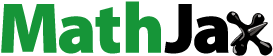
Abstract
Background: For patients with recurrent or second primary disease, re-irradiation can be challenging due to overlap with previously irradiated volumes. Dose painting may be attractive for these patients, as the focus is on delivering maximal dose to areas of high tumor activity. Here, we compare dose painting by contours (DPBC) treatment plans based on 18F-fluorodeoxyglucose (FDG) positron emission tomography (PET) with conventional plans.
Material and methods: We included 10 patients with recurrent or second primary head and neck cancer (HNC) eligible for re-irradiation. Our conventional re-irradiation regimen is hyperfractionated radiotherapy 1.5 Gy twice daily over 4 weeks, giving a total dose of 60 Gy. For DPBC, we defined two prescription volumes, PV33 and PV66, corresponding to 33 and 66% of the highest FDG uptake in the tumor. The clinical target volume (CTV) prescription dose was 60 Gy, PV33; 65–67 Gy and PV66; 70–73 Gy. The DPBC plan is to be given the first 20 fractions and the conventional plan the last 20 fractions. Dose to organs at risk (OARs) were compared for DPBC and conventional treatment. By summation of the initial curative plan and the re-irradiation plan, we also evaluated differences in dose to the 2 ccm hot spot (D2cc).
Results: We achieved DPBC plans with adequate target coverage for all 10 patients. There were no significant differences in OAR doses between the standard plans and the DPBC plans (p=.7). Summation of the initial curative plan and the re-irradiation plan showed that the median D2cc increased from 130 Gy (range 113–132 Gy; conventional) to 140 Gy (range 115–145 Gy; DPBC).
Conclusions: Our proposed DPBC could be straightforwardly implemented and all plans met the objectives. Re-irradiation of HNC with DPBC may increase tumor control without more side effects compared to conventional radiotherapy.
Introduction
Thirty to fifty percent of patients with locally advanced head and neck cancer (HNC) will relapse after their first course of treatment, often including radiotherapy [Citation1]. Many of these relapses are inoperable, or the patient refuses further surgery. The prognosis of patients with loco-regional HNC relapse is poor; <20% live 5 years or more [Citation1]. Second primary HNC also represents a clinical challenge although it has a somewhat better prognosis [Citation2,Citation3]. High dose re-irradiation has traditionally been considered too toxic. Still, modern delivery techniques such as volumetric-modulated arc therapy (VMAT) gives a reduction in the re-irradiated high-dose volume and may give increased overall survival in the order of 30% at the cost of increased long-term morbidity [Citation4].
Studies conducted on local relapses have shown that these are often located within the original gross tumor volume (GTV), and more specifically within the most 18F-FDG avid parts of the tumor as visible on positron emission tomography/computed tomography (PET/CT) [Citation5–7]. The aim of dose painting is to deliver comparatively higher radiation doses to the hypermetabolic part of the tumor with the assumption that high FDG uptake represents the most aggressive phenotype. By increasing the dose to these parts of the tumor, the aim is to increase tumor control without compromising organs at risk (OAR). This would be applicable in both a primary and in a relapse setting. Dose painting may be achieved by two different approaches: (i) dose painting by numbers (DPBN) where each image voxel in the tumor is prescribed a dose based on its PET intensity [Citation8], or (ii) dose painting by contours (DPBC) where the tumor is split into subvolumes according to ranges of PET intensities [Citation8]. DPBC may be accomplished by either (i) prescribing a set dose to each subvolume and aiming for a homogeneous dose delivery (within the subvolume) [Citation9] or (ii) prescribing a minimum dose to each subvolume, allowing for an inhomogeneous dose distribution increasing towards the maximum point prescription dose (in analogy with DPBN).
In this study, the aim was to compare FDG-PET guided DPBC plans using approach (ii) above with a conventional plan for patients referred for re-irradiation of HNC, with emphasis on both target coverage and doses to OAR. To our knowledge, this is the first study investigating re-irradiation of HNC using dose painting.
