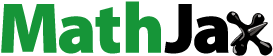
Abstract
Background: Late effects induced by radiotherapy (RT) are of great concern for mediastinal Hodgkin’s lymphoma (HL) patients and it is therefore important to reduce normal tissue dose. The aim of this study was to investigate the impact on the normal tissue dose and target coverage, using various combinations of intensity modulated proton therapy (IMPT), volumetric modulated arc therapy (VMAT) and 3-dimensional conformal RT (3D-CRT), planned in both deep inspiration breath hold (DIBH) and free breathing (FB).
Material and methods: Eighteen patients were enrolled in this study and planned with involved site RT. Two computed tomography images were acquired for each patient, one during DIBH and one during FB. Six treatment plans were created for each patient; 3D-CRT in FB, 3D-CRT in DIBH, VMAT in FB, VMAT in DIBH, IMPT in FB and IMPT in DIBH. Dosimetric impact on the heart, left anterior descending (LAD) coronary artery, lungs, female breasts, target coverage, and also conformity index and integral dose (ID), was compared between the different treatment techniques.
Results: The use of DIBH significantly reduced the lung dose for all three treatment techniques, however, no significant difference in the dose to the female breasts was observed. Regarding the heart and LAD doses, large individual variations were observed. For VMAT, the mean heart and LAD doses were significantly reduced using DIBH, but no significant difference was observed for 3D-CRT and IMPT. Both IMPT and VMAT resulted in improved target coverage and more conform dose distributions compared to 3D-CRT. IMPT generally showed the lowest organs at risk (OAR) doses and significantly reduced the ID compared to both 3D-CRT and VMAT.
Conclusions: The majority of patients benefited from treatment in DIBH, however, the impact on the normal tissue dose was highly individual and therefore comparative treatment planning is encouraged. The lowest OAR doses were generally observed for IMPT in combination with DIBH.
Introduction
There has been a tremendous development in the treatment of Hodgkin’s lymphoma (HL) the last couple of decades, primarily through the introduction of combined modality treatment consisting of both chemotherapy and radiotherapy (RT), resulting in survival rates above 90% [Citation1,Citation2]. Due to the relatively high survival rate and young age for most HL patients, a large proportion of the patients become long-time survivors and hence late effects of the treatment are of great concern. Previously, extended field radiotherapy (EFRT) and involved field radiotherapy (IFRT) were used to treat entire nodal stations, which has been shown to result in increased mortality from late effects, most commonly from secondary cancer, cardiovascular diseases and respiratory diseases [Citation3–6]. Modern HL treatment has focused on reducing the risk of late effects while still maintaining high disease control [Citation7]. This has been achieved through reducing the prescribed dose [Citation1,Citation2], and the irradiated volume, by introducing the involved node radiotherapy (INRT) and involved site radiotherapy (ISRT) concepts where only the initially involved lymph nodes are included in the target [Citation8]. Also, more advanced image guidance and conform treatment techniques, such as 3-dimensional conformal radiation therapy (3D-CRT), intensity modulated radiation therapy (IMRT), volumetric modulated arc therapy (VMAT) and helical tomotherapy, have been introduced in HL RT [Citation9–11]. The dynamic treatment techniques provide a very conformal high-dose distribution, however at the expense of larger healthy tissue volumes being irradiated to low doses. Hence, new techniques that enable a reduction of the normal tissue irradiation for all dose levels would be desirable. Deep inspiration breath hold (DIBH) has demonstrated the ability to reduce dose to healthy tissue for mediastinal HL, due to favorable anatomical changes in tumor position in relation to healthy tissue [Citation12–16]. Also, proton therapy (PT) has shown a positive impact on the normal tissue dose compared to photon therapy [Citation6,Citation17–19]. Most studies for mediastinal HL have used the passive scattering technique, however, more modern PT, using pencil beam scanning (PBS) and intensity modulated PT (IMPT), enables even more conformal treatment plans. Also, automatic beam triggering is nowadays available for PT, enabling gated beam delivery in DIBH [Citation20]. To our knowledge, only two previous treatment planning studies exist on the use of both PT and DIBH for mediastinal HL, showing an overall benefit for the combined use of the techniques [Citation21,Citation22]. Thus, further investigations of the combined use of PT and DIBH are highly desirable. The aim of this study was to investigate the impact on the normal tissue dose and target coverage, using various combinations of IMPT, VMAT and 3D-CRT planned in both DIBH and free breathing (FB). To our knowledge, no previous study exists that have investigated all these treatment techniques in both FB and DIBH for mediastinal HL.
Material and methods
Patients
Between April 2014 and June 2017, 19 patients with mediastinal HL were enrolled in this retrospective comparative dose planning study. One patient was excluded from the study because the target volumes were not deemed comparable between the two computed tomography (CT) images acquired due to insufficient image quality. provides the detailed characteristics of the patient cohort, including age, gender, stage and disease location. The patients were classified as unfavorable if they had one or more risk factors according to the Swedish national treatment guidelines for HL (www.cancercentrum.se). Risk factors for stage IA and IIA are bulky disease, >2 involved sites and erythrocyte sedimentation rate ≥50. Risk factors for stage IIB-IV are male, <45 years, stage IV, hemoglobin <105 g/L, S-albumin <40 g/L, leukocytes >15 × 109/L and B-lymphocytes <8% or <0.6 × 109/L. Residual PET-positive disease after chemotherapy was also considered a risk factor. Only disease above the diaphragm was included in this study.
