Introduction
Radiation therapy (RT) for locally advanced head and neck cancer (LAHNC) can lead to many long-term complications, such as xerostomia, swallowing dysfunction, osteoradionecrosis, and vascular stenosis [Citation1]. These complications have been extensively studied to characterize radiation tolerance of normal tissues; treatment planning goals to reduce the morbidity of RT are widely accepted [Citation2–4]. The classic brain complication of RT in LAHNC patients is radiation necrosis, thus current treatment planning goals are focused on limiting areas of high dose exposure (e.g., limit maximum brain point dose to less than 60 Gy). But apart from brain radionecrosis, the dose-volume effects of brain radiation exposure for LAHNC remain poorly understood. As a result, few metrics are available to help radiation oncologists to assess the plan quality.
The neurocognitive effects of low and moderate dose exposure to cranial radiation remain significantly understudied among LAHNC survivors compared to the populations of childhood cancer and adults with brain tumors, where even low dose cranial radiation has been associated with neurocognitive impairment [Citation5–8] and impaired quality of life (QoL) [Citation9–12]. A preliminary understanding of the radiation dose distribution associated with modern treatment planning and delivery techniques is therefore needed to aid in developing future studies assessing the cognitive effects of RT in LAHNC patients. Since the cognitive function ascribed to distinct brain regions are well-established for other diseases such as childhood cancer and an adult-onset brain tumor, characterizing brain dosimetry of LAHNC plans with greater anatomic detail may help in identifying cognitive domains which are most likely to be negatively affected.
To gain a better understanding of brain dosimetry of modern LAHNC treatment plans, we sought to perform detailed brain segmentation of treatment plans for a cohort of randomly-selected patients who received definitive RT for locally-advanced nasopharyngeal carcinoma and tonsillar carcinoma. We identified the superior, middle and inferior temporal gyri, medial temporal cortex (which contains the subgranular zone and hippocampus) and cerebellar lobes as the brain regions which are most likely to receive clinically-significant radiation doses that were also identifiable on computed tomography (CT) treatment planning scans. Herein, we report the dosimetric results and show how this information may be used to determine the association between brain region-specific delivered dose and impairment in specific cognitive domains in LAHNC survivors treated with RT.
Material and methods
We randomly selected 15 patients treated with definitive intensity-modulated RT (IMRT) for locally advanced (T3-T4 or N1-N3) squamous cell carcinoma of the nasopharynx and 15 patients treated with definitive RT for locally advanced (T3-T4 or N1-N3) squamous cell carcinoma of the tonsil. Both sliding window IMRT and volumetric modulated arc therapy were allowed. A description of the stage and treatment plan for each case is included as .
Table 1. Summary of sublobar brain anatomy dosimetry.
Treatment planning CT scans were reviewed in Varian Eclipse software (Varian Medical Systems, Palo Alto, CA, USA). The superior temporal gyrus (STG), middle temporal gyrus (MTG), inferior temporal gyrus (ITG), medial temporal lobe (MTL), anterior cerebellar lobes (ACL), posterior cerebellar lobes (PCL), and vermis were segmented by a radiation oncologist (McDonald) using a reference atlas [Citation13] () and reviewed for accuracy by a neuroradiologist (Milner). The MTL was defined as the area of the temporal lobes apart from the STG, MTG and ITG structures; the MTL, therefore, contains the hippocampal and other subcortical regions [Citation14]. These structures were felt to comprise the most consistently identifiable sublobar anatomy respecting the limited brain resolution of LAHNC CT simulation scans due to the larger field-of-view needed to include the shoulders.
Figure 1. Example case of sublobar brain anatomy delineation: STG (yellow), MTG (pink), ITG (blue), MTL (orange), ACL (light blue), PCL (magenta), and vermis (green).
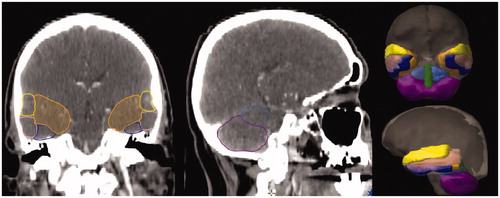
The dose-volume histogram (DVH) for each structure was calculated from the original treatment plan. For each structure, the maximum dose, mean dose, percent receiving 30 Gy (V30Gy[%]) and percent receiving 10 Gy (V10Gy[%]) were extracted for all the patients and descriptive statistics were calculated.
Results
All 30 cases met our institutional goal of brain DMax less than 60 Gy. Any other brain dosimetry planning goals used in the planning process were at the discretion of the treating radiation oncologist. A summary of the dosimetry of all contoured structures, stratified by tumor type, is presented as .
presents the three composite DVHs (minimum, mean and maximum) of each supratentorial brain region segmented for each case, stratified by the tumor location. Among patients with tonsillar carcinoma, the temporal lobes typically did not receive doses >10 Gy, but exceptions were noted with respect to ITG. For nasopharyngeal carcinoma, the low dose spill on the order of 10 Gy to the ITG, MTG and MTL was nearly universal (as a corollary of tumor location) but the 30 Gy isodose volume to these regions varied considerably as did dose to the STG.