Material and methods
Patients
The two main inclusion criteria in this treatment planning study were (i) patients with squamous cell carcinoma of the head and neck referred to our center for re-irradiation and (ii) tumor diameter of minimum 2.0 cm. Ten patients were included (); seven males and three females. All had a past history of squamous cell carcinoma of the head or neck and had undergone a previous course of curative radiotherapy with (n = 6) or without (n = 4) concomitant chemotherapy. Six patients had surgery at some point. Seven patients had local relapse; two with second primary cancer, and one with a third primary cancer. The age at the onset of re-irradiation was between 51 and 80 years with a median of 62 years. Except for one patient who received cetuximab, none received concomitant treatment in the re-irradiation regimen.
Table 1. An overview of the patient material.
All patients were planned for both conventional radiotherapy and for dose painting. Patients nos. 1–5 were part of an initial phase of the study to establish the DPBC method. These patients were treated with conventional radiotherapy. Patient nos. 6, 7, 9, and 10 underwent treatment with dose painting, as part of a feasibility study (ClinicalTrials.gov identifier NCT02921581). Patient no. 8 received conventional radiotherapy because of abscess formation in the tumor and surrounding neck areas and concern about excessive toxicity which might have been caused by dose painting. The time between the start of the first course of radiotherapy and the start of re-irradiation ranged from 17 to 153 months with a median of 53 months.
This study was approved by the Regional Ethics Committee (Regional Committees for Medical and Health Research Ethics, South East Norway). Informed consent was obtained from all patients.
Treatment planning
All patients included in this study underwent a treatment planning FDG-PET/CT scan (Biograph 32, Siemens Healthcare, Munich, Germany) from the skull base to mid chest with the arms down. Further details regarding FDG-PET/CT acquisition can be found in a previous publication [Citation10]. Treatment planning was performed using Raystation (v. 4.5/5.0, RaySearch Laboratories, Stockholm, Sweden).
Our conventional re-irradiation regimen consisted of 1.5 Gy twice daily to 60 Gy over 4 weeks. The GTV (defined as the macroscopic disease visible on PET/CT) was expanded by either (i) 5 mm to generate the clinical target volume (CTV, accounting for microscopic disease) or (ii) 10 mm margin in which also limited elective lymph node areas were included. In the latter case, the CTV received a dose of 45 Gy over the first 3 weeks, followed by an additional boost of 15 Gy to the GTV. In the former case, the entire CTV received a dose of 60 Gy.
For dose painting, we adopted a modified version of the methodology of the Ghent group [Citation8] using their linear dose prescription formula:
(1)
(1)
where, Dhigh is the maximum dose and Dlow is the minimum dose, where the latter is the conventional prescription dose of 60 Gy. Furthermore, we set Ihigh=0.95·Imax and Ilow=0.25·Ihigh; where, Imax is the highest PET image value found within the tumor or lymph nodes. We generated two DPBC subvolumes, PV33 and PV66, by applying thresholds relative to the PET intensity distribution. The prescribed doses to these volumes were defined as:
(2)
(2)
(3)
(3)
Substituting DI with D33 (and DI with D66) in EquationEquation (1)(1)
(1) , we obtained threshold intensities defining the PV33 and PV66 volumes:
(4)
(4)
For the first five patients, we used Dhigh=80 Gy, resulting in D33 and D66 of 66.6 Gy and 73.3 Gy, respectively. Due to concerns of prescribing clinically excessive doses, Dhigh was reduced to 75 Gy for the last five patients, resulting in D33 and D66 of 65 Gy and 70 Gy, respectively. The planning goal for the dose painting plans was to achieve a minimum dose to the subvolumes of 100% of the prescription dose, and a minimum of 95% to the conventional target volume. For the conventional CTV (or GTV), a PTV was generated by expanding the CTV (or GTV) by 3 mm with the planning goal of achieving a minimum dose of 95% of the prescription dose to 98% of the volume (D98 ≥ 95%). No PTVs were generated for PV33 and PV66. This treatment was actually delivered to patient nos. 6, 7, 9, and 10.
Our dose painting schedule consisted of 20 fractions of dose painting followed by 20 fractions of conventional treatment. The spatiotemporal stability of FDG-PET images of HNC during radiotherapy is not known. Signs of increased inflammation in and around the tumor on the PET images after 2 weeks of radiotherapy has been reported [Citation11], thus obscuring the true remaining FDG uptake in the tumor cells. We therefore chose to limit dose painting to the first 2 weeks in line with the Ghent approach [Citation11,Citation12]. Our goal was to establish a solution which was clinically feasible. Thus, we decided not to employ imaging during treatment, but instead switch to conventional radiotherapy (without dose painting) after 2 weeks based on the first planning FDG-PET/CT.
