Abstract
Purpose: To compare early and late toxicities, dosimetric parameters and quality of life (QoL) between conventionally fractionated proton beam therapy (PBT) and intensity-modulated radiation therapy (IMRT) in prostate cancer (PCA) patients.
Methods: Eighty-eight patients with localized PCA treated between 2013 and 2017 with either definitive PBT (31) or IMRT (57) were matched using propensity score matching on PCA risk group, transurethral resection of the prostate, prostate volume, diabetes mellitus and administration of anticoagulants resulting in 29 matched pairs. Early and late genitourinary (GU) and gastrointestinal (GI) toxicities according to Common Terminology Criteria for Adverse Events (CTCAE) and QoL based on EORTC-QLQ-C30/PR25 questionnaires were collected prospectively until 12 months after radiotherapy (RT). Associations between toxicities and dose–volume parameters in corresponding organs at risk (OARs) were modeled by logistic regression.
Results: There were no significant differences in GI and GU toxicities between both treatment groups except for late urinary urgency, which was significantly lower after PBT (IMRT: 25.0%, PBT: 0%, p = .047). Late GU toxicities and obstruction grade ≥2 were significantly associated with the relative volume of the anterior bladder wall receiving 70 Gy and the entire bladder receiving 60 Gy, respectively. The majority of patients in both groups reported high functioning and low symptom scores for the QoL questionnaires before and after RT. No or little changes were observed for most items between baseline and 3 or 12 months after RT, respectively. Global health status increased more at 12 months after IMRT (p = 0.079) compared to PBT, while the change of constipation was better at 3 months after PBT compared to IMRT (p = 0.068).
Conclusions: Overall, IMRT and PBT were well tolerated. Despite the superiority of PBT in early constipation and IMRT in late global health status compared to baseline, overall QoL and the risks of early and late GU and GI toxicities were similar for conventionally fractionated IMRT and PBT.
Introduction
Definitive radiotherapy (RT) with or without androgen deprivation therapy is a widely used treatment modality in localized or locally advanced prostate cancer (PCA) [Citation1]. RT with intensity-modulated radiotherapy (IMRT) achieves a precise dose delivery and allows for dose escalation while sparing normal tissue from high doses [Citation2–4]. Proton beam therapy (PBT) aims to further improve the sparing of surrounding healthy tissue by decreasing the volume irradiated with low to medium doses, which may reduce the risk of secondary malignant tumors and of radiation-induced toxicities [Citation5–9]. However, there are still controversies regarding the toxicity risk of each technique in PCA patients. Conventionally fractionated IMRT studies reported late grade ≥2 GI and GU toxicity of approximately 2 and 12%, respectively [Citation10]. In the conventionally fractionated arm of the CHHiP trial, the cumulative 5-year incidence of grade ≥2 GI and GU toxicity was estimated at 14% and 9%, respectively [Citation11]. In large series of conventionally fractionated PBT studies, late GI and GU toxicities of grade ≥2 were reported to be around 4% and 5%, respectively [Citation12–15]. Sheets et al. [Citation16] described lower rates of associated GI side effects after IMRT compared with PBT, while Yu et al. [Citation17] showed no difference in early toxicities between both treatment modalities. A recent case-matched study on GI and GU toxicity outcomes after PBT and IMRT demonstrated no significant difference after adjustment for confounders and predictive factors on prospectively collected data, even though no quality of life (QoL) analysis was conducted [Citation18]. There are still very few reports regarding QoL of patients with PCA that received PBT [Citation16,Citation19,Citation20]. No difference in QoL scores between patients treated with PBT or IMRT could be demonstrated so far [Citation16,Citation19]. In this study, a matched-pair analysis was performed in PCA patients treated with conventionally fractionated PBT or IMRT. Early and late GI and GU toxicity rates and their relationship to dosimetric parameters in organs at risk (OARs) were analyzed. In addition, the difference in QoL between these two patient groups was evaluated.
Patients and methods
Patient characteristics
A total of 88 consecutive patients treated with definitive intent at the Department of Radiotherapy and Radiation Oncology of the University Hospital Carl Gustav Carus Dresden were included in this comparative study ( for post-matching and Supplementary Table S1 for pre-matching cohort). Thirty-one patients received conventionally fractionated PBT between January 2015 and March 2017 within the prospective study Proto-R-Prostata (DRKS00008754), and 57 patients were treated with conventionally fractionated IMRT between May 2013 and December 2016. All patients met the following criteria: age over 18 years, histologically confirmed localized or locally advanced PCA without positive pelvic lymph nodes or distant metastases, and an Eastern Cooperative Oncology Group (ECOG) status ≤2. Written informed consent was obtained from patients. This retrospective analysis was approved by the local Ethics Committee (EK426102017).
Table 1. Clinical parameters of the patients included in the matched-pair analysis.
Radiation treatment planning and delivery
For delineation and treatment planning, a computed tomography (CT) scan with 2 mm slice thickness was acquired for each patient. Prostate, seminal vesicles and the OARs bladder, rectum, sigmoid, penile bulb and femoral heads were delineated by an expert radiation oncologist. To evaluate the relation between side effects and dose to OARs, the bladder wall (3 mm), anterior and posterior bladder walls as well as the rectal wall (3 mm) were retrospectively contoured by one observer (LA).
