 2017;43:1033.
- Vanneste BGL, van Wijk Y, Lutgens LC, et al. Dynamics of rectal balloon implant shrinkage in prostate VMAT: Influence on anorectal dose and late rectal complication risk. Strahlenther Onkol. 2018;194:31–40.
- Fachal L, Gomez-Caamano A, Barnett GC, et al. A three-stage genome-wide association study identifies a susceptibility locus for late radiotherapy toxicity at 2q24.1. Nat Genet. 2014;46:891–894.
- Kerns SL, Dorling L, Fachal L, et al. Meta-analysis of genome wide association studies identifies genetic markers of late toxicity following radiotherapy for prostate cancer. EBioMedicine. 2016;10:150–163.
- Zindler JD, Thomas CR Jr., Hahn SM, et al. Increasing the therapeutic ratio of stereotactic ablative radiotherapy by individualized isotoxic dose prescription. JNCIJ. 2016;108:djv305.
- De Ruysscher D, van Baardwijk A, Steevens J, et al. Individualised isotoxic accelerated radiotherapy and chemotherapy are associated with improved long-term survival of patients with stage III NSCLC: a prospective population-based study. Radiother Oncol. 2012;102:228–233.
- Bongers ML, de Ruysscher D, Oberije C, et al. Model-based cost-effectiveness of conventional and innovative chemo-radiation in lung cancer. Int J Technol Assess Health Care. 2017;33:681–690.
- van Wijk Y, Vanneste BGL, Walsh S, et al. Development of a virtual spacer to support the decision for the placement of an implantable rectum spacer for prostate cancer radiotherapy: Comparison of dose, toxicity and cost-effectiveness. Radiother Oncol. 2017;125:107–112.
- Kupelian PA, Willoughby TR, Reddy CA, et al. Hypofractionated intensity-modulated radiotherapy (70 Gy at 2.5 Gy per fraction) for localized prostate cancer: Cleveland Clinic experience. Int J Radiat Oncol Biol Phys. 2007;68:1424–1430.
- Withers HR, Thames HD, Jr, Peters LJ. A new isoeffect curve for change in dose per fraction. Radiother Oncol. 1983;1:187–191.
- Marzi S, Saracino B, Petrongari MG, et al. Modeling of alpha/beta for late rectal toxicity from a randomized phase II study: conventional versus hypofractionated scheme for localized prostate cancer. J Exp Clin Cancer Res. 2009;28:117.
- Michalski JM, Gay H, Jackson A, et al. Radiation dose-volume effects in radiation-induced rectal injury. Int J Radiat Oncol Biol Phys. 2010;76:S123–S129.
- Appelt AL, Vogelius IR. A method to adjust radiation dose-response relationships for clinical risk factors. Radiother Oncol. 2012;102:352–354.
- Walsh S, van der Putten W. A TCP model for external beam treatment of intermediate-risk prostate cancer. Med Phys. 2013;40:031709.
- Stewart RD, Li XA. BGRT: biologically guided radiation therapy-the future is fast approaching!. Med Phys. 2007;34:3739–3751.
- D'Amico AV, Whittington R, Malkowicz SB, et al. Biochemical outcome after radical prostatectomy, external beam radiation therapy, or interstitial radiation therapy for clinically localized prostate cancer. JAMA 1998;280:969–974.
- Viswanathan AN, Yorke ED, Marks LB, et al. Radiation dose-volume effects of the urinary bladder. Int J Radiat Oncol Biol Phys. 2010;76:S116–S122.
- Lambin P, van Stiphout RG, Starmans MH, et al. Predicting outcomes in radiation oncology–multifactorial decision support systems. Nat Rev Clin Oncol. 2013;10:27–40.
- Chetty IJ, Martel MK, Jaffray DA, et al. Technology for innovation in radiation oncology. Int J Radiat Oncol Biol Phys. 2015;93:485–492.
- Lambin P, Zindler J, Vanneste B, et al. Modern clinical research: How rapid learning health care and cohort multiple randomised clinical trials complement traditional evidence based medicine. Acta Oncol. 2015;54:1289–1300.
- Lambin P, Roelofs E, Reymen B, et al. 'Rapid Learning health care in oncology' – an approach towards decision support systems enabling customised radiotherapy'. Radiother Oncol. 2013;109:159–164.
- Reymen B, van Baardwijk A, Wanders R, et al. Long-term survival of stage T4N0-1 and single station IIIA-N2 NSCLC patients treated with definitive chemo-radiotherapy using individualised isotoxic accelerated radiotherapy (INDAR). Radiother Oncol. 2014;110:482–487.
- Hoogeman MS, van Herk M, de Bois J, et al. Strategies to reduce the systematic error due to tumor and rectum motion in radiotherapy of prostate cancer. Radiother Oncol. 2005;74:177–185.
- Pinkawa M, Klotz J, Djukic V, et al. Learning curve in the application of a hydrogel spacer to protect the rectal wall during radiotherapy of localized prostate cancer. Urology. 2013;82:963–968.
- Lambin P, Zindler J, Vanneste BG, et al. Decision support systems for personalized and participative radiation oncology. Adv Drug Deliv Rev. 2017;109:131–153.
- Vanneste BG, Pijls-Johannesma M, Van De Voorde L, et al. Spacers in radiotherapy treatment of prostate cancer: is reduction of toxicity cost-effective? Radiother Oncol. 2015;114:276–281.