Abstract
Introduction: Decreasing the radiation dose in the surgical area is important to lower the risk of wound complications when surgery and radiotherapy are combined for the treatment of spinal metastases. The purpose of this study was to compare the radiation dose in the surgical area for spinal metastases between single fraction external beam radiotherapy (EBRT), single fraction stereotactic body radiotherapy (SBRT) and single fraction SBRT with active sparing (SBRT-AS) of the posterior surgical area.
Methods: Radiotherapy treatment plans for EBRT, SBRT and SBRT-AS of the posterior surgical area were created for 13 patients with spinal metastases. A single fraction of 8Gy was prescribed to the spinal metastasis in the EBRT plan. For the SBRT treatment plans, a single fraction of 18Gy was prescribed to the metastasis and 8Gy to the rest of the vertebral body. For the SBRT plan with active sparing the dose in the designated surgical area was minimized without compromising the dose to the organs at risk.
Results: The median dose in the surgical area was 2.6Gy (1.6–5.3Gy) in the SBRT plan with active sparing of the surgical area compared to a median dose of 3.7Gy (1.6–6.3Gy) in the SBRT plan without sparing and 6.5Gy (3.5–9.1Gy) in the EBRT plans (p < .001). The radiation doses to the spinal metastases and organs at risk were not significantly different between the SBRT plan with and without sparing the surgical area.
Conclusions: The radiation dose to the surgical area is significantly decreased with the use of SBRT compared to EBRT. Active sparing of the surgical area further decreased the mean radiation dose in the surgical area without compromising the dose to the spinal metastasis and the organs at risk.
Introduction
The combination of surgery and radiotherapy has been shown to be effective in achieving pain relief, maintaining ambulatory function and improving quality of life in patients with symptomatic spinal metastases [Citation1–3]. Optimal timing of surgery and radiotherapy is however crucial as radiotherapy may impair wound healing [Citation4,Citation5]. Surgical intervention for spinal metastases is typically followed by conventional 2D external beam radiotherapy (EBRT) for local tumor control and additional pain relief. The dose distribution with EBRT is non-conformal; consequently the radiation dose to the spinal metastasis is limited by the radiation tolerance of the spinal cord and the dose received by the skin and underlying soft tissues is high due to the photon energy attenuation [Citation6]. The administration of post-operative EBRT is therefore deferred 1–2 weeks post-operative to allow for initial wound healing [Citation4].
Advances in radiotherapy techniques have led to the development of conformal radiation techniques such as stereotactic body radiotherapy (SBRT). The conformal dose distribution of SBRT permits dose escalation to ablative radiation doses to the spinal metastasis while limiting the dose to the spinal cord and other surrounding tissues, including the skin [Citation7,Citation8]. Post-operative use of SBRT, rather than EBRT, is therefore likely associated with a lower risk of wound complications [Citation5]. The use of SBRT after surgical stabilization is, however, challenging as the metallic spinal implants cause imaging artifacts and radiation backscattering prevents accurate target delineation, treatment planning and delivery [Citation9]. Reversing the order of surgery and SBRT may overcome some of these technical challenges. Active dose sparing of the surgical area may eliminate the need for the time interval between the two treatment modalities as wound healing is no longer impaired by the damaging effects of high-dose irradiation.
The purpose of this study was to investigate the effect of active sparing of the surgical area with SBRT on the radiation dose in the surgical area as compared to routine single fraction SBRT and single fraction EBRT.
Methods
The imaging studies of thirteen patients treated in a recently conducted phase I/II study (ClinicalTrials.gov identifier: NCT02622841 [A.L. Versteeg et al. 2017, unpublished manuscript]) for symptomatic unstable spinal metastases in the thoracic or lumbar spine requiring surgical stabilization and radiotherapy were included in this study. Patients with symptomatic or radiographic high-grade spinal cord compression (Bilsky 2 or 3 [Citation10]) were not included in this cohort.