All patients underwent simulation and treatment with an endo-rectal balloon to spare the rectum and to stabilize prostate position. For the IMRT group, the balloon (Additec GmbH, Markt Indersdorf, Germany) was filled with 80 ml air, while for the PBT group the balloon (MEDRad® Pro-Tekt, Bayer Medical Care Inc., Wilmington, DE, USA) was filled with 120 ml water as recommended by the manufacturers. All patients participated in a bladder filling protocol to achieve an approximately equal bladder filling of 150–350 ml at each fraction [Citation21].
For IMRT patients, the clinical target volume 2 (CTV2) included the prostate with an isotropic margin of 4 mm, excluding the rectal wall. The CTV1 included the prostate (CTV2) plus 2 cm of the proximal seminal vesicles and an additional 4 mm margin in all directions. The planning target volume 2 (PTV2) encompassed the CTV2 with an isotropic 4 mm margin, and the PTV1 included the CTV1 plus a 5 mm posterior margin and an 8 mm margin in all other directions. The total prescribed dose for the PTV2 was 74–78 Gy delivered in 37–39 fractions of 2 Gy daily. The prescribed dose for PTV1 was 58 Gy delivered in 29 fractions of 2 Gy each.
IMRT plans were created with the treatment planning system Pinnacle³ 9.8 (Philips Radiation Oncology Systems, Fitchburg, WI, USA). RT was delivered using high-energy X-rays (15 MV) through a Siemens linear accelerator (ARTISTE™ Solution from Siemens® Medical Solutions, Malvern, PA, USA). The daily patient positioning was based on the bony anatomy in the target region using X-ray imaging (ExacTrac® X-Ray 6D, Brainlab AG, Feldkirchen, Germany) and fusion to three intraprostatic fiducial goldmarkers (Additec GmbH, Markt Indersdorf, Germany).
For PBT patients, the internal clinical target volume 2 (iCTV2) included the prostate only with an isotropic margin of 4 mm excluding the rectal wall. The iCTV1 included the prostate (iCTV2) plus 2 cm of the proximal seminal vesicles and an additional isotropic margin of 4 mm. The relative biological effectiveness (RBE) was considered to be constant at 1.1. The total RBE-corrected prescribed dose for the iCTV2 was 74–76 Gy in 37–38 fractions of 2 Gy daily. The prescribed dose for iCTV1 (prostate and seminal vesicles) was 58 Gy in 29 fractions of 2 Gy each. PBT was planned with XiO® 5.00 (Impac Medical Systems Inc., Maryland Heights, MO, USA). A passive scatter technique consisting of 2-parallel opposed lateral fields was used to deliver the PBT. The daily patient positioning was based on the bony anatomy in the target region and three intraprostatic fiducial markers (PolyMark™, CIVCO Medical Solutions, Kalona, IA, USA) within a 3 mm margin using X-ray imaging. Positioning was verified with an in-room CT scan on a weekly basis.
Endpoint definition and extraction of dose–volume parameters
Treatment-related GU and GI side effects were prospectively scored at baseline, weekly during RT, at the end of RT and at 3–6-month intervals after RT using the Common Terminology Criteria for Adverse Events (CTCAE) version 4 scoring system (U.S. Department of Health and Human Services, National Institutes of Health, National Cancer Institute, Washington, D.C., USA). The following GU side effects were investigated: non-infective cystitis, hematuria, obstruction, urinary incontinence, urinary urgency, urinary frequency and pain. Diarrhea, fecal incontinence, proctitis and rectal bleeding were GI endpoints. Additionally, the maximum severity scores of both GU and GI early (up to 3 months after RT) and late (at 12 months after RT) side effects were analyzed. For patients with a non-zero baseline value and an increase in severity, the maximum score was used irrespective of the pretreatment value. Severity score 0 was assigned to patients without an increase in severity compared to pretreatment. Endpoints were dichotomized at grade ≥1 (grade 0 vs remaining) and grade ≥2 (grade 0 and 1 vs remaining) according to the incidence rates of each side effect. QoL was prospectively assessed using the European Organization for Research and Treatment of Cancer (EORTC) QLQ-C30 version 3.0 questionnaires and the prostate-cancer specific module QLQ-PR25 at baseline, at the end of RT as well as 3 and 12 months after RT (see Supplementary Table S2 for the specific items) [Citation22,Citation23]. The dose distributions for the RT plans of all patients after the matching process were retrospectively exported using RayStation® version 6.0 scripts (RaySearch Laboratories AB, Stockholm, Sweden). Relative volume parameters VxGy (relative volume of an OAR receiving x Gy [in %]) ranging from 40 to 70 Gy in increments of 10 Gy, mean dose (Dmean) as well as V75Gy and the dose parameter D2% (dose in 2% of the OAR volume [in Gy]), representing near maximum doses, were analyzed. These seven dose–volume parameters were extracted for all contoured OARs. To compensate for the different prescribed doses, all plans were retrospectively rescaled to 74 Gy and the corresponding dose–volume parameters were additionally extracted.
