Abstract
Background: Different modern radiation therapy treatment solutions for breast cancer (BC) and regional nodal irradiation (RNI) have been proposed. In this study, we evaluate the potential reduction in radiation-induced skin morbidity obtained by intensity modulated proton therapy (IMPT) compared with intensity modulated photon therapy (IMXT) for left-side BC and RNI.
Material and Methods: Using CT scans from 10 left-side BC patients, treatment plans were generated using IMXT and IMPT techniques. A dose of 50 Gy (or Gy [RBE] for IMPT) was prescribed to the target volume (involved breast, the internal mammary, supraclavicular, and infraclavicular nodes). Two single filed optimization IMPT (IMPT1 and IMPT2) plans were calculated without and with skin optimization. For each technique, skin dose-metrics were extracted and normal tissue complication probability (NTCP) models from the literature were employed to estimate the risk of radiation-induced skin morbidity. NTCPs for relevant organs-at-risk (OARs) were also considered for reference. The non-parametric Anova (Friedman matched-pairs signed-rank test) was used for comparative analyses.
Results: IMPT improved target coverage and dose homogeneity even if the skin was included into optimization strategy (HIIMPT2 = 0.11 vs. HIIMXT = 0.22 and CIIMPT2 = 0.96 vs. CIIMXT = 0.82, p < .05). A significant relative skin risk reduction (RR = NTCPIMPT/NTCPIMXT) was obtained with IMPT2 including the skin in the optimization with a RR reduction ranging from 0.3 to 0.9 depending on the analyzed skin toxicity endpoint/model. Both IMPT plans attained significant OARs dose sparing compared with IMXT. As expected, the heart and lung doses were significantly reduced using IMPT. Accordingly, IMPT always provided lower NTCP values.
Conclusions: IMPT guarantees optimal target coverage, OARs sparing, and simultaneously minimizes the risk of skin morbidity. The applied model-based approach supports the potential clinical relevance of IMPT for left-side BC and RNI and might be relevant for the setup of cost-effectiveness evaluation strategies based on NTCP predictions, as well as for establishing patient selection criteria.
Introduction
Radiation therapy (RT) following breast conserving surgery is delivered to the whole breast plus or minus to the draining nodes (i.e., supraclavicular, infraclavicular, and internal mammary) in order to reduce breast cancer (BC) recurrence [Citation1], improve disease-free survival, and distant metastasis-free survival [Citation2]. At the same time, regional nodal irradiation (RNI) also may lead to an increase in the complexity of the RT strategy as well as a potential increase in organs-at-risk (OARs) doses and treatment related morbidity, including pneumonitis and, in women with left-sided BC, cardiac diseases and mortality [Citation3,Citation4].
Different advanced RT treatment solutions, such as intensity modulated photon therapy (IMXT) with or without deep inspiration breath-hold (DIBH), for breast and RNI have been evaluated with in silico studies [Citation5–8]. Among the different techniques, the advantages of proton beam therapy have been reported by Ranger et al. [Citation6] in a comprehensive dosimetric analysis showing high levels of conformation able to achieve the most balanced compromise between higher conformation around the target and the lowest OAR doses. In particular, proton therapy seems to be the best technical solution for cardiac sparing when treatment of the regional lymph nodes is indicated [Citation9,Citation10]. However, concerns exist about radiation-induced skin toxicities given the higher skin dose related to a proton beam compared with a photon beam, and different levels of dermatitis have been reported in clinical studies, ranging from 5% to 43% for grade 3 dermatitis [Citation11–13]. Those reported toxicities were mainly obtained by means of passive scattering. Nowadays, with intensity modulated proton therapy (IMPT) delivered with pencil beam scanning (PBS), a decreased dose to skin surface can be expected due to higher flexibility and enhanced modulation capability, as recently investigated in breast treatment without nodal irradiation [Citation14].
Acute skin toxicity is frequent during RT and the severity of this complication can lead to temporary arrest of the RT treatment. Skin toxicity is a common side-effect following BC irradiation, and, although generally of grade 1 or 2, it can often adversely affects patients’ quality of life. Recently, Normal Tissue Complication Probability (NTCP) models for severe acute radiation-induced skin morbidity have been developed for BC patients treated with photons or protons [Citation12,Citation15,Citation16]. These models are based on dose-volume histograms (DVHs) from pseudo-skin structure (a layer of 5 mm from the body contour) [Citation12,Citation15] or are based on skin dose evaluation by dose-surface histograms (DSH) from the body (i.e., the skin) [Citation16].