Treatment planning
Patients were immobilized in a vacuum mattress (BlueBAG™ Vacuum Cushion, Elekta, Stockholm, Sweden) with arms in abduction above the head. Computed tomography (CT) simulation was performed prior to radiotherapy treatment in radiotherapy position on a Philips large bore CT Scanner (slice thickness 1.2 mm; Philips Medical Systems, Cleveland, OH, USA). In addition, all patients underwent a MRI-scan (1.5 Tesla, slice thickness 1.1–4 mm, Ingenia; Philips Medical System, Best, The Netherlands) also in treatment position. The MRI sequences were registered to the CT by rigid mutual information registration on a box around the tumor. The attending radiation oncologist contoured the gross tumor volume (GTV), the clinical target volume (CTV), the organs at risk (OAR), and the posterior surgical area using in-house delineation software on MRI imaging (T1, T2stir and DWI) [Citation11]. The GTV consisted of the visible volume of the spinal metastasis and the CTV of the vertebra based on the extent the metastasis on pre-operative imaging [Citation12]. The GTV and CTV were expanded with 2 mm to create the planning target volume (PTV), respectively the PTV-boost and the PTV-elective. The posterior surgical area was cranially and caudally bordered by the upper, respectively, lower endplate of the vertebral body adjacent to the planned lower and upper level of pedicle screw instrumentation, laterally by the tips of transverse processes, anteriorly by the vertebral body contour and posteriorly by the outermost skin layer (). Organs at risk (OAR) in close proximity to the tumor were delineated as well. A 2 mm planning OAR volume (PRV) margin was applied to the spinal cord to account for geometrical uncertainties and variations in spinal cord position [Citation13,Citation14].
Figure 1. Different radiotherapy treatment plans for one patient. (A, B) Sagittal and transverse image of conventional EBRT treatment plan. (C, D) Sagittal and transverse image of SBRT treatment plan without sparing of surgical area. (E, F) Sagittal and transverse image of SBRT treatment plan with sparing of the surgical area. The yellow line denotes the surgical area. The dark blue area receives 8 Gy, the turquoise area 9 Gy, the yellow area 16.2 Gy and the orange area 18 Gy.
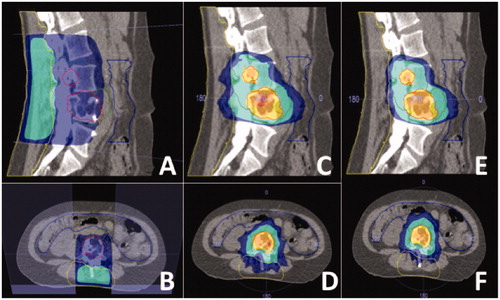
EBRT treatment plans
For EBRT treatment planning, a routine single fraction of 8Gy to the spinal metastasis and the adjacent upper and lower vertebral body was prescribed. A single postero-anterior field with a 100% iso-doseline at 6 cm or 7 cm for a 6 MV or 10 MV, photon beam was planned using the Monaco treatment planning system version 5.1 (Elekta, Stockholm, Sweden). Adequate coverage was achieved if the vertebral body harboring the spinal metastasis received at least 80% of the prescribed dose. An antero-posterior field was added to achieve sufficient coverage, if adequate coverage with a single postero-anterior field alone could not be achieved.