Figure 2. Dose-volume histograms of supratentorial brain structures. The minimum, mean, and maximum values were calculated at each 1 Gy dose increment to create each dose-volume histogram.
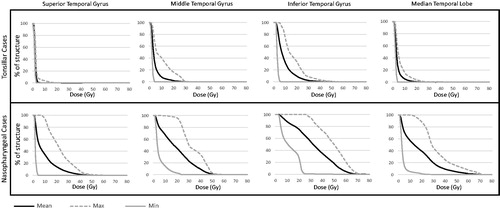
presents three composite DVHs of each infratentorial brain region segmented for each case, stratified by tumor location. Across both tumor types, the dose to the posterior fossa was highly variable. A number of plans were noted where doses of 30 Gy included a significant portion of the cerebellum, though, in patients with tonsillar carcinoma, the 30 Gy isodose volume was typically confined to the PCL as opposed to more diffuse cerebellar spill observed in the nasopharyngeal carcinoma cases.
Figure 3. Dose-volume histograms of infratentorial brain structures. The minimum, mean, and maximum values were calculated at each 1 Gy dose increment to create each dose-volume histogram.
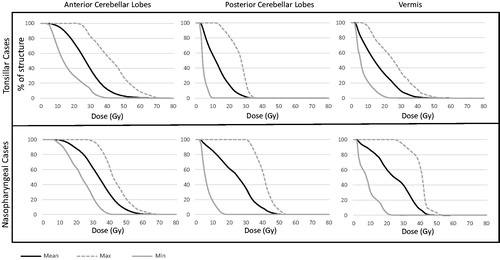
The proposed research battery is presented in and was developed based on the neurocognitive processes subserved by the temporal and cerebellar regions. Specifically, targeted sensory-perceptual testing can assess for auditory or visual neglect caused by a temporal lesion or apraxias caused by a cerebellar lesion. In addition to sensory-perceptual testing, this targeted battery includes validated standardized neuropsychological measures with large normative samples across the adult lifespan. These measures can be utilized such that the patient with LAHNC can be compared to normative data that take into account age, gender and ethnicity, in order to determine if he/she is performing below expectation. Each test is listed by the construct it measures and is separated out by temporal lobe functions for the patients with nasopharyngeal carcinomas and by cerebellar regions functions for the patients with tonsillar carcinomas.
Table 2. Proposed neurocognitive screening battery for patient with LAHNC post-RT. We recommend referral for more comprehensive neuropsychological testing if deficits are found in two or more of these areas assessed.
Discussion
In this manuscript, we assessed sublobar brain radiation dosimetry within the temporal and cerebellar cortices in a randomly-selected sample of locally advanced tonsil and nasopharyngeal radiation therapy plans. The main intent of this brief report is to describe the types of dose distributions that occur in a typical population of the patients treated with definitive RT for locally advanced nasopharynx or tonsil tumors. Assuming that our random sample is representative of the larger universe of RT plans for LAHNC, we noted various characteristics about brain dosimetry that may be useful to inform the design of future cognitive outcome studies in this population. Indeed, objective evidence of cognitive impairment following RT for LAHNC has been reported [Citation15], but the relationship between the degree and severity of impairment and radiation dosimetry is unclear. Furthermore, the total radiation dose and dose distribution in LAHNC plans are quite different from primary brain tumor plans and, as a result, the cognitive effects of RT require specific study among LAHNC survivors. Developing a basic understanding of the brain radiation dose distribution associated with LAHNC sub-sites can help tailor a cognitive battery designed to detect the most likely deficits.
As expected, a number of nasopharyngeal carcinoma cases were associated with significant exposure to the temporal lobes. Within the temporal lobes, the greatest exposure was to the MTG, ITG and MTL, with mean doses exceeding 20 Gy in the majority of the cases across the cohort. The mean dose to the STG was lower than the other areas of the temporal lobe, but V30Gy [%] was greater than 25% in more than half of the cases. Despite the fact that the pterygoid plates are routinely included within the clinical target volume for tonsillar carcinomas [Citation16, Citation17], any temporal lobe exposure to the 30 Gy isodose volume was almost always limited to ITG. At least qualitatively, brain radiation exposure was primarily limited to the posterior fossa in the tonsillar cases. The temporal lobes are involved in a number of cognitive processes, with its primary functions related to the processing of auditory input (especially verbal auditory input of the language-dominant temporal lobe (STG)), visual recognition (ITG) and memory functions (MTL) [Citation18].