All patients were planned for both conventional and DPBC treatment. To ensure no bias towards the DPBC plan, this was optimized with the same objectives for OARs as the conventional plan. The evaluated dose distributions thus consisted of 20 fractions of DPBC plus 20 fractions of the conventional plan versus 40 fractions of the conventional plan.
Five patients were planned with volumetric intensity modulated arc therapy (VMAT) on a Varian TrueBeam STx (Varian Medical Systems, Palo Alto, CA, USA) with a HD120 MLC (2.5 mm and 5 mm central and outer leaf thickness, respectively) and 6 MV photons. The remaining five patients were planned on a Siemens Artiste (Siemens Healthcare, Erlangen, Germany) using mArc, also with 6 MV photons. Dose calculation was done by Raysearch’s implementation of collapsed cone v. 3.0/3.2 with a grid size of 2 mm. All plans were checked for dosimetric accuracy using an Arc Check phantom (Sun Nuclear Corporation, Melbourne, FL, USA). All plans were within the tolerance limit used at our hospital with a gamma pass rate above 95% for the 3%/3 mm criteria, indicating that the DPBC plans could be successfully delivered.
Plan evaluation
To evaluate the differences between DPBC and our standard treatment, we contoured a number of OARs according to guidelines [Citation13–15]. For the organs involved in swallowing and the salivary glands (parotid gland and submandibular gland), mean doses were compared, while for the spinal cord/brain stem and the mandible, the (maximum) D1% doses (dose to 1% of the volume) were compared. Using the Anaconda algorithm in Raystation, the dose distribution from CT basis in the primary treatment was deformed into the CT basis for the DPBC plan. This allowed us to evaluate the accumulated total dose from the two treatment series. In the accumulated plans, we limited the total dose to 50 Gy for the spinal cord, using a repair factor of 30%, requiring at least 1 year between treatments. [Citation16]. The dose to the highest 2 ccm volume (D2cc) as well as 10–50 cc was extracted from the summed plans.
Statistics
Wilcoxon’s matched pairs signed rank sum tests (significance level p<.05) were used when comparing dose volume histogram (DVH) parameters. Cohort-based DVHs were generated by calculating the median volume across all patients at each dose bin. Patient scatter was estimated by first and third quartiles.
Results
demonstrates the difference between a homogenous re-irradiation plan and our DPBC approach for patient no. 4. For DPBC, the three different dose levels assigned to the tumor are clearly visible and closely follow the PET image intensities. Moreover, the summed plan for the primary treatment plus the re-irradiation DPBC plan shows a rather small region receiving doses in the excess of 120 Gy. The median CTV volume (GTV +5 mm margin) was 47.0 ccm (range 15.8–152.9 ccm). The median and range of the planning volumes PV33 and PV 66 were 6.4 cc (1.5–31.9 cc) and 1.8 cc (0.3–9.9 cc), respectively. We achieved adequate target coverage for all 10 patients using DPBC since 95% of the prescription dose covered the CTV, PV33 and PV66. The cohort-based DVHs for the target volumes, the parotid glands and the spinal cord are shown in . As seen for doses to OARs, inter-patient variations are much greater than differences between the DPBC and conventional approach.
Figure 1. (a) Axial image of FDG-PET/CT and corresponding images from the treatment plans for patient no. 4. Top left panel: Pre-treatment PET/CT. Top right panel: Re-irradiation 1.5 Gy × 40, 60 Gy in total without dose painting. Bottom left panel: With dose painting to a maximum point dose of 80 Gy. Bottom right panel: The combined treatment plan of dose painting and the previous radiotherapy after deformable registration. (b) Closer view on the bottom left panel of Figure 1(a). Green color indicates the 57 Gy isodose (95% of 60 Gy) covering the GTV. Orange color: 63.3 Gy isodose (95% of 66.7 Gy) covering PV33. Red color: 69.7 Gy isodose (95% of 73.3 Gy) covering PV66.