Matched-pair analysis
To investigate differences in the incidence frequencies of early and late GU and GI side effects as well as QoL between comparable patient groups receiving PBT or IMRT, a matched-pair analysis was conducted. The following variables, which are potential confounders for the occurrence of toxicities, were used for matching: risk group according to the D’Amico score, transurethral resection of the prostate (TUR-P), prostate volume (binarized on median value), diabetes mellitus, and administration of anticoagulants. During propensity score matching (one-to-one without replacement) with in-house written Python version 2.7.10 software (Python Software Foundation, Wilmington, DE, USA) using the module scipy (stats) 29 pairs were identified (15 exact matches). The maximum allowed difference in propensity scores (caliber) was defined as 0.2 times the standard deviation of the logit of the propensity score [Citation24]. The toxicities had no influence on the matching process. The matched patient groups did not significantly differ in the matching variables (Supplementary Table S1). Nevertheless, patients in the PBT group were significantly younger and received a significantly lower prescribed dose compare to those receiving IMRT ().
Statistical analysis
To examine differences in continuous variables between patient groups, the nonparametric Mann–Whitney U test was conducted. Differences in categorical variables and severity grades of the different side effects were investigated by the χ2 test and the Fisher exact test. Only matched pairs with data available for both patients were included in the analyses. To identify relations between side effects and dose–volume parameters of associated OARs univariable logistic regression was applied. Parameters showing a significant association to the investigated endpoint and the largest area under the receiver operating characteristic curve (AUC) were selected.
QoL was evaluated according to the EORTC QLQ-C30 scoring guidelines [Citation25]. Each item is scored on a linear scale ranging from 0 to 100. On functional scales, high scores represent a healthy level of functioning or global health status. High scores on symptom scales represent a high level of symptomatology. To assess patient individual changes in QoL scores over time (baseline vs 3 months and 12 months after RT, respectively) the nonparametric Wilcoxon signed-rank test was performed. Differences in QoL scores in the IMRT and in the PBT group at specific time points were investigated by the Mann–Whitney U test. The clinical relevance of changes was classified as suggested by Osoba et al. [Citation26] into ‘no change’, ‘a little’, ‘moderate’ and ‘very much’ for absolute differences in QoL scores of 0–4.9, 5.0–9.9, 10.0–19.9 and ≥20.0, respectively. All analyses were performed with two-sided tests by SPSS 25 software (IBM Corporation, Armonk, NY, USA). p-values <.05 were considered statistically significant.
Results
Incidence rates of side effects are summarized in and . Most patients developed early grade ≥1 GU side effects in both treatment groups (IMRT: 89.7% vs PBT: 93.1%). Urinary urgency was the most common symptom (86.2% in both groups), followed by an increased urinary frequency (IMRT: 79.4%, PBT: 75.9%). Only one patient in each treatment group developed grade 3 GU toxicities. Early grade ≥1 GI toxicities occurred less frequently in both groups (IMRT: 55.2%, PBT: 65.5%). The most common side effects were proctitis (IMRT: 37.9% vs PBT: 48.3%) and diarrhea (IMRT: 41.4% vs PBT: 44.8%). Only one patient in the PBT group developed grade 3 diarrhea. The PBT group reported lower GU toxicities at 12 months after RT compared to the IMRT group (IMRT: 63.3% vs PBT: 54.4%). Urinary frequency and obstruction were the most common late GU symptoms (IMRT: 36.4%, PBT: 31.8% and IMRT: 42.9%, PBT: 23.8%, respectively). Grade 1 urinary urgency was significantly lower in the PBT group (IMRT: 25.0% vs PBT: 0%, p = .047), with no higher grade urinary urgency observed. One patient in the IMRT group developed grade 3 obstruction. The incidence of late GI toxicity was low in both groups (IMRT: 36.4%, PBT: 22.7%). Grade 1 proctitis was the most common toxicity (IMRT: 22.7%, PBT: 4.5%), followed by grade 1 rectal bleeding (IMRT: 13.6%, PBT: 4.5%). In one patient in the PBT group rectal bleeding grade 3 was documented. There were no significant differences in GI toxicities between both treatment groups. Details on patients presented with grade 3 toxicities are reported in Supplementary Table S3.
Figure 1. Overall genitourinary (GU) and gastrointestinal (GI) toxicities up to 3 months after radiotherapy (early) and at 12 months after RT (late). IMRT: intensity-modulated radiotherapy; PBT: proton beam therapy.
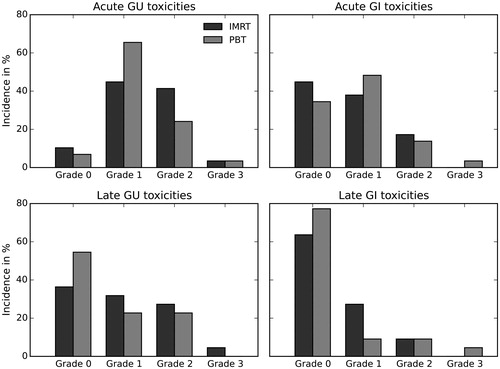
Table 2. Early and late (12 months after radiotherapy) genitourinary (GU) and gastrointestinal (GI) side effects according to CTCAE version 4.0.