The consideration of the expected clinical outcome is the basis of the so-called model-based patient selection approach, which has been proposed for a cost-effective identification of patients candidate to receive proton therapy [Citation17,Citation18]. Despite the decreased costs, proton therapy is still more expensive compared with photon RT; therefore, consistent selection criteria are needed. This is especially true when considering the use of protons for a large and differentiate cohort of patients, as for instance those affected by BC [Citation19].
In this framework, it is essential to make treatment decisions based on a careful evaluation of the potential benefits and risks inherent to each RT technique [Citation20]. The present report aims at expanding on the evaluation of proton beam therapy in left-side BC and RNI. More specifically, the study aims at extending the recent investigation on PBS skin-sparing methodologies by a systematic morbidity risk evaluation on a cohort of patients with nodal involvement. Alongside, an ad hoc risk toxicity analysis was performed to guarantee that the skin benefits do not have a detrimental impact on major OARs.
Material and methods
Patients and treatment plans
Ten left-side localized BC patients undergoing postoperative RT after conserving surgery were consecutively enrolled for this in silico study.
Breast target and OARs structures () were contoured on free-breathing CT images according to the RTOG-1005 and AIRO guidelines [Citation21] and heart contouring guidelines [Citation22]. The clinical target volume (CTV) included the breast and the supraclavicular, infraclavicular, and internal mammary nodes. The planning target volume (PTV) was derived by expanding the breast CTV by 1 cm in all directions except the medial (5 mm) and by expanding the nodal CTV by 7 mm in all directions. Breast-PTV was limited anteriorly to exclude the first 5 mm of tissue under the external body surface. In addition, two pseudo-skin structures were defined: one as a 3 mm deep layer (skin3mm) [Citation14] and the other as a 5 mm deep layer (skin5mm) [Citation12,Citation15], both starting from the external body contour and following the extension of the PTV.
Table 1. Planning Target Volume (PTV) and Organs-At-Risk (OAR) planning optimization objectives.
For each patient, four classes of plans were generated: an IMXT plan and three proton plans (IMPT1, IMPT2, and IMPT3 as described below). For all patients, prescription dose was 50 Gy in 2 Gy daily fractions to the PTV with IMXT and 50 relative biological equivalent Gy[RBE] with IMPT. The RBE was considered to be constant and set to 1.1, i.e., D (GyRBE) = 1.1 D (Gy). For brevity, in this paper, we referred only to the unit Gy for proton dose values although they include the RBE corrections.
The IMXT step and shoot plans were calculated with Pinnacle3 TPS V9.8 (Philips Radiation Oncology System, Fitchburg, WI, USA) using the Direct Machine Parameter Optimization (DMPO) technique and collapsed cone convolution (CCC) algorithm setting a dose grid of 0.2 × 0.2 × 0.2 cm3/voxel. The DMPO parameter setting was a maximum number of segments of 50, a minimum segment Monitor Units of 5, a minimum segment area 8 cm2. Photon treatments were designed to deliver six beams equally spaced in the axial plane throughout a 180° sector angle. The first medial beam angle was chosen avoiding direct exposure of the contralateral breast. The beam energy was 6 MV for all beams. For the IMXT linac-plans, the optimization objectives listed in were used. Constraints and weights were adjusted during the optimization process, in order to minimize OAR doses. Two ring structures, with 10 mm expansion inside the external body contour, were created to surround one the breast PTV (ring breast) and the other the nodal PTV (ring LN). These structures aimed at limiting the high doses outside the targets during IMXT optimization.