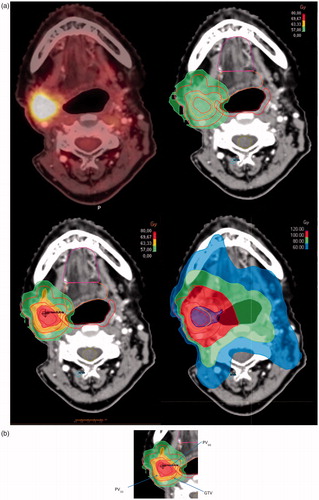
Figure 2. Cohort-based DVHs. Panel 1, CTV 60 Gy; panel 2, PV33; panel 3, PV66; panel 4, spinal cord; panel 5, ipsilateral parotid gland and panel 6, contralateral parotid gland. Median DVHs are solid lines, dashed lines are first and third quartile, black is conventional plan, red is dose painting to 75 Gy (n = 5), and blue is dose painting to 80 Gy (n = 5).
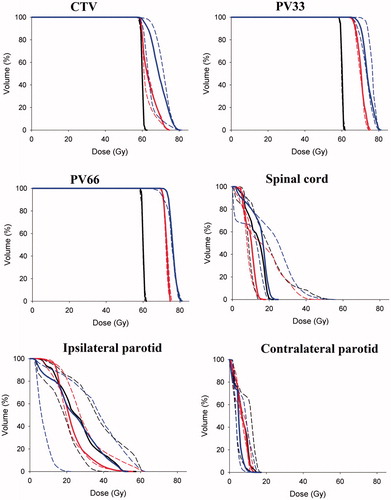
shows the cohort-based median doses to the parotid glands, mandible and spinal cord. For each OAR, there was no significant difference between the conventional and DPBC plan (p>.09). We further compared all pairs (conventional vs dose painting) of the doses to the four OARs (n = 40). Still there was no statistically significant difference (p=.70). shows D2cc from the summation of the first curative plan and the new re-irradiation plan. The cohort-based median D2cc increased from 130 Gy (conventional treatment; range 113–132 Gy) to 140 Gy (DPBC; range 115–145 Gy). Looking at the difference in dose between the DPBC and conventional plan as a function of the corresponding overlap volume, the curves approached zero for volumes in the order of 30–50 ccm ().
Figure 3. Dose to the 2 ccm hot spot volume accounting for both treatments (first radiotherapy and re-irradiation). The two radiotherapy planning CTs have been merged by deformable registration. For patient no. 5, it was impossible to acquire the first treatment plan because of treatment abroad.
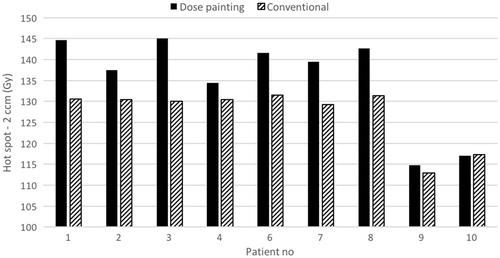
Figure 4. Difference in dose between dose painting re-irradiation and conventional re-irradiation as a function of hot spot volume. The first radiotherapy plan has been included by deformable registration. Dotted lines indicate 75 Gy dose painting plans (instead of 80 Gy).
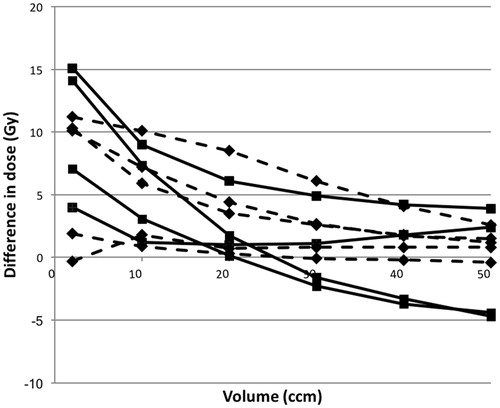
Table 2. The median and range of OAR doses for 10 patients.
Discussion
Dose painting has so far mainly been proposed for patients having curable disease. However, dose painting may also improve the survival for patients with recurrent HNC. To the best of our knowledge, this is the first treatment planning study on dose painting of HNC in need of re-irradiation. We evaluated the dose painting plans by comparing the OAR doses to those of the conventional plans. As found in previous dose painting studies, the OAR doses were not increased [Citation8,Citation16]. Four of our patients included in this study were actually treated using the presented dose painting approach. We will include more patients to further assess feasibility, toxicity and local control in an ongoing study.