In the dosimetric comparison, all dose–volume parameters of the investigated OARs were significantly lower in the PBT group compared to the IMRT group (Supplementary Table S4). For the plans rescaled to 74 Gy, dose–volume parameters of intermediate doses were significantly lower for PBT compared to IMRT. The high dose parameters D2% and V75Gy in bladder, bladder wall and posterior bladder wall did not differ significantly between both groups. These two parameters were significantly higher in the rectum for PBT compared to IMRT (Supplementary Table S5). To determine specific dose–volume parameters related to side effects, logistic regressions were conducted. Parameters with a significant impact under PBT were also tested for the IMRT cohort to evaluate whether these parameters are of importance for both treatment modalities (). Late grade ≥2 GU toxicity was significantly associated with the dose–volume parameter V70Gy of the anterior bladder wall (p = .031) for the PBT patients, while this was not the case for the IMRT patients (p = .13). Late grade ≥2 obstruction was significantly associated with the dose–volume parameter V60Gy of the entire bladder for both cohorts (PBT: p = .047, IMRT: p = .043). In ) the prediction of the corresponding logistic regression models (lines) and the observed outcome data (points) are presented. The data points reflect the mean incidence rates of the side effect and the mean values of the corresponding dose–volume parameter of equally sized patient groups of both treatments. Late grade ≥2 GU toxicities were significantly associated with the dose–volume parameter V70Gy of the anterior bladder wall (p = .031) while this was not the case for the IMRT patients (p = .13). Late grade ≥2 obstruction was significantly associated with the dose–volume parameter V60 Gy of the entire bladder (p = .047). The latter was also the case for IMRT patients (p = .043).
Figure 2. Logistic regression models for late genitourinary (GU) symptoms grade ≥2 as a function of the relative volume of the anterior bladder wall receiving at least 70 Gy (a) and late obstruction grade ≥2 as a function of the relative volume of the bladder receiving at least 60 Gy (b) based on proton beam therapy (PBT, solid lines) and intensity-modulated radiotherapy (IMRT, dashed lines). Data points represent mean incidence rates and mean values of the corresponding dose–volume parameter of equally sized patient groups, standard deviations are visualized by bars.
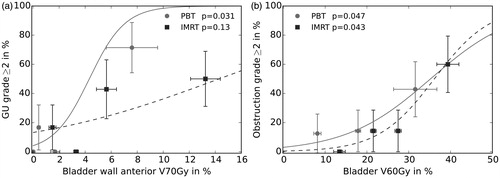
Table 3. Logistic regression of late (12 months after radiotherapy) genitourinary symptoms and dose–volume parameters of the entire bladder wall and anterior bladder wall.
The QoL scores at different time points for both modules QLQ-C30 and QLQ-PR25 and both treatment groups are reported in Supplementary Tables S6–S8 and Figures S1–S3. The majority of patients in both groups reported high functioning scores (>60) and relatively low symptom scores (<35) for the QLQ-C30 questionnaire and low symptom scores for the QLQ-PR25 module before and after RT. The response rates for the functional scales of EORTC QLQ-PR25 were low (sexual activity: 28–62%, sexual functioning: 7–14%) and thus, did not allow for analysis (Supplementary Table S2). Patient individual differences in the QoL scores between baseline and 3 or 12 months after RT, respectively, are presented in and Supplementary Figures S4–S6. For both time points and patient groups no or little changes were observed for the majority of QLQ-C30 items as well as for the QLQ-PR25 symptom scale. At 3 months after RT compared to baseline, constipation was worse for the IMRT group compared to PBT group (mean difference IMRT: 6.7, PBT: −6.7, p=.068). The change in global health status between 12 months after RT and baseline was higher in the IMRT group compared to the PBT group (mean difference IMRT: 8.3, PBT: −2.8, p = .079). On the QLQ-PR25 symptom scale, for the IMRT group, a moderate increase of urinary symptoms 3 months as well as 12 months after RT compared to baseline was reported (p = .012 and p = .036, respectively). The bowel symptoms and incontinence aids increased 12 months after PBT, albeit not statistically significant.
Table 4. Quality of life score differences for the EORTC QLQ-C30 and EORTC QLQ-PR25 questionnaires 3 months after RT vs baseline and 12 months after RT vs baseline for the IMRT and PBT group.
Discussion
Overall, IMRT and PBT were well tolerated. The PBT patients tolerated RT somewhat better than the IMRT patients in the long-term follow up, but the difference in the incidence rates of both groups were not statistically significant, except for late urinary urgency. The majority of patients in both groups reported high functioning scores for the QLQ-C30 questionnaire and low symptom scores for the QLQ-C30 and QLQ-PR25 modules before and after RT.