Protons treatment plans were generated using Raystation 6.0 (RaySearch Laboratories) with a dose grid of 0.2 × 0.2 × 0.2 cm3/voxel. In all plans, a three-beam arrangement (gantry at 340°, 10°, and 60°) was applied using a 4.08 cm (water equivalent thickness) range shifter. Beam energies were in the range from 70 to 160 MeV, i.e., 4.1–17.6 cm in water. All treatment plans were optimized with single field optimization (SFO) approach. A boundary breast-PTV structure was defined as a 5 mm layer, covering the most external portion of the PTV. Each patient had three different proton plans with the same objectives and constraints in the cost-functions () except for the skin3mm and boundary breast-PTV structures which were not included in the cost function for the first plan (IMPT1). In the second plan (IMPT2), the skin3mm structure (corresponding to the average derma thickness) was added in the optimization as an objective at a maximum dose of 30 Gy [Citation16], and the boundary breast-PTV structure was used to keep the dose in the PTV at least equal to the photon plan, in particular in the area of the PTV closest to the skin. Finally, in the third plan (IMPT3), the dose to the skin3mm structure was forced to be equal to the IMXT plan by means of objectives on D10%, D20%, D30%, …, D90% ().
Plan acceptance criteria were defined according to . Plans were considered acceptable if at least 95% of PTV received at least 90% of prescription dose.
Dosimetric analysis
For each patient, individual DICOM RT plans were converted into Matlab-readable format (Mathworks, Natick, MA) using CERR (Computational Environment for Radiotherapy Research) [Citation23], and DVHs from planning data were extracted. Relevant DVH metrics for PTV and OARs were analyzed: the percentage volume receiving dose (Vx); near maximum dose (D2%); near minimum dose (D98%); mean dose (Dmean), and median dose (Dmed). In addition, the DSH of the body were computed as representative of skin irradiation [Citation16,Citation24] and DSH metrics extracted.
PTV coverage was assessed via the conformity index (CI = V95%/PTVvol) and the homogeneity index (HI = [D2% – D98%]/Dmed).
Normal tissue complication probability and statistical analysis
Toxicity risks for radiation-induced skin toxicity in BC patients were calculated according to recently published NTCP models [Citation15,Citation16].
NTCP values for lung, heart, and thyroid [Citation3,Citation4,Citation16,Citation25–27] were also computed in order to perform a comprehensive risk evaluation.
The relative risk (RR = NTCPIMPT/NTCPIMXT) ratios for IMPT1 and IMPT2 plans compared with photons were accordingly calculated.
The median and the range were employed to describe all continuous variables and the non-parametric Wilcoxon or ANOVA tests (Friedman matched-pairs signed-rank test) were used to determine any significant statistical differences (p < .05).
Results
Median target (breast PTV and nodal PTV) size was 1061.2 cm3 (range, 609.0–1117.8). All plans fulfilled the clinical dose criteria set for PTV/OARs. Dose distribution indices for the target and pseudo target volumes (i.e., skin3mm and boundary breast-PTV structure) showed a satisfactory PTV coverage for all techniques ( and ). However, all IMPT solutions in all patients achieved greater PTV coverage with superior dose homogeneity (maximum HI = 0.16 vs. 0.21 and minimum CI = 0.88 vs. 0.81) compared with IMXT ( and ), even when the skin was included into proton optimization strategy (HIIMPT2 = 0.11 and CIIMPT2 = 0.96). In particular, IMPT3 plans allow to obtain the same dose coverage on the skin-like structure as in the IMXT plan, but with an increased PTV coverage with respect to IMPT2, mainly in the boundary breast-PTV structure (). Both IMPT2 and IMPT3 allowed reducing the body surface mean doses () to levels lower than those obtained with IMXT. shows the comparison of dose distributions in two representative patients from IMXT, IMPT1, and IMPT2 treatment plans.
Figure 1. Median cumulative patients dose volume histograms for the PTV (a) and median cumulative patient dose–surface histogram for the skin (b).
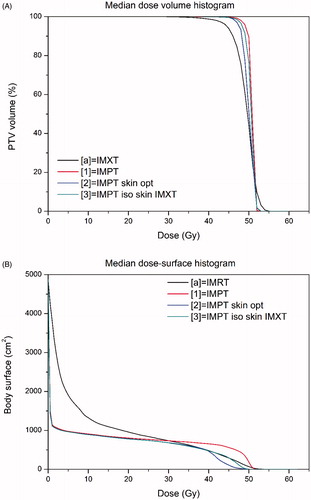
Figure 2. Comparison of intensity modulated photon therapy (IMXT) plan (left), intensity modulated proton (IMPT1) therapy (middle), and IMPT2 (right) dose distributions in two representative patients.