A major concern in re-irradiation of HNC is the extent of overlap between the first and second radiotherapy treatment volumes. The dose painting volumes defined in this study were most likely so small that no substantial increase in side effects over that of conventional radiotherapy is expected. However, mucosal ulcers after dose painting have been shown to represent a clinical problem both in the Ghent [Citation12] and Copenhagen study [Citation9]. Thus, there is some concern regarding the dose to the overlap volume and the possible risk of mucosal ulcers. This needs to be taken into careful consideration, possibly with more emphasis on the dose to the mucosa. Other structures such as the carotid artery are also a concern [Citation17]. When evaluating the overlapping volumes for the first five patients, we were worried about the safety of the 80 Gy regimen in terms of late side effects. The regimen was therefore adjusted to a new maximum dose of 75 Gy.
The overlap volume was calculated from the plan summation after deforming the first radiotherapy CT series into the second series. Deformable registration (DR) has its weaknesses [Citation11]. If the difference between the two image sets is too large, the DR algorithm may perform poorly resulting in an inaccurate summation of dose. For example, disappearance of tumor volume after (or during) radiotherapy may introduce errors in the DR results. The alternative is rigid registration, or simply visually comparing the two plans. However, the deformable algorithm was considered to perform well following inspection by a radiation oncologist.
Anatomic changes during a course of radiotherapy may be handled by adaptive radiotherapy, applying image guidance at the treatment unit and replanning if indicated [Citation18]. In dose painting, the changes in functional imaging over time is an additional concern. Significant alterations in the PET uptake distribution will compromise the dose painting plan. In some studies, the same dose painting plan has been delivered over a full schedule of 6–7 weeks [Citation9,Citation16]. In the present study, we chose to limit dose painting to the first 2 weeks in line with the Ghent approach [Citation11,Citation12]. To our knowledge, there is no published data on the spatiotemporal stability of FDG-PET and its implications for dose painting of HNC. There is one study on FDG-PET/CT before radiotherapy of HNC, showing that FDG-PET/CT performed three days apart yields reproducible dose painting plans [Citation19]. Our goal was to establish a solution which was clinically feasible and cost-effective, and thus we chose to switch to conventional radiotherapy (without dose painting) after two weeks based on the first planning FDG-PET/CT. Clinical feasibility was another reason for limiting the imaging modality to FDG-PET/CT, although it may be desirable to incorporate other modalities such as magnetic resonance imaging in the future [Citation20].
No margin was added when generating the PTV for the dose painting subvolumes in the current work. In some studies, the dose painting target is the only boost volume within the conventional GTV and it is in this case straightforward to generate a PTV [Citation9,Citation21,Citation22]. It is difficult to see the PTV concept applied on voxels in DPBN. In the present study, we wished to convert the DPBN Ghent formalism to DPBC omitting the PTV inside the conventional CTV. This was also a pragmatic approach, acknowledging that the true dose painting distribution will be somewhat blurred over a fractionated schedule. Furthermore, the dose painting subvolumes will most often be enclosed by the CTV, ensuring at least the conventional dose to these volumes.
In conclusion, re-irradiation of HNC using dose painting is clinically feasible using already available resources and might offer increased tumor control without additional side effects. Our three-level DPBC could be straightforwardly implemented for all patients and all treatment plans met the objectives. Further studies should be undertaken to determine the actual clinical benefit of dose painting in a re-irradiation setting.
Disclosure statement
No potential conflict of interest was reported by the authors.
Additional information
Funding
References
- Wong SJ, Machtay M, Li Y. Locally recurrent, previously irradiated head and neck cancer: concurrent re-irradiation and chemotherapy, or chemotherapy alone? J Clin Oncol. 2006;24:2653–2658.
- Hoebers F, Heemsbergen W, Moor S, et al. Reirradiation for head-and-neck cancer: delicate balance between effectiveness and toxicity. Int J Radiat Oncol Biol Phys. 2011;81:e111–e118.
- Ho AS, Kraus DH, Ganly I, et al. Decision making in the management of recurrent head and neck cancer. Head Neck. 2014;36:144–151.
- Duprez F, Berwouts D, Madani I, et al. High-dose reirradiation with intensity-modulated radiotherapy for recurrent head-and-neck cancer: disease control, survival and toxicity. Radiother Oncol. 2014;111:388–392.