Table 1. Patient and disease characteristics.
Ethical consideration
The use of the RT database for retrospective research has been approved by the committee of the Regional Ethical Review Board in Lund (No. 2013/742).
CT imaging and deep inspiration breath hold techniques
Two CT images were acquired for treatment planning and dosimetric comparison purposes, one during DIBH and one during FB. The patients were positioned supine, generally in immobilizing fullbody vacuum bags, with the arms positioned above the head. However, two patients were positioned with the arms along the side of the body. The CT images were acquired using a Siemens Somatom definition AS plus (Siemens Medical Solutions, Erlangen, Germany) with a slice thickness of 3 mm. For improved image quality, contrast-enhanced CT (60 ml, VisipaqueTM 270 mg I/ml, Iodixanol, GE Healthcare AS, Oslo, Norway) was acquired for eight of the patients, two in FB and six in DIBH.
The real-time position management (RPM) system (Varian Medical Systems, Palo Alto, CA) or the optical surface scanning system Sentinel (C-rad Positioning AB, Uppsala, Sweden) was used to track the respiratory motion for the CT scan in DIBH. The RPM system and the Sentinel system have previously been described elsewhere [Citation23,Citation24]. All patients were trained by an oncology nurse to perform deep inspiration at a comfortable and reproducible level prior to the CT scan. For the first 10 patients, audio-coached enhanced inspiration gating (EIG) was used in combination with the RPM-system to acquire the DIBH CT images [Citation23]. The patients were repeatedly deep breathing for approximately 4–5 seconds throughout the CT scan. For each inspiration, a smaller sequenced segment of the CT scan length was acquired with an axial CT scan mode. These segments were appended to each other to assess the full scan volume. For the remaining eight patients, the Sentinel system was used to acquire the DIBH CT images, using both audio and visual guidance [Citation24]. A helical CT scan was acquired during a single DIBH. For all patients, the respiratory motion was tracked at sternum, at the surface above the xiphoid process. The median deep inspiration amplitude was 11.7 mm (range 9.0–20.7 mm) and the median gating window was 3.2 mm (range 2.7–4.3 mm). The same criteria for the amplitude and gating window were used for all patients in this study, regardless of the breathing technique or gating system used, resulting in equal DIBH CT images. Both EIG and DIBH are therefore referred to as DIBH.
Contouring
Target volumes were delineated using the ISRT technique [Citation8], based on a 18F-fluoro-deoxyglucose (FDG) positron emission tomography (PET)/CT image acquired during FB (GE Discovery 690, General Electric Healthcare and Philips Gemini TF 16, Philips Medical Systems, Amsterdam, Netherlands). The information from the PET images were transferred to the FB and DIBH planning CTs through visual comparison between the images. All structures were delineated in both the DIBH and FB CT-sets by the same radiation oncologist. The clinical target volume (CTV) was defined as involved lymph node stations, to which radiation treatment was warranted. The median CTV volume was 338 cm3 (range 124–1530 cm3) in FB and 341 cm3 (range 123–1381 cm3) in DIBH. A planning target volume (PTV) was constructed with an 8 mm isotropic margin to CTV, and cropped 4 mm from the skin surface. The organs at risk (OAR) delineated were the heart, the left anterior descending (LAD) coronary artery, lungs, and for female patients also the breasts. The lungs were automatically delineated using the segmentation wizard in the treatment planning system (TPS, Eclipse, version 13.6, Varian Medical Systems, Palo Alto, CA) and then manually verified. All other structures were delineated manually. The heart was defined as the entire myocardium including the big vessels up to the departure of the coronary arteries from aorta ascendens. LAD was delineated with a 6 mm diameter from the vessels departure from aorta as far as it could be visualized, often to the middle of the heart. The female breasts were outlined as the clinical limits of the breast, including all glandular tissue and cropped 5 mm from the skin surface. All OARs were delineated without margins.
Treatment planning
Six treatment plans were created for each patient; 3D-CRT in FB, 3D-CRT in DIBH, VMAT in FB, VMAT in DIBH, IMPT in FB and IMPT in DIBH, resulting in a total of 108 treatment plans. All treatment plans were created in the Eclipse TPS (version 13.6, Varian Medical Systems, Palo Alto, CA). The prescribed dose was 29.75 Gy/Gy(RBE) (relative biological equivalent) in 17 fractions normalized to the mean dose of the PTV, assuming a constant RBE of 1.1 for protons. The main goals creating the treatment plans were that 100% of the PTV volume should be covered by 95% of the prescribed dose, while the volume receiving more than 105% of the prescribed dose was minimized. At the same time, the dose to the OARs was kept as low as possible. To achieve the best possible dose distributions, each treatment plan was individually optimized.