The incidence rates of early and late side effects in both groups are in accordance to the literature [Citation12,Citation14,Citation16–18,Citation27–29]. Yu et al. [Citation17] compared early-stage PCA patients treated with PBT or IMRT. One year post-treatment no difference could be observed in GU (PBT: 18.8% vs IMRT: 17.5%) and GI toxicity (PBT: 9.9% vs IMRT: 10.2%). Except for the higher incidence rate of grade ≥2 GU toxicities following IMRT, the toxicity rates one year after treatment are comparable in this study and no differences could be seen in overall GU (IMRT: 31.8%, PBT: 22.7%) and GI toxicity (PBT: 13.6% vs IMRT: 9.1%). Sheets et al. [Citation16] conducted a matching analysis of non-metastatic PCA patients between IMRT and PBT. Late GI toxicity rates were lower in IMRT patients (12.2 vs 17.8 per 100 person-years), but there was no significant difference in GU toxicity rates between IMRT and PBT. A lower incidence rate of GI toxicity one year after IMRT compared to PBT can be confirmed in this study (9.1 vs 13.6 per 100 person-years) but the difference was statistically not significant. Recently, Pan et al. [Citation29] compared 572 patients treated with PBT to 2862 patients treated with IMRT in a retrospective score-matched study on younger PCA patients. One year after RT, PBT patients showed significantly lower composite urinary toxicity than IMRT patients RT (23.1% vs 31.6%), but the incidence rate of bowel symptoms was similar (7.7% vs 7.4%). The GU incidence rates are highly consistent with this study (PBT: 22.7%, IMRT: 31.8%). The GI side effects occurred more frequently in both treatment groups of this study compared to the study of Pan et al. especially following PBT (PBT: 13.6% vs IMRT: 9.1%). Nevertheless, these values are highly consistent with GI incidence rates (PBT: 12.8% vs IMRT: 10.8%) in a case-matching study by Fang et al. [Citation18] investigating patients with localized PCA treated with PBT or IMRT to 79.2 Gy (94 patients each). No significant differences in either risks of early or late GI and GU toxicities could be found after adjustment for confounders and predictive factors in this study.
All dose–volume parameters of the rectum and bladder were lower for PBT compared to IMRT. These dosimetric differences may be explained by the different dose–volume characteristics of both treatment modalities but may also result from the slightly higher prescribed doses in the IMRT group than in the PBT group (difference of the mean dose prescriptions about 2.8 Gy; IMRT: 77.4 Gy, PBT: 74.6 Gy). To adjust for the different dose prescriptions, a retrospective rescaling of all plans to 74 Gy was conducted. With this rescaling, the intermediate dose parameters were still significantly smaller for PBT compared to IMRT for all investigated OARs. However, after rescaling, high dose parameters were comparable between both modalities for the bladder, while V75Gy and D2% were even significantly higher in PBT for the rectum. The box-shaped irradiation fields of the proton scattering technique are less conformal than the IMRT dose distributions leading to high doses in adjacent OARs. The more modern PBT technique pencil beam scanning may achieve more conformal dose distributions. Nevertheless, dose distributions of rescaled plans may differ from entirely new treatment plans as they would be created using corresponding planning objectives. In the above-mentioned study by Fang et al. [Citation18] the dosimetric parameters of bladder and rectum were significantly lower for PBT compared to IMRT with endo-rectal balloons for both cohorts. However, different dosimetric comparison studies between PBT and IMRT draw different conclusions. PBT seems to either decrease high doses to the rectum [Citation8] or yield similar rectal dosimetric parameters [Citation7]. Another study showed increased high-dose exposure to the rectum, but decreased low-doses to the bladder [Citation30]. The studies did not report on the use of bladder filling protocols. In this study, late GU toxicity and obstruction were associated with high proton doses of the anterior bladder wall and the bladder, respectively. Makishima et al. [Citation31] revealed higher values for the parameters V60Gy–V70Gy of the bladder in patients with late grade 2 GU toxicity following PBT compared to patients with grade <2 toxicity, but the differences were not significant. The majority of studies found no bladder dose–volume relationship to late GU toxicity following RT using photons for prostate-cancer [Citation32]. Thus, Viswanathan et al. [Citation32] refer clinicians to the QUANTEC data [Citation33] based on the Radiation Therapy Oncology Group (RTOG) 0415 study of PCA [Citation34] to limit high doses >65 Gy to the bladder. These normal-tissue tolerance guidelines for treatment planning in PBT are based on patient data after three-dimensional conformal RT (3D-CRT) [Citation32,Citation35]. Due to the steeper dose gradient in PBT compared to 3D-CRT, other dose–volume parameters or only parts of the OARs, such as anterior or posterior bladder wall, may be more important in predicting toxicities than those of the entire bladder (). These findings could help physicians to better select patients that can benefit from PBT with less toxicity according the favorable patients’ anatomy.