- Machtay M, Natwa M, Andrel J, et al. Pretreatment FDG-PET standardized uptake value as a prognostic factor for outcome in head and neck cancer. Head Neck. 2009;31:195–201.
- Castaldi P, Rufini V, Bussu F, et al. Can ‘early’ and ‘late’ 18F-FDG PET-CT be used as prognostic factors for the clinical outcome of patients with locally advanced head and neck cancer treated with radio-chemotherapy? Radiother Oncol. 2012;103:63–68.
- Due AK, Vogelius IR, Aznar MC, et al. Recurrences after intensity modulated radiotherapy for head and neck squamous cell carcinoma more likely to originate from regions with high baseline [18F]-FDG uptake. Radiother Oncol. 2014;111:360–365.
- Vanderstraeten B, Duthoy W, De Gersem W, et al. [18F]fluoro-deoxy-glucose positron emission tomography ([18F]FDG-PET) voxel intensity-based intensity-modulated radiation therapy (IMRT) for head and neck cancer. Radiother Oncol. 2006;79:249–258.
- Rasmussen JH, Hakansson K, Vogelius IR, et al. Phase I trial of 18F-Fludeoxyglucose based radiation dose painting with concomitant cisplatin in head and neck cancer. Radiother Oncol. 2016;120:76–80.
- Dale E, Moan JM, Osnes TA, et al. Cervical lymph node metastases of squamous cell carcinoma of unknown origin: the diagnostic value of FDG PET/CT and clinical outcome. Eur Arch Otorhinolaryngol. 2017;274:1015–1019.
- Berwouts D, Olteanu LA, Duprez F, et al. Three-phase adaptive dose-painting-by-numbers for head-and-neck cancer: initial results of the phase I clinical trial. Radiother Oncol. 2013;107:310–316.
- Madani I, Duprez F, Boterberg T, et al. Maximum tolerated dose in a phase I trial on adaptive dose painting by numbers for head and neck cancer. Radiother Oncol. 2011;101:351–355.
- Christianen ME, Langendijk JA, Westerlaan HE, et al. Delineation of organs at risk involved in swallowing for radiotherapy treatment planning. Radiother Oncol. 2011;101:394–402.
- Christianen ME, Schilstra C, Beetz I, et al. Predictive modelling for swallowing dysfunction after primary (chemo)radiation: results of a prospective observational study. Radiother Oncol. 2012;105:107–114.
- Brouwer CL, Steenbakkers RJ, Bourhis J, et al. CT-based delineation of organs at risk in the head and neck region: DAHANCA, EORTC, GORTEC, HKNPCSG, NCIC CTG, NCRI, NRG Oncology and TROG consensus guidelines. Radiother Oncol. 2015;117:83–90.
- Nieder C, Grosu AL, Andratschke NH, et al. Update of human spinal cord reirradiation tolerance based on additional data from 38 patients. Int J Radiat Oncol Biol Phys. 2006;66:1446–1449.
- Yamazaki H, Ogita M, Kodani N, et al. Frequency, outcome and prognostic factors of carotid blowout syndrome after hypofractionated re-irradiation of head and neck cancer using CyberKnife: a multi-institutional study. Radiother Oncol. 2013;107:305–309.
- Schwartz DL. Current progress in adaptive radiation therapy for head and neck cancer. Curr Oncol Rep. 2012;14:139–147.
- Rasmussen JH, Vogelius IR, Aznar MC, et al. Spatio-temporal stability of pre-treatment 18F-Fludeoxyglucose uptake in head and neck squamous cell carcinomas sufficient for dose painting. Acta Oncol. 2015;54:1416–1422.
- Alber M, Thorwarth D. Multi-modality functional image guided dose escalation in the presence of uncertainties. Radiother Oncol. 2014;111:354–359.
- van Elmpt W, De Ruysscher D, van der Salm A, et al. The PET-boost randomised phase II dose-escalation trial in non-small cell lung cancer. Radiother Oncol. 2012;104:67–71.
- Thorwarth D, Eschmann SM, Paulsen F, et al. Hypoxia dose painting by numbers: a planning study. Int J Radiat Oncol Biol Phys. 2007;68:291–300.