3 D-CRT plans
For the 3D-CRT plans, two 6 MV anterior–posterior/posterior–anterior (AP/PA) parallel opposed fields were generally used (gantry angles 0° and 180°), with a PTV-to-field-edge margin of 7 mm. To individually optimize each plan, the beams were weighted and if needed wedges and/or supplementary fields (6 or 10 MV) were used to improve dose homogenization in the target volume. The 3D-CRT plans were generated for either a TrueBeam linear accelerator (version 2.0, Varian Medical Systems, Palo Alto, CA) or an Elekta Synergy linear accelerator (Elekta AB, Stockholm, Sweden). The Anisotropic Analytical Algorithm (AAA, version 13.6) was used for dose calculation.
VMAT plans
Basic VMAT plans with standard arc geometries were created. All plans were created with 6 MV using two, three or four arcs depending on patient anatomy and PTV volume. Depending on the resulting field size (x-direction), some fields were modified so that the field size in the x-direction for any given arc had a maximum size of 15 cm. For arcs with field size (x-direction) less or equal to 15 cm two arcs were typically used. For modified arcs with original field size larger than 15 cm, a third arc was typically added to maintain plan quality. In one case with a very large PTV, four arcs were used. All plans were optimized using the Photon Optimizer (version 13.6) for a TrueBeam linear accelerator and final dose was calculated with AAA (version 13.6). Full arcs with collimator angles 5° and 355° were typically used, but for lateral targets where full arcs would cause collision with the patient or couch the arc length was modified. In one case with four arcs collimator rotation 85° and 95° in addition to the standard 5° and 355° mentioned above were used.
IMPT plans
Either AP/PA fields (12 patients) or two oblique AP fields in 10° and 350° (six patients) were used, whichever was considered most beneficial for the individual patient. During optimization, constraints were assigned to the PTV and all OARs. The highest priority was set for the PTV and thereafter the heart, LAD and lungs had equal priority. The priority for the female breasts differed slightly between the AP/PA plans and the plans with two oblique AP fields. For the AP/PA plans, the priority was the same as for the heart, LAD and lungs. In the plans with two oblique AP fields, the female breasts had a slightly higher priority than the other OARs, since both fields passed through the breast tissue. High density materials, such as medical implants or contrast agent, were replaced with water equivalent structures for dose calculation purposes. The IMPT plans were created for an IBA PT System (Proteus Plus, IBA, Louvain-la-Neuve, Belgium) with a PBS beam delivery. The Proton Convolution Superposition algorithm (PCS, version 13.7) was used for dose calculation, with beam data from the Swedish proton center, the Skandion clinic (Uppsala, Sweden).
For the IMPT plans, additional margins for the range uncertainty was calculated as 3.5% of the range to the distal edge of the CTV plus 1 mm. If the range uncertainty exceeded the CTV-to-PTV margin of 8 mm, an additional margin was added. Otherwise the same PTV as for the photon plans was used. For the PA field for one patient in FB and four patients in DIBH, the distal margin exceeded the CTV-to-PTV margin by 1 mm and thus the margin was expanded 1 mm in this direction.
Treatment
All patients managed the DIBH CT acquisition and were clinically treated with a 3D-CRT plan in either DIBH or FB, whichever was considered most beneficial for the patient. Ten patients were treated using DIBH 3D-CRT and the remaining eight patients with FB 3D-CRT. During EIG and DIBH treatment, the respiratory motion was tracked with the TrueBeam integrated gating systems, Polaris Spectra infrared camera (Northern Digital Medical, Waterloo, Canada) or the optical surface scanning system Catalyst (C-rad Positioning AB, Uppsala, Sweden) [Citation24]. The patient position was verified with orthogonal X-ray imaging or cone beam CT (CBCT). The CBCTs were acquired during three or four DIBHs.
Dosimetric and statistical analysis
Dose volume histograms (DVH) were extracted for all treatment plans from the TPS, and average DVHs for all patients were calculated for all the treatment techniques. To compare the dose distributions for the different treatment techniques, the mean dose to the heart (Dmean,heart), LAD (Dmean,LAD), lungs (Dmean,lungs), right breast (Dmean,right breast) and left breast (Dmean,left breast), the dose received by 2% of the heart and LAD volumes (D2%,heart and D2%,LAD), the lung volume receiving 5 and 20 Gy (V5Gy,lungs and V20Gy,lungs) and the CTV and PTV volume covered by 95% of the prescribed dose (V95%,CTV and V95%,PTV) were retrieved. For comparison of the PTV dose uniformity, the heterogeneity index (HI) was calculated (EquationEquation (1)(1)
(1) ).
(1)
(1)
where D2% and D98% are the doses to 2% and 98% of the volume, respectively.
Further, to compare the dose to healthy tissue between the 3D-CRT, VMAT and IMPT plans the integral dose (ID) was evaluated (EquationEquation (2)(2)
(2) ).