QoL was reported in both cohorts comparable to other PCA patients described in the literature using the same scoring questionnaires. Examples include baseline QoL scores from the EORTC QLQ-C30 Reference Values Manual [Citation36] for different patient cohorts including 3361 PCA patients of all stages, QLQ-C30 and QLQ-PR25 scores evaluated by Cuypers et al. [Citation37] pre- and post-diagnosis for patients treated radically with RT or prostatectomy and scores from Lips et al. [Citation38] comparing 92 patients treated with 76 Gy IMRT to 78 patients treated with 3D-CRT. In the study of Lips et al. the global health status was moderately higher, but they investigated younger patients. Patients were also asked for QoL 6 months after IMRT and reported little or moderately lower symptoms and higher functioning compared to the patients of the present study 12 months after IMRT. The PBT group, however, reported QoL with no or little differences to patients investigated by Lips et al. Late QoL more than 10 years after prostatectomy followed by adjuvant RT or ‘wait and see’ was investigated by van Leeuwen et al. [Citation39] in a cross-sectional and cross-country study. Drummond et al. [Citation40] reported QoL according to QLQ-C30 and QLQ-PR25 2–18 years post-diagnosis in a cross-sectional study including 1127 PCA survivors treated with external beam RT only. In this study, the QoL scores 12 months after IMRT were mainly little or moderately worse compared to the mentioned studies and almost no differences were seen in the PBT group on the QLQ-C30 scale. Some studies assessed QoL after PBT using other scoring systems, the Expanded Prostate Cancer Index Composite (EPIC) as well as, the International Prostate Symptom Score (IPSS) and the Prostate Cancer Symptom Indices (PCSI). Gray et al. [Citation20] compared the mean QoL changes from baseline at 3 and 12 months between PBT and IMRT, defined as a mean change score exceeding half the standard deviation of the baseline value. At 3 months, clinically meaningful changes in all domains of urinary irradiation, incontinence and bowel QoL were discovered for patients treated with IMRT, but not for those treated with PBT. Based on the same definition, this study reported a comparable number of clinically meaningful decrements. In addition, a clinically meaningful decrement in bowel QoL was observed in patients who received PBT. At 12 months, all cohorts in this study and in the study by Gray et al. reported clinically meaningful decrements in bowel QoL. This was also seen in the urinary irradiation domain, except for the PBT cohort in the study by Gray et al. (see Supplementary Table S9). Mendenhall et al. [Citation14] assessed QoL at >4 years after PBT of 78 Gy in 211 PCA patients. Bryant et al. [Citation12] and Henderson et al. [Citation41] reported QoL data 5 years after PBT treatment. In all of the three studies, the IPSS or EPIC summary scores for bowel, urinary irritative/obstructive and urinary incontinence domains remained relatively stable after PBT compared to the baseline values. These results are in line with our study suggesting that PBT provides acceptable toxicity.
This retrospective study uses a matched-pair analysis that avoids inhomogeneous patient and treatment characteristics between PBT and IMRT in order to reduce selection bias. Moreover, all patients were treated with an endo-rectal balloon that has the same impact on the planning parameters of both treatment modalities. Toxicity and QoL were the same for both groups, making the results easy to compare. A main limitation of this study is the small number of evaluable patient pairs, leading to a low power for detecting small differences between the groups. Exemplary power analyses have been performed to estimate the effect size that may turn out as significant in this study using PASS version 14.0.8 (NCSS, LLC, Kaysville, UT, USA): Assuming a toxicity incidence rate of 30% in one group and 5% in the other group of 20 patients each with α = 0.05 results in a power of 0.55. The incidence rates of late urinary urgency grade ≥1 were close to this showcase and led to a significant difference between IMRT and PBT (). For QoL, a mean difference in QoL score change from baseline of about 15-point scores between IMRT and PBT cohorts and a standard deviation of 20-point scores was assumed. Using a normal approximation, α = 0.05 and 20 patients each, the Mann–Whitney U Test has a power of 0.61. Similar QoL values were observed for constipation at 3 months after RT compared to baseline leading to a statistical trend between IMRT and PBT (). Due to the low patient number, however, toxicity changes below 20% points or QoL changes below half the standard deviation have a low power and will likely not be detected in this study. A further limitation is the difference in the mean prescribed doses of 2.8 Gy between both cohorts. Rescaling may not properly reflect entirely new treatment plans. Moreover, longer follow-up times are necessary to further investigate late GU and GI toxicities, which may become manifest beyond 3 years after RT [Citation42]. For modeling the dose-response and to establish new treatment planning goals for PBT, the number of patients and events has to be increased in further studies. Future prospective trials, possibly including a model-based approach [Citation43], with long-term results are needed to better appreciate the use of PBT as primary treatment in PCA patients.
In this study, PBT seems to be well tolerated compared to IMRT and showed no negative impact on side effects of PCA patients. The QoL changes from baseline were slightly better for constipation at 3 months after PBT whereas global health status was slightly worse at 12 months after PBT compared to IMRT. In general, QoL was similar for both treatment techniques. However, PBT dose distributions and correlations with clinical side effects may differ compared to the photon dose distribution and require further investigation.
Conflict of interest
No potential conflict of interest was reported by the authors.
Supplemental Material
Download PDF (1.5 MB)Disclosure statement
In the past 5 years, Dr. Baumann attended an advisory board meeting of MERCK KGaA (Darmstadt), for which the University of Dresden received a travel grant. He further received funding for his research projects and for educational grants to the University of Dresden by Teutopharma GmbH (2011–2015), IBA (2016), Bayer AG (2016–2018), Merck KGaA (2016–2030) Medipan GmbH (2014–2018).