SBRT treatment plan
Volumetric arc therapy (VMAT) treatment plans were generated for all patients in the Monaco treatment planning system version 5.1. The plans were designed for an Elekta Synergy linear accelerator equipped with a 5 mm multi-leaf collimator (Elekta Inc., Crawley, UK). Two 10 MV photon beam posterior partial arcs with an average arc length of 115° were employed. The maximum number of control points per arc was set to 144, the minimum segment width to 0.5 cm, the collimator angle to 0°. In general, VMAT plans were generated using two dual partial arcs starting at a 290° angle (290° to 180°) and at a 70° angle (70° to 180°). The dose calculation grid resolution was 2 × 2 × 2 mm. A simultaneous integrated boost strategy was adopted prescribing 18Gy to the PTV-boost and 8 Gy to the PTV-elective. A single fraction of 18 Gy to the spinal metastasis was prescribed, as studies have shown pain response rates in over 80% of the patients with a dose of 16 Gy or higher [Citation15]. Additionally, 18 Gy single fraction SBRT has been shown to result in less vertebral compression fractures (VCFs) compared with a fraction of 24 Gy (21% vs. 39%, respectively) [Citation16,Citation17]. The PTV-elective was prescribed 8 Gy to treat any bony subclinical disease, which is in line with the dose administered when single fraction EBRT is used to treat spinal metastases in a palliative setting. Adequate coverage of the PTV was defined as at least 90% of the target volume receiving 90% of the prescribed dose. The dose constraints for the OAR were based on local institutional guidelines. The spinal cord tolerance (maximum 12 Gy) was the primary concern during treatment planning. If necessary, a lower dose to the GTV was accepted to meet the dose constraint of the spinal cord.
SBRT treatment plan with active dose sparing of the surgical area
The SBRT plan with sparing of the surgical area was created in the same way as described in the SBRT treatment plan above. VMAT plans were generated using one full double arc instead of the two dual partial arcs. The dose to the surgical area was minimized without compromising the constraints of the OAR and/or target volume coverage.
Statistical analysis
The non-parametric Wilcoxon signed rank test and Friedman test for paired data were used to compare the dosimetric differences between the plans generated for single fraction EBRT, single fraction SBRT and SBRT with dose sparing of the surgical site and. Statistical analyses were performed with RStudio (Version 0.99.903). Significance was defined as p < .05.
Results
The demographic and tumor characteristics of the included patients are displayed in . The majority of the tumors were located in the thoracolumbar junction or lower lumbar area, the median distance of the tumor to the skin was 4.1 cm (1.0–8.1 cm). The dosimetric parameters of the EBRT and SBRT treatment plans are summarized in . The median PTV boost dose for the SBRT plans with and without dose sparing was 17.9 Gy (16.4–18.6 Gy) and 17.5 Gy (16.8–18.3 Gy), respectively. The median percentage of the PTV boost receiving at least 16.2 Gy was similar for the SBRT plans with and without active sparing of the surgical area (83%, range 67–93%; vs. 82%, range 70–96%). The median percentage of the PTV elective receiving at least 7.2 Gy or higher was 62% (25–75%) for the EBRT plan. Median delivery time of the EBRT plan (88 seconds (s), range 84–95) was significantly shorter compared to the SBRT plan with dose sparing (714 s, range 583–1046) and the SBRT plan without dose sparing (815 s, range 516–922) (p < .001). There were no significant differences in the radiation dose to the OAR between the SBRT plan with and without sparing of the surgical area.
Table 1. Patient and tumor characteristics.
Table 2. Dosimetric parameters.
The median radiation dose in the surgical area was significantly lower in the SBRT treatment plan with active sparing of the surgical area (2.6 Gy, range 1.6–5.3 Gy) and the SBRT plan without sparing (3.7 Gy, 1.6–6.3 Gy) compared to the EBRT plan (6.5 Gy, range 3.5–9.1 Gy) (p < .001). The volume of the surgical area receiving 7.2 Gy or higher was 21cc (range 4–148cc) in the SBRT plan with active sparing and 46cc (range 16–138cc) in the SBRT plan without sparing and significantly lower than the 209cc (range 117–568cc) in the EBRT treatment plan (p < .001).
Discussion
This planning study compared single fraction EBRT (8 Gy) to single fraction SBRT (18 Gy) dose distributions, the latter technique with and without active sparing of the posterior surgical area, to explore the feasibility of reducing the radiation dose to the surgical area without compromising the dose to the spinal metastasis and the organs at risk. The use of SBRT compared to EBRT resulted, as expected, in a significant decrease in mean radiation dose to the surgical area. Active sparing of the surgical area with SBRT further decreased the mean dose to this area without compromising the planning quality.