(2)
(2)
where
is the density and V is the volume. The ID was calculated for the full CT scanned body volume excluding the PTV volume and was corrected for the lung density. Generic density values of 0.26 g/cm3 for the lung and 1.06 g/cm3 for all other tissues were used [Citation25].
The plan conformity was evaluated using Paddick’s conformity index (CIPaddick) [Citation26] (EquationEquation (3)(3)
(3) ).
(3)
(3)
where TV is the target volume, PIV is the prescription isodose volume and TVPIV is the target volume covered by the prescription isodose. In this study, the TV corresponds to the PTV and PIV the 95% isodose volume. A perfectly conformal plan has a CIPaddick value of 1.
Friedman tests were carried out to investigate if there were any statistical significant differences between the six different treatment techniques (FB 3D-CRT, DIBH 3D-CRT, FB VMAT, DIBH VMAT, FB IMPT and DIBH IMPT). If a statistically significant difference was observed, post hoc two-sided paired Wilcoxon’s tests were carried out. A significance level of 0.05 was used. Multiple testing was not accounted for because it was considered too conservative due to the large number of comparisons.
All dosimetric and statistical analyses were performed in Matlab (R2014b, Mathworks, Natick, MA).
Results
All dosimetric parameters are presented in and the average DVHs for the target volumes and OARs are presented in . The mean OAR doses for each individual patient are presented in , where a large variation in the OAR doses between the individual patients can be observed.
Figure 1. Average dose volume histograms for all patients comparing deep inspiration breath hold (DIBH, solid lines) and free breathing (FB, dashed lines) as well as intensity modulated proton therapy (IMPT, red), volumetric modulated arc therapy (VMAT, green) and 3-dimensional conformal radiation therapy (3D-CRT, blue) for the (a) CTV, (b) PTV, (c) lungs, (d) heart, (e) left anterior descending (LAD) coronary artery, (f) right breast and (g) left breast.
Figure 2. The mean dose to the (a) lungs, (b) heart, (c) LAD, (d) right breast and (e) left breast for free breathing (FB) 3-dimensional conformal radiation therapy (3D-CRT), deep inspiration breath hold (DIBH) 3D-CRT, FB volumetric modulated arc therapy (VMAT), DIBH VMAT, FB intensity modulated proton therapy (IMPT) and DIBH IMPT. The values for each individual patient are shown in black and the median values for the whole patient cohort in red.
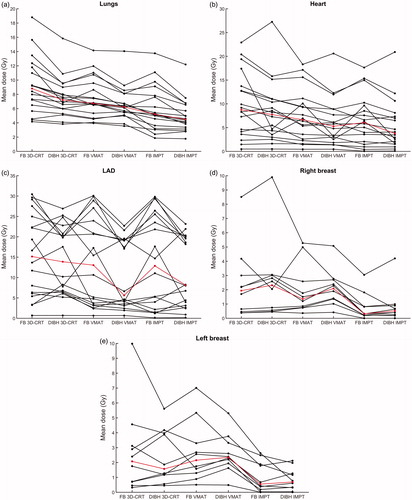
Table 2. Dosimetric parameters for free breathing (FB) and deep inspiration breath hold (DIBH) 3-dimensional conformal radiation therapy (3D-CRT), volumetric modulated arc therapy (VMAT) and intensity modulated proton therapy (IMPT).
Comparison of DIBH and FB
Lungs
Regarding the lung dose, the vast majority of patients benefited from DIBH, with reduced Dmean,lungs for 94, 83 and 94% of the patients for 3D-CRT, VMAT and IMPT, respectively (). For 3D-CRT, VMAT and IMPT the median Dmean,lungs, V20Gy,lungs and V5Gy,lungs were significantly reduced by approximately 10–20% for DIBH compared to FB.
Heart and LAD
Regarding both the heart and LAD doses, the benefit of using DIBH varied substantially between the patients (). The median Dmean,heart and Dmean,LAD were reduced by approximately 10–40% and 10–60%, respectively, for DIBH compared to FB, however, this difference only reached statistical significance for VMAT. For DIBH compared to FB, both the Dmean,heart and Dmean,LAD decreased for 61% of the patients for 3D-CRT and IMPT, and 72% of the patients for VMAT. No significant differences in D2%,heart and D2%,LAD were observed between DIBH and FB for neither 3D-CRT, VMAT nor IMPT.
Breasts
There was no significant difference in the median Dmean,right breast and Dmean,left breast between DIBH and FB for neither 3D-CRT, VMAT nor IMPT.
Target coverage
Very similar target coverage was achieved between DIBH and FB for all treatment techniques (). For 3D-CRT and VMAT, no significant difference was observed in V95%,CTV or V95%,PTV between DIBH and FB. For IMPT, however, small but statistically significant decreases in V95%,CTV and V95%,PTV were observed for DIBH.