Dr. Baumann, as former chair of OncoRay (Dresden) and present CEO and Scientific Chair of the German Cancer Research Center (DKFZ, Heidelberg), signed/s contracts for his institute(s) and for the staff for research funding and collaborations with a multitude of companies worldwide.
For the German Cancer Research Center (DKFZ, Heidelberg) Dr. Baumann is on the supervisory boards of HI-STEM gGmbH (Heidelberg).
For this study, Dr. Baumann confirms that none of the above-mentioned funding sources were involved in the study design or materials used, nor in the collection, analysis and interpretation of data nor in the writing of the article.
References
- Mottet N, Bellmunt J, Bolla M, et al. EAU-ESTRO-SIOG guidelines on prostate cancer. Part 1: screening, diagnosis, and local treatment with curative intent. Eur Urol. 2017;71:618–629.
- Yu T, Zhang Q, Zheng T, et al. The effectiveness of intensity modulated radiation therapy versus three-dimensional radiation therapy in prostate cancer: a meta-analysis of the literatures. PLoS One. 2016;11:e0154499.
- Bauman G, Rumble RB, Chen J, et al. Intensity-modulated radiotherapy in the treatment of prostate cancer. Clin Oncol (R Coll Radiol). 2012;24:461–473.
- Yamazaki H, Nakamura S, Nishimura T, et al. Transitioning from conventional radiotherapy to intensity-modulated radiotherapy for localized prostate cancer: changing focus from rectal bleeding to detailed quality of life analysis. J Radiat Res. 2014;55:1033–1047.
- Baumann M, Krause M, Overgaard J, et al. Radiation oncology in the era of precision medicine. Nat Rev Cancer. 2016;16:234.
- Schneider U, Lomax A, Pemler P, et al. The impact of IMRT and proton radiotherapy on secondary cancer incidence. Strahlenther Onkol. 2006;182:647–652.
- Trofimov A, Nguyen PL, Coen JJ, et al. Radiotherapy treatment of early-stage prostate cancer with IMRT and protons: a treatment planning comparison. Int J Radiat Oncol Biol Phys. 2007;69:444–453.
- Vargas C, Fryer A, Mahajan C, et al. Dose–volume comparison of proton therapy and intensity-modulated radiotherapy for prostate cancer. Int J Radiat Oncol Biol Phys. 2008;70:744–751.
- Zips D, Baumann M. Place of proton radiotherapy in future radiotherapy practice. Semin Radiat Oncol. 2013;23:149–153.
- Zelefsky MJ, Chan H, Hunt M, et al. Long-term outcome of high dose intensity modulated radiation therapy for patients with clinically localized prostate cancer. J Urol. 2006;176:1415–1419.
- Dearnaley D, Syndikus I, Mossop H, et al. Conventional versus hypofractionated high-dose intensity-modulated radiotherapy for prostate cancer: 5-year outcomes of the randomised, non-inferiority, phase 3 CHHiP trial. Lancet Oncol. 2016;17:1047–1060.
- Bryant C, Smith TL, Henderson RH, et al. Five-year biochemical results, toxicity, and patient-reported quality of life after delivery of dose-escalated image guided proton therapy for prostate cancer. Int J Radiat Oncol Biol Phys. 2016;95:422–434.
- Schulte RW, Slater JD, Rossi CJ, Jr, et al. Value and perspectives of proton radiation therapy for limited stage prostate cancer. Strahlenther Onkol. 2000;176:3–8.
- Mendenhall NP, Hoppe BS, Nichols RC, et al. Five-year outcomes from 3 prospective trials of image-guided proton therapy for prostate cancer. Int J Radiat Oncol Biol Phys. 2014;88:596–602.
- Iwata H, Ishikawa H, Takagi M, et al. Long-term outcomes of proton therapy for prostate cancer in Japan: a multi-institutional survey of the Japanese Radiation Oncology Study Group. Cancer Med. 2018;7:677–689.
- Sheets NC, Goldin GH, Meyer A, et al. Intensity-modulated radiation therapy, proton therapy, or conformal radiation therapy and morbidity and disease control in localized prostate cancer. JAMA. 2012;307:1611–1620.
- Yu JB, Soulos PR, Herrin J, et al. Proton versus intensity-modulated radiotherapy for prostate cancer: patterns of care and early toxicity. J Natl Cancer Inst. 2013;105:25–32.
- Fang P, Mick R, Deville C, et al. A case-matched study of toxicity outcomes after proton therapy and intensity-modulated radiation therapy for prostate cancer. Cancer. 2015;121:1118–1127.
- Hoppe BS, Michalski JM, Mendenhall NP, et al. Comparative effectiveness study of patient-reported outcomes after proton therapy or intensity-modulated radiotherapy for prostate cancer. Cancer. 2014;120:1076–1082.
- Gray PJ, Paly JJ, Yeap BY, et al. Patient-reported outcomes after 3-dimensional conformal, intensity-modulated, or proton beam radiotherapy for localized prostate cancer. Cancer. 2013;119:1729–1735.