The cerebellar exposure of the PCL among nasopharyngeal carcinoma cases, with a median V30Gy [%] of 73.1% was significant. Furthermore, the ACL and vermis were also generally exposed to mean doses exceeding 20 Gy. Among the patients with tonsillar carcinoma, cerebellar exposure above 30 Gy was primarily within the PCL, with a median V30Gy[%] of 35.7%, likely due to the inferior extent of the PCL when compared to the ACL and vermis. Research from neuroimaging studies has shown the cerebellum to be involved in more than just motor functioning [Citation19]. Additional cognitive functions thought to be served by the cerebellum include sensorimotor tasks (ACL), working memory and executive functioning (PCL), as well as emotional processing (vermis).
As suggested above, both nasopharyngeal and tonsillar carcinoma cases would benefit from targeted cognitive screening in order to determine which patients would best benefit from more comprehensive neuropsychological follow-up and who are at the highest risk for development of cognitive deficits due to RT. This brief report indicates that specific attention should be directed to certain cognitive functions based on both tumor location and differences in dose distribution characteristics. Using a more tailored battery in future studies of cognitive outcomes in LAHNC survivors may increase the likelihood of detecting changes missed by a more generic screening battery. Based on our observations in this small cohort, we recommend that special attention is paid to visual, motor, and memory domains in patients with nasopharyngeal carcinoma, and processing speed, memory, and motor domains in patients with tonsillar carcinoma.
Supplemental Material
Download MS Word (15.4 KB)Disclosure statement
No potential conflict of interest was reported by the authors.
References
- Strojan P, Hutcheson KA, Eisbruch A, et al. Treatment of late sequelae after radiotherapy for head and neck cancer. Cancer Treat Rev. 2017;59:79–92.
- Deasy JO, Moiseenko V, Marks L, et al. Radiotherapy dose-volume effects on salivary gland function. Int J Radiat Oncol Biol Phys. 2010;76:S58–S63.
- Rancati T, Schwarz M, Allen AM, et al. Radiation dose-volume effects in the larynx and pharynx. Int J Radiat Oncol Biol Phys. 2010;76:S64–SS9.
- Marks LB, Yorke ED, Jackson A, et al. Use of normal tissue complication probability models in the clinic. Int J Radiat Oncol Biol Phys. 2010;76:S10–SS9.
- Saad S, Wang TJ. Neurocognitive deficits after radiation therapy for brain malignancies. Am J Clin Oncol. 2015;38:634–640.
- Spiegler BJ, Kennedy K, Maze R, et al. Comparison of long-term neurocognitive outcomes in young children with acute lymphoblastic leukemia treated with cranial radiation or high-dose or very high-dose intravenous methotrexate. JCO. 2006;24:3858–3864.
- Lawrence YR, Li XA, el Naqa I, et al. Radiation dose–volume effects in the brain. Int J Radiat Oncol Biol Phys. 2010;76:S20–SS7.
- Greene-Schloesser D, Robbins ME, Peiffer AM, et al. Radiation-induced brain injury: a review. Front Oncol. 2012;2:73.
- Scoccianti S, Detti B, Cipressi S, et al. Changes in neurocognitive functioning and quality of life in adult patients with brain tumors treated with radiotherapy. J Neuro-Oncol. 2012;108:291–308.
- Butler JM, Rapp SR, Shaw EG. Managing the cognitive effects of brain tumor radiation therapy. Curr Treat Options in Oncol. 2006;7:517–523.
- Doyle M, Bradley NM, Li K, et al. Quality of life in patients with brain metastases treated with a palliative course of whole-brain radiotherapy. J Palliat Med. 2007;10:367–374.
- Steinmann D, Paelecke-Habermann Y, Geinitz H, et al. Prospective evaluation of quality of life effects in patients undergoing palliative radiotherapy for brain metastases. BMC Cancer. 2012;12:283.
- Micheau AH, Hoa D. Brain and face CT: interactive anatomy atlas. 2017. Available from: https://www.imaios.com/en/e-Anatomy/Head-and-Neck/Head-CT.
- Squire LR, Stark CE, Clark RE. The medial temporal lobe. Annu Rev Neurosci. 2004;27:279–306.
- Welsh LC, Dunlop AW, McGovern T, et al. Neurocognitive function after (chemo)-radiotherapy for head and neck cancer. Clinic Oncol (R Coll Radiol). 2014;26:765–775.
- Gregoire V, Eisbruch A, Hamoir M, et al. Proposal for the delineation of the nodal CTV in the node-positive and the post-operative neck. Radiother Oncol. 2006;79:15–20.
- Dawson LA, Anzai Y, Marsh L, et al. Patterns of local-regional recurrence following parotid-sparing conformal and segmental intensity-modulated radiotherapy for head and neck cancer. Int J Radiat Oncol Biol Phys. 2000;46:1117–1126.
- Kolb B, Whishaw IQ. Fundamentals of human neuropsychology. Macmillan; 2009.
- Stoodley CJ, Schmahmann JD. Functional topography in the human cerebellum: a meta-analysis of neuroimaging studies. NeuroImage. 2009;44:489–501.