Comparison of IMPT, VMAT and 3D-CRT
Lungs
For both DIBH and FB, the lowest median Dmean,lungs was observed for IMPT, followed by VMAT and 3D-CRT (). The Dmean,lungs was reduced by approximately 40% and 20–30% for IMPT compared to 3D-CRT and VMAT, respectively. The lowest V20Gy,lungs was observed for VMAT, followed by IMPT and 3D-CRT, and the lowest V5Gy,lungs was observed for IMPT, followed by 3D-CRT and VMAT. All these differences were statistically significant. Hence, compared to 3D-CRT, IMPT reduced the irradiated lung volume for all dose levels, while VMAT reduced the lung volume receiving high doses, however at the cost of increasing the low dose volume ().
Heart and LAD
For both DIBH and FB, the lowest median Dmean,heart was observed for IMPT, followed by VMAT and 3D-CRT (), with all differences being statistically significant. The Dmean,heart was reduced by approximately 30–50% and 10–30% for IMPT compared to 3D-CRT and VMAT, respectively. The median Dmean,LAD was significantly lower for VMAT and IMPT compared to 3D-CRT, however, no significant difference was observed between VMAT and IMPT. No significant differences in D2%,heart and D2%,LAD were observed between any of the treatment techniques.
Breasts
For both DIBH and FB, the median Dmean,right breast and Dmean,left breast were significantly reduced by approximately 60–90% for IMPT compared to both VMAT and 3D-CRT, however, no significant difference was observed between VMAT and 3D-CRT ().
Paddick’s conformity index and integral dose
For both DIBH and FB, the highest CIPaddick was observed for VMAT, followed by IMPT and 3D-CRT, with all differences being statistically significant. The ID was approximately halved for IMPT compared to both VMAT and 3D-CRT.
Target coverage
For both DIBH and FB, the V95%,CTV, V95%,PTV and HIPTV were significantly improved for IMPT and VMAT compared to 3D-CRT (). The target coverage and HI were similar for VMAT and IMPT, however, for some comparisons there were small but statistically significant differences.
Comparison of FB IMPT and DIBH VMAT
The lowest OAR doses were generally observed for the combination of DIBH and IMPT ( and ). However, this combination may not always be feasible and to compare FB IMPT with DIBH 3D-CRT and VMAT is therefore also of clinical interest. Since DIBH VMAT was generally superior to DIBH 3D-CRT, FB IMPT was compared to DIBH VMAT. There was no significant difference in the median Dmean,lungs and Dmean,heart between FB IMPT and DIBH VMAT. However, V20Gy,lungs and Dmean,LAD were significant lower for DIBH VMAT compared to FB IMPT and vice versa for V5Gy,lungs, Dmean,right breast and Dmean,left breast.
Discussion
Treatment using DIBH is widely assessable in modern RT, and was hereby found to be well tolerated and to reduce doses to OARs for the majority of the patients with mediastinal HL for various treatment techniques. For the vast majority of the patients, the mean lung dose was reduced using DIBH. However, larger variations were observed for the mean heart and LAD doses, increasing the dose during DIBH for individual patients (). Regarding the female breasts, no significant difference was observed between DIBH and FB. For IMPT, a small difference was observed in the target coverage between FB and DIBH, which probably is clinically irrelevant. Thus the DIBH and FB plans could be considered comparable regarding the target coverage.
Both IMPT and VMAT improved the target coverage and resulted in more conform dose distributions compared to 3D-CRT. However, IMPT was more beneficial than VMAT, since it reduced the OAR doses for all dose levels, while VMAT reduced the volumes receiving high doses, however, at the expense of increasing the low-dose volumes. Also, the ID, reflecting the total body radiation exposure, was greatly reduced for all patients using IMPT compared to VMAT and 3D-CRT, potentially leading to a lower risk of secondary malignancies [Citation6]. The lowest doses to the OARs were generally observed for the combination of DIBH and IMPT. For LAD, however, the lowest mean dose was observed for DIBH VMAT. The entire LAD is usually located within the high-dose area, and therefore greatly benefits from the excellent conformity achieved by VMAT while it is not affected by the increased low-dose bath.
The use of DIBH increased the lung volume, which resulted in a smaller proportion of the lung included in the treatment fields. Also, the heart was pulled caudally and the mediastinum elongated, which resulted in an increased separation between the heart and the target volume for most patients. However, this study showed that the desired separation between the heart and target volume was not always achieved with DIBH. For a few of the patients, the overlap between the heart and target volume was instead increased during DIBH, which resulted in higher heart doses. This effect was most pronounced for 3D-CRT, since the dose distribution was less conformed compared to VMAT and IMPT. The anatomical changes during DIBH were highly individual, and for some patients there were none or only a small overlap between the heart and target volume in FB, limiting the possible heart dose reduction for DIBH. For patients with an overlap between the heart and target volume, deformations during DIBH led to more or less advantageous positions with regards to treatment beams. Hence, the impact of DIBH on the heart dose for mediastinal HL is highly individual and cannot be predicted in advance. Therefore, CT images in both FB and DIBH should be acquired for each patient and comparative treatment planning performed. However, large heart and LAD dose reductions were observed using DIBH for individual patients. For example, one of the patients had relative mean heart and LAD dose reductions of approximately 70% and 30–40% using DIBH for all three treatment techniques.