- O’Doherty ÚM, McNair HA, Norman AR, et al. Variability of bladder filling in patients receiving radical radiotherapy to the prostate. Radiother Oncol. 2006;79:335–340.
- Aaronson NK, Ahmedzai S, Bergman B, et al. The European organization for research and treatment of cancer QLQ-C30: a quality-of-life instrument for use in international clinical trials in oncology. J Natl Cancer Inst. 1993;85:365–376.
- van Andel G, Bottomley A, Fosså SD, et al. An international field study of the EORTC QLQ-PR25: a questionnaire for assessing the health-related quality of life of patients with prostate cancer. Eur J Cancer. 2008;44:2418–2424.
- Austin PC. Optimal caliper widths for propensity-score matching when estimating differences in means and differences in proportions in observational studies. Pharmaceut Statist. 2011;10:150–161.
- Fayers P, Aaronson N, Bjordal K, et al. The EORTC QLQ-C30 scoring manual. 3rd ed. Brussels, Belgium: European Organisation for Research and Treatment of Cancer; 2001.
- Osoba D, Rodrigues G, Myles J, et al. Interpreting the significance of changes in health-related quality-of-life scores. J Clin Oncol. 1998;16:139–144.
- Pugh TJ, Munsell MF, Choi S, et al. Quality of life and toxicity from passively scattered and spot-scanning proton beam therapy for localized prostate cancer. Int J Radiat Oncol Biol Phys. 2013;87:946–953.
- Zelefsky MJ, Levin EJ, Hunt M, et al. Incidence of late rectal and urinary toxicities after three-dimensional conformal radiotherapy and intensity-modulated radiotherapy for localized prostate cancer. Int J Radiat Oncol Biol Phys. 2008;70:1124–1129.
- Pan HY, Jiang J, Hoffman KE, et al. Comparative toxicities and cost of intensity-modulated radiotherapy, proton radiation, and stereotactic body radiotherapy among younger men with prostate cancer. J Clin Oncol. 2018;36:1823–1830.
- Zhang X, Dong L, Lee AK, et al. Effect of anatomic motion on proton therapy dose distributions in prostate cancer treatment. Int J Radiat Oncol Biol Phys. 2007;67:620–629.
- Makishima H, Ishikawa H, Tanaka K, et al. A retrospective study of late adverse events in proton beam therapy for prostate cancer. Mol Clin Oncol. 2017;7:547–552.
- Viswanathan AN, Yorke ED, Marks LB, et al. Radiation dose–volume effects of the urinary bladder. Int J Radiat Oncol Biol Phys. 2010;76:S116–S122.
- Marks LB, Yorke ED, Jackson A, et al. The use of normal tissue complication probability (NTCP) models in the clinic. Int J Radiat Oncol Biol Phys. 2010;76:S10–S19.
- Lee WR, Dignam JJ, Amin MB, et al. Randomized phase III noninferiority study comparing two radiotherapy fractionation schedules in patients with low-risk prostate cancer. J Clin Oncol. 2016;34:2325–2332.
- Michalski JM, Gay H, Jackson A, et al. Radiation dose–volume effects in radiation-induced rectal injury. Int J Radiat Oncol Biol Phys. 2010;76:S123–S129.
- Scott N, Fayers P, Aaronson N, et al. EORTC QLQ-C30 reference values manual. 2nd ed. Brussels, Belgium: EORTC Quality of Life Group; 2008.
- Cuypers M, Lamers RED, Cornel EB, et al. The impact of prostate cancer diagnosis and treatment decision-making on health-related quality of life before treatment onset. Support Care Cancer. 2018;26:1297–1304.
- Lips I, Dehnad H, Kruger AB, et al. Health-related quality of life in patients with locally advanced prostate cancer after 76 Gy intensity-modulated radiotherapy vs. 70 Gy conformal radiotherapy in a prospective and longitudinal study. Int J Radiat Oncol Biol Phys. 2007;69:656–661.
- van Leeuwen M, Kieffer JM, Efficace F, et al. International evaluation of the psychometrics of health-related quality of life questionnaires for use among long-term survivors of testicular and prostate cancer. Health Qual Life Outcomes. 2017;15:97.
- Drummond FJ, Kinnear H, O’Leary E, et al. Long-term health-related quality of life of prostate cancer survivors varies by primary treatment. Results from the PiCTure (prostate cancer treatment, your experience) study. J Cancer Surviv. 2015;9:361–372.
- Henderson RH, Hoppe BS, Marcus RB, et al. Urinary functional outcomes and toxicity five years after proton therapy for low- and intermediate-risk prostate cancer: results of two prospective trials. Acta Oncol. 2013;52:463–469.
- Pollack A, Zagars GK, Starkschall G, et al. Prostate cancer radiation dose response: results of the M. D. Anderson phase III randomized trial. Int J Radiat Oncol Biol Phys. 2002;53:1097–1105.
- Langendijk JA, Lambin P, De Ruysscher D, et al. Selection of patients for radiotherapy with protons aiming at reduction of side effects: the model-based approach. Radiother Oncol. 2013;107:267–273.