The occurrence of wound complications is one of the primary concerns when radiotherapy and surgery are combined in a short time frame, regardless of the order of execution. Wound complication rates of up to 46% have been reported when EBRT and surgery were combined less than a week apart [Citation4]. Considering the increased application of SBRT as adjuvant radiotherapy treatment Itshayek et al. [Citation5] conducted a systematic review investigating the effect of timing of SBRT and surgical treatment on the risk of wound complications. The authors concluded that due the conformal dose distribution SBRT is likely associated with a lower risk of wound complications [Citation5]. Yet, considering the sensitivity of the early phases of the wound healing process in tissues exposed to radiation, a time interval of at least one week between surgery and SBRT is still recommended [Citation5]. Besides the time interval between surgery and radiotherapy other factors including incision size, radiation dose, number of fractions and patient related factors are important for the risk of wound complications [Citation18]. Kumar et al. [Citation19] investigated the effect of different radiation doses on wound healing in albino mice with surgical wounds. They demonstrated that a dose of 2 Gy to the wound already resulted in delayed healing compared with a wound without exposure to radiation [Citation19]. Wound healing time increased, although non-significantly, with increasing doses of radiation [Citation19]. Other authors also demonstrated a dose-dependent relation between radiation exposure and impaired wound healing with higher radiation doses [Citation20]. In addition, several studies have demonstrated that the mean dose rather than the maximum dose to the surgical area is associated with acute radiation toxicity [Citation21,Citation22]. As the risk of wound complications is multifactorial no definitive radiation dose threshold could be determined for the occurrence wound complications. It is currently unknown whether the additional decrease in mean radiation dose with active sparing of the surgical area as observed in this planning study has a clinically significant impact on the risk of wound complications. The results of the different studies however emphasize the importance of decreasing the radiation dose to a surgical area to minimize impairment of the normal wound healing process.
The use of SBRT for the treatment of spinal metastases is increasing, however the number and quality of studies evaluating the effectiveness of SBRT in the post-operative setting is still limited [Citation23–25]. The first studies show high pain response rates and long-term control but the time interval between surgery and post-operative SBRT remains present [Citation8,Citation23–25]. Post-operative SBRT is not only delayed by the waiting time for wound healing but also by SBRT treatment preparation requirements. The precise delivery of high radiation doses requires up-to-date and accurate MRI imaging, which is challenging in the postoperative setting for the patient and with the spinal implants causing imaging artifacts [Citation8,Citation25]. Additional invasive imaging techniques such as a CT myelogram are therefore often needed to ensure accurate target delineation in treatment planning. Furthermore, the spinal implants cause backscattering of electrons increasing the dose in front of the material while limiting the dose behind the spinal implants [Citation8]. Yazici et al. [Citation26] demonstrated that the dose to the spinal cord increased up to 18.6% when spinal implants were present in the SBRT treatment field. Avoiding interaction between the radiation beam and the spinal implant to prevent high doses to the spinal cord is therefore important but also complicates post-operative SBRT planning [Citation26]. Reversing the order of surgery and SBRT may overcome these technical challenges. The patients included in this study were treated in a phase I/II study investigating the safety of SBRT followed by surgical stabilization within 24 hours for the treatment of unstable spinal metastases (AL Versteeg et al. 2017, unpublished manuscript). A planning strategy with active sparing of the surgical area was used for the administered SBRT treatment. One of the additional inclusion criteria for the phase I/II study was the absence of a history of surgery and/or radiotherapy to the treatment site as these are known risk factors for wound complications. None of the patients experienced disturbed wound healing. Reversing the order of surgery and radiotherapy, and minimizing the radiation dose to the surgical area with SBRT enabled elimination of the traditional required time interval between surgery and radiotherapy.