Several studies have compared treatment of mediastinal HL in DIBH and FB for photon therapy, both for 3D-CRT and IMRT [Citation13–16]. The reduced lung dose observed in this study using DIBH confirms the results of the prior studies. However, previous studies have shown a significant decrease in the mean heart dose for DIBH, which was only observed for VMAT in this study, although a decrease was observed for most patients for the other treatment techniques as well. The study by Petersen et al. [Citation14] was the only one that investigated the LAD dose and they showed a significant reduction in the mean LAD dose using DIBH, which was only observed for VMAT in this study. Possible reasons for the difference in heart and LAD doses could be that smaller treatment margins were used for DIBH compared to FB in some of the studies, different target localization in mediastinum and that different photon treatment delivery techniques (3D-CRT/IMRT/VMAT) were used. In accordance with our study, Petersen et al. [Citation14] and Aznar et al. [Citation16] did not find any significant difference in the mean breast dose between DIBH and FB. Charpentier et al. [Citation15], however, reported a significant increase in the mean breast dose for DIBH compared to FB.
Regarding the use of PT, most previous studies for mediastinal HL have investigated the impact on normal tissue dose using the passive scattering technique in FB. In accordance to this study, they showed that the dose to the lungs, heart and breasts was generally reduced for proton compared to photon therapy [Citation6,Citation17–19]. However, in this study, the more advanced delivery technique PBS, which enables even larger normal tissue sparing, has been used. In a review article, Tseng et al. [Citation6] present a weighted average OAR dose comparison of 14 studies between PT (passive scattering and PBS), modern RT (IMRT, VMAT and tomotherapy) and 3D-CRT during FB. The Dmean,heart, Dmean,breasts and Dmean,lungs were reduced by 3.57 Gy, 1.47 Gy and 2.81 Gy for PT compared to 3D-CRT, which is comparable to the dose reductions observed in this study. Comparing PT with modern RT, the corresponding dose reductions were 2.24 Gy, 2.45 Gy and 3.28 Gy, which are slightly larger than the dose reductions observed in this study. Tseng et al. concluded that a larger dose reduction could be achieved for PT compared to modern RT than for modern RT compared to 3D-CRT for all OARs. In this study, however, a larger benefit of using modern RT (VMAT) compared to 3D-CRT was observed, thus reducing the differences between PT (IMPT) and modern RT (VMAT). However, a clear disadvantage of VMAT is the increased low-dose bath.
To our knowledge, only two previous studies have investigated the combined use of DIBH and PT for mediastinal HL [Citation21,Citation22]. However, no previous study as comprehensive as this exists, comparing DIBH and FB for IMPT, VMAT and 3D-CRT for the same patient cohort. Rechner et al. [Citation22] compared PT and IMRT for both DIBH and FB. Both this study and Rechner et al. showed the lowest OAR doses for the combined use of DIBH and PT. In contrary to what was observed in this study, they showed a significant reduction in the mean heart and LAD doses and no significant difference in the mean lung dose, comparing DIBH and FB for PT. Also, they found no significant difference in the heart and lung mean dose between DIBH photon therapy and FB PT. In this study, however, significant differences in favor of FB IMPT were found compared to DIBH 3D-CRT, but not compared to DIBH VMAT. Generally, a larger benefit on normal tissue dose for proton compared to photon therapy was observed in this study, whereas, Rechner et al. found a larger benefit of DIBH compared to FB. Possible reasons for the differences observed between the studies could be different target localization in mediastinum, the use of different treatment techniques (single field uniform dose/IMPT and 3D-CRT/IMRT/VMAT) and that smaller margins were used in the superior–inferior direction for DIBH by Rechner et al., whereas, in this study the same margins for FB and DIBH were used.
Baues et al. [Citation21] is the only previous study that has compared IMPT and VMAT during DIBH for mediastinal HL, however, they did not make a comparison between DIBH and FB. They showed reduced mean doses of 38–83% for the lungs, heart and breasts using IMPT compared to VMAT, comparable to the reductions of 27–76% observed in this study. Also, they showed a 49% decrease in the mean dose to healthy tissue, corresponding to the 47% decrease in ID observed in this study. However, they showed a 32% reduction in V20Gy,lungs for IMPT compared to VMAT, whereas a 22% increase was observed in this study.
PET images during DIBH, shown feasible by Petersen et al. [Citation14], were not available in this study. However, to reduce contouring uncertainties, target delineation was performed by the same oncologist and carefully investigated for each patient during FB and DIBH. In order to make a strict comparison between all different treatment techniques, the same CTV-PTV margins were used for FB and DIBH. Also, essentially the same PTV volumes were used for the IMPT plans, with only a small (1 mm) additional margin for range uncertainties added for a few patients. Thus, the dose reductions observed for DIBH were derived only by anatomical changes and not reduced CTV-PTV margins.
A limitation of this study is that intrafractional motion during treatment has not been considered, which has the largest dosimetric effects for IMPT, followed by VMAT and 3D-CRT. Intrafractional motion in combination with VMAT and PBS delivery can lead to unwanted dosimetric interplay effects, resulting in a degradation of the dose distribution to the target and/or OARs [Citation27,Citation28]. For PT, range uncertainties may also negatively impact the dose distribution [Citation27]. However, this was a pure treatment planning study, guiding to what is possible regarding dose sparing to OARs using different treatment techniques, and thus, the plans were not created with regards to robust treatment delivery. Robust optimization could have been used [Citation29,Citation30], however, this technique was not available during the execution of this study. However, robust PBS plans have been shown feasible for mediastinal lymphomas, with minimal impact of interplay effect if repainting and/or larger spot size is used [Citation31]. Further, reduced margins due to mitigation of the target motion during DIBH were not considered in this study. However, the treatment is delivered during several DIBHs, raising concerns about uncertainties in the intrafractional DIBH target position reproducibility [Citation32]. Another limitation is that a constant RBE value of 1.1 was assumed for the IMPT plans according to ICRU recommendations [Citation33], however in reality the RBE increases toward the distal edge of the SOBP [Citation34]. In the case of mediastinal HL, this could potentially increase the biological effective dose to the OARs since they are situated beyond the SOBP and this would depend on the field setting used.
In addition, this study focuses on dosimetric effects, and it is hard to estimate the exact clinical benefit for each individual from the dose distributions obtained for the different treatment techniques. However, as low doses as possible to OARs are always preferable in order to reduce side effects. Especially in these often young patients, with a long life-expectancy, who also receive chemotherapy which may increase risk of cardiac-/lung toxicity as well as secondary malignancies.
Conclusions
The majority of patients in this study benefited from DIBH, however, the impact on the normal tissue dose was highly individual and therefore comparative treatment planning between DIBH and FB is encouraged. The largest benefits were generally observed for the combination of DIBH and IMPT. However, since multiple OARs are considered simultaneously and there is a large variation in disease distribution, there is not a single best treatment technique for all mediastinal HL patients, and the treatment technique should be chosen individually for each patient.
Disclosure statement
No potential conflict of interest was reported by the authors.
References
- Eich HT, Diehl V, Gorgen H, et al. Intensified chemotherapy and dose-reduced involved-field radiotherapy in patients with early unfavorable Hodgkin's lymphoma: final analysis of the German Hodgkin Study Group HD11 trial. JCO. 2010;28:4199–4206.
- Engert A, Plütschow A, Eich HT, et al. Reduced treatment intensity in patients with early-stage Hodgkin’s lymphoma. N Engl J Med. 2010;363:640–652.
- Aleman BM, van den Belt-Dusebout AW, Klokman WJ, et al. Long-term cause-specific mortality of patients treated for Hodgkin's disease. J Clin Oncol. 2003;21:3431–3439.
- Castellino SM, Geiger AM, Mertens AC, et al. Morbidity and mortality in long-term survivors of Hodgkin lymphoma: a report from the Childhood Cancer Survivor Study. Blood. 2011;117:1806–1816.
- Ng A, Bernardo M, Weller E, et al. Long-term survival and competing causes of death in patients with early-stage Hodgkin's disease treated at age 50 or younger. J Clin Oncol. 2002;20:2101–2108.
- Tseng YD, Cutter DJ, Plastaras JP, et al. Evidence-based review on the use of proton therapy in lymphoma from the Particle Therapy Cooperative Group (PTCOG) lymphoma subcommittee. Int J Radiat Oncol Biol Phys. 2017;99:825–842.
- Maraldo MV, Specht L. A decade of comparative dose planning studies for early-stage Hodgkin lymphoma: what can we learn? Int J Radiat Oncol Biol Phys. 2014;90:1126–1135.
- Specht L, Yahalom J, Illidge T, et al. Modern radiation therapy for Hodgkin lymphoma: field and dose guidelines from the international lymphoma radiation oncology group (ILROG). Int J Radiat Oncol Biol Phys. 2014;89:854–862.
- Voong KR, McSpadden K, Pinnix CC, et al. Dosimetric advantages of a "butterfly" technique for intensity-modulated radiation therapy for young female patients with mediastinal Hodgkin's lymphoma. Radiat Oncol. 2014;9:94.
- Lohr F, Georg D, Cozzi L, et al. Novel radiotherapy techniques for involved-field and involved-node treatment of mediastinal Hodgkin lymphoma: when should they be considered and which questions remain open? Strahlenther Onkol. 2014;190:864–871.
- Fiandra C, Filippi AR, Catuzzo P, et al. Different IMRT solutions vs. 3D-conformal radiotherapy in early stage Hodgkin’s lymphoma: dosimetric comparison and clinical considerations. Radiat Oncol. 2012;7:186.
- Stromberg JS, Sharpe MB, Kim LH, et al. Active breathing control (ABC) for Hodgkin's disease: reduction in normal tissue irradiation with deep inspiration and implications for treatment. Int J Radiat Oncol Biol Phys. 2000;48:797–806.