We acknowledge some limitations of the present study. First, the radiotherapy treatment plans in this study were created by an experienced spine radiotherapy planner potentially limiting the generalizability of the results. Radiotherapy planning is operator dependent and many acceptable-but-different radiotherapy plans can be created for the same patient. Second, to be able to maintain an adequate dose coverage to the metastasis, the radiation dose that is decreased in the surgical area has to be distributed to other areas of the body potentially compromising other OAR. However, in this study the radiation dose to the surgical area could be decreased for all patients without compromising the OAR dose.
Single fraction SBRT was compared with single fraction EBRT instead of multifraction (e.g., 10 × 3 Gy) EBRT as previous studies have shown no difference in pain response between single fraction or multifraction EBRT [Citation27]. Single fraction EBRT has been associated with higher rates of retreatment compared to multifraction EBRT although it has been speculated that this may be attributed to selection bias. Radiation oncologists may be more reluctant to retreat patients with a history of multifraction EBRT considering the total dose to the spinal cord. Furthermore, comparing single fraction SBRT to multifraction EBRT requires complex calculations for a genuine comparison of the dosimetrics. It can be expected that a multifraction EBRT regimen results in even higher mean total radiation doses to the surgical area when compared to a single fraction EBRT technique.
In conclusion, compared to EBRT, use of SBRT significantly decreases the dose to the posterior surgical area thereby likely decreasing the risk of impaired wound healing after surgery. Active sparing of the surgical area further decreases the dose without significantly compromising the dose to the spinal metastasis and organs at risk. Considering the dose-dependent relationship between radiation exposure and impaired wound healing, it may be recommended to actively spare the surgical area in an SBRT plan for patients who recently underwent surgery or are scheduled for surgery as it might be beneficial in reducing the incidence of wound complications. Using SBRT, instead of EBRT, in combination with surgery may enable innovation of treatment strategies.
Disclosure statement
No potential conflict of interest was reported by the authors.
References
- Patchell RA, Tibbs PA, Regine WF, et al. Direct decompressive surgical resection in the treatment of spinal cord compression caused by metastatic cancer: a randomised trial. Lancet. 2005;366:643–648.
- Falicov A, Fisher CG, Sparkes J, et al. Impact of surgical intervention on quality of life in patients with spinal metastases. Spine J. 2006; 31:2849–2856.
- Fehlings MG, Nater A, Tetreault L, et al. Survival and clinical outcomes in surgically treated patients with metastatic epidural spinal cord compression: results of the prospective multicenter AOSpine study. J Clin Oncol. 2016;34:268–276.
- Itshayek E, Yamada J, Bilsky M, et al. Timing of surgery and radiotherapy in the management of metastatic spine disease: a systematic review. Int J Oncol. 2010;36:533–544.
- Itshayek E, Cohen JE, Yamada Y, et al. Timing of stereotactic radiosurgery and surgery and wound healing in patients with spinal tumors: a systematic review and expert opinions. Neurol Res. 2014;36:510–523.
- Verlaan J-J, Westhoff PG, Hes J, et al. Sparing the posterior surgical site when planning radiation therapy for thoracic metastatic spinal disease. Spine J. 2012;12:324–328.
- Hamilton AJ, Lulu BA, Fosmire H, et al. Preliminary clinical experience with linear accelerator-based spinal stereotactic radiosurgery. Neurosurgery. 1995;36:311–319.
- Redmond KJ, Lo SS, Fisher C, et al. Postoperative stereotactic body radiation therapy (SBRT) for spine metastases: a critical review to guide practice. Int J Radiat Oncol Biol Phys. 2016;95:1414–1428.
- Wang X, Yang JN, Li X, et al. Effect of spine hardware on small spinal stereotactic radiosurgery dosimetry. Phys Med Biol. 2013;58:6733–6747.