- Paumier A, Ghalibafian M, Gilmore J, et al. Dosimetric benefits of intensity-modulated radiotherapy combined with the deep-inspiration breath-hold technique in patients with mediastinal Hodgkin's lymphoma. Int J Radiat Oncol Biol Phys. 2012;82:1522–1527.
- Petersen PM, Aznar MC, Berthelsen AK, et al. Prospective phase II trial of image-guided radiotherapy in Hodgkin lymphoma: benefit of deep inspiration breath-hold. Acta Oncol. 2015;54:60–66.
- Charpentier AM, Conrad T, Sykes J, et al. Active breathing control for patients receiving mediastinal radiation therapy for lymphoma: impact on normal tissue dose. Pract Radiat Oncol. 2014;4:174–180.
- Aznar MC, Maraldo MV, Schut DA, et al. Minimizing late effects for patients with mediastinal Hodgkin lymphoma: deep inspiration breath-hold, IMRT, or both? Int J Radiat Oncol Biol Phys. 2015;92:169–174.
- Hoppe BS, Flampouri S, Zaiden R, et al. Involved-node proton therapy in combined modality therapy for Hodgkin lymphoma: results of a phase 2 study. Int J Radiat Oncol Biol Phys. 2014;89:1053–1059.
- Chera BS, Rodriguez C, Morris CG, et al. Dosimetric comparison of three different involved nodal irradiation techniques for stage II Hodgkin's lymphoma patients: conventional radiotherapy, intensity-modulated radiotherapy, and three-dimensional proton radiotherapy. Int J Radiat Oncol Biol Phys. 2009;75:1173–1180.
- Li J, Dabaja B, Reed V, et al. Rationale for and preliminary results of proton beam therapy for mediastinal lymphoma. Int J Radiat Oncol Biol Phys. 2011;81:167–174.
- Enmark M, Lundkvist N, Fager M, et al. PTC17-0493: Clinical Commissioning of Gated Proton Pencil Beam Scanning. Additional Proceedings to the 56th Annual Meeting of the Particle Therapy Cooperative Group (PTCOG). Int J Part Ther. 2017;4:39.
- Baues C, Marnitz S, Engert A, et al. Proton versus photon deep inspiration breath hold technique in patients with Hodgkin lymphoma and mediastinal radiation: a planning comparison of deep inspiration breath hold intensity modulation radiotherapy and intensity modulated proton therapy. Radiat Oncol. 2018;13:122.
- Rechner LA, Maraldo MV, Vogelius IR, et al. Life years lost attributable to late effects after radiotherapy for early stage Hodgkin lymphoma: the impact of proton therapy and/or deep inspiration breath hold. Radiother Oncol. 2017;125:41–47.
- Edvardsson A, Nilsson MP, Amptoulach S, et al. Comparison of doses and NTCP to risk organs with enhanced inspiration gating and free breathing for left-sided breast cancer radiotherapy using the AAA algorithm. Radiat Oncol. 2015;10:84.
- Kugele M, Edvardsson A, Berg L, et al. Dosimetric effects of intrafractional isocenter variation during deep inspiration breath-hold for breast cancer patients using surface-guided radiotherapy. J Appl Clin Med Phys. 2018;19:25–38.
- Flejmer AM, Edvardsson A, Dohlmar F, et al. Respiratory gating for proton beam scanning versus photon 3D-CRT for breast cancer radiotherapy. Acta Oncol. 2016;55:577–583.
- Paddick I. A simple scoring ratio to index the conformity of radiosurgical treatment plans. Technical note. J Neurosurg. 2000;93 Suppl.3: 219–222.
- Bert C, Durante M. Motion in radiotherapy: particle therapy. Phys Med Biol. 2011;56:R113–R144.
- Edvardsson A, Nordstrom F, Ceberg C, et al. Motion induced interplay effects for VMAT radiotherapy. Phys Med Biol. 2018;63:085012.
- Liu W, Zhang X, Li Y, et al. Robust optimization of intensity modulated proton therapy. Med Phys. 2012;39:1079–1091.
- Li Y, Niemela P, Liao L, et al. Selective robust optimization: a new intensity-modulated proton therapy optimization strategy. Med Phys. 2015;42:4840–4847.
- Zeng C, Plastaras JP, Tochner ZA, et al. Proton pencil beam scanning for mediastinal lymphoma: the impact of interplay between target motion and beam scanning. Phys Med Biol. 2015;60:3013–3029.
- Enmark M, Olofsson J, Ceberg S, Jonsson J. [P205] The impact on pencil beam scanning (PBS) proton therapy for mediastinal lymphoma from deep inspiation breath-hold (DIBH) variability. Phys Med. 2018;52:159.
- Prescribing, Recording, and Reporting Proton-beam Therapy. ICRU Report 78 2007.
- Paganetti H. Relative biological effectiveness (RBE) values for proton beam therapy. Variations as a function of biological endpoint, dose, and linear energy transfer. Phys Med Biol. 2014;59:R419–R472.