- Bilsky MH, Laufer I, Fourney DR, et al. Reliability analysis of the epidural spinal cord compression scale. J Neurosurg Spine. 2010;13:324–328.
- Bol GH, Kotte AN, van der Heide UA, et al. Simultaneous multi-modality ROI delineation in clinical practice. Comput Methods Programs Biomed. 2009;96:133–140.
- Cox BW, Spratt DE, Lovelock M, et al. International spine radiosurgery consortium consensus guidelines for target volume definition in spinal stereotactic radiosurgery. Int J Radiat Oncol Biol Phys. 2012;83:e597–e605.
- Cai J, Sheng K, Sheehan JP, et al. Evaluation of thoracic spinal cord motion using dynamic MRI. Radiother Oncol. 2007;84:279–282.
- Tseng C-L, Sussman MS, Atenafu EG, et al. Magnetic resonance imaging assessment of spinal cord and cauda equina motion in supine patients with spinal metastases planned for spine stereotactic body radiation therapy. Int J Radiat Oncol Biol Phys. 2015;1;91:995–1002.
- Ryu S, Jin R, Jin J-Y, et al. Pain control by image-guided radiosurgery for solitary spinal metastasis. J Pain Symptom Manage. 2008;35:292–298.
- Rose PS, Laufer I, Boland PJ, et al. Risk of fracture after single fraction image-guided intensity-modulated radiation therapy to spinal metastases. J Clin Oncol. 2009;27:5075–5079.
- Germano IM, Carai A, Pawha P, et al. Clinical outcome of vertebral compression fracture after single fraction spine radiosurgery for spinal metastases. Clin Exp Metastasis. 2016;33:143–149.
- Stewart FA, Akleyev AV, Hauer-Jensen M, et al. ICRP publication 118: ICRP statement on tissue reactions and early and late effects of radiation in normal tissues and organs-threshold doses for tissue reactions in a radiation protection context. Ann ICRP. 2012;41:1–322.
- Kumar P, Jagetia GC. Modulation of wound healing in Swiss albino mice by different doses of gamma radiation. Burns. 1995;21:163–165.
- Balter S, Hopewell JW, Miller DL, et al. Fluoroscopically guided interventional procedures: a review of radiation effects on patients' skin and hair. Radiology. 2010;254:326–341.
- Gokhale AS, Beriwal S, Smith RP, et al. Clinical and dosimetric factors associated with acute rectal toxicity in patients treated with (131) cs brachytherapy for prostate cancer. Brachytherapy. 2010;9:328–334.
- Gokhale AS, McLaughlin BT, Flickinger JC, et al. Clinical and dosimetric factors associated with a prolonged feeding tube requirement in patients treated with chemoradiotherapy (CRT) for head and neck cancers. Ann Oncol. 2010;21:145–151.
- Gerszten PC, Germanwala A, Burton SA, et al. Combination kyphoplasty and spinal radiosurgery: a new treatment paradigm for pathological fractures. J Neurosurg Spine. 2005;3:296–301.
- Gerszten PC, Monaco EAIII. Complete percutaneous treatment of vertebral body tumors causing spinal canal compromise using a transpedicular cavitation, cement augmentation, and radiosurgical technique. Neurosurg Focus. 2009;27:E9.
- Massicotte E, Foote M, Reddy R, et al. Minimal access spine surgery (MASS) for decompression and stabilization performed as an out-patient procedure for metastatic spinal tumours followed by spine stereotactic body radiotherapy (SBRT): first report of technique and preliminary outcomes. Technol Cancer Res Treat. 2012;11:15–25.
- Yazici G, Sari SY, Yedekci FY, et al. The dosimetric impact of implants on thespinal cord dose during stereotactic bodyradiotherapy. Radiat Oncol. 2016;11:71.
- Chow E, Zeng L, Salvo N, et al. Update on the systematic review of palliative radiotherapy trials for bone metastases. Clin Oncol (R Coll Radiol). 2012;24:112–124.