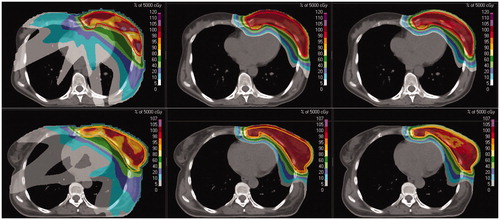
Table 2. Dose distribution indices for the PTV and pseudo target volumes (Skin3mm and superficial PTV-breast structures).
Table 3. Dosimetric parameters and comparative analysis for different organs for intensity modulated photon (IMXT) and intensity modulated proton without (IMPT1) and with skin included in the cost function (IMPT2).
Concerning normal tissue, OAR dose metrics in IMPT3 always show intermediate values between IMPT1 and IMPT2 ( and ), thus no further analysis was performed on IMPT3 plans.
As to skin sparing, if compared with IMPT1 and IMXT plans, IMPT2 significantly reduced different dose metrics extracted from both skin and pseudo skin structures ().
In particular, a reduction of about a factor 5 and 10 in the heart mean dose and left ventricle mean dose was observed when using protons independently of the specific IMPT plan. At the same time, a reduction of a factor 2 or 3 was obtained in the case of left lung dose metrics.
Beyond skin dose sparing, our analysis also showed that the specific IMPT configuration adopted did not influence dose sparing for major OARs compared with IMXT. Of note, although IMPT2, compared with IMPT1, shows an additional significant sparing for all OARs with the exception of the thyroid, this gain was not particularly relevant if normalized to the variance of the analyzed metrics.
Dosimetric analysis was combined with toxicity risk models ( and ) in order to predict RR ratios () for proton plans compared with photons.
Figure 3. Patients normal tissue complication probability (NTCP), odds ratio (OR), and hazard ratio (HR) values for different organs and endpoints for intensity modulated photon therapy (IMXT) and for intensity modulated proton therapy plans without (IMPT1) or with skin included in the cost function (IMPT2).
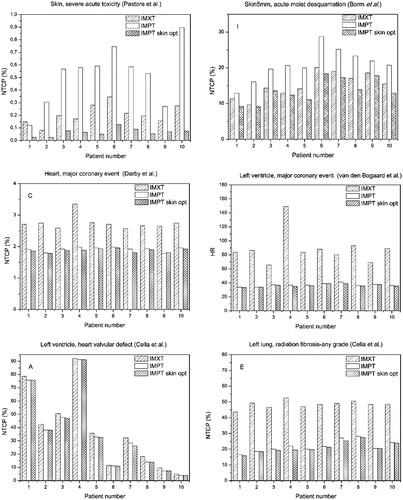
Table 4. Relative risk (RR) ratios comparison according to normal tissue complication probability analysis for severe acute skin toxicity for intensity modulated proton plan without (IMPT1) and with skin included in the cost function (IMPT2).
Using skin NTCP models, the RR of severe skin toxicity turns out to be overall higher than 1 for IMPT1 plans, even though a large variability range was observed among patients, while a significant RR reduction was obtained with IMPT2 including skin in the optimization ().
Figure 4. Relative risk (RR) ratio comparison for skin morbidity according to different normal tissue complication probability (NTCP) models for intensity modulated proton plans without (IMPT1) and with skin included in the cost function (IMPT2).
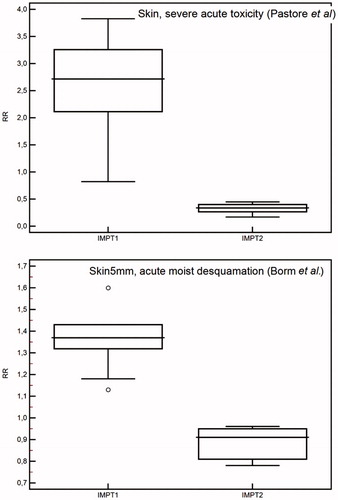
The application of NTCP models to cardiac, lung, and thyroid complications showed an overall reduction in the toxicity risk prediction for both IMPT1 and IMPT2 plans compared with IMXT plan, with RR ranging from 0.3 to 0.9 depending on the considered toxicity endpoint. As outlined in , reduced risk for such endpoints was registered for all patients, also indicating that the RR variability among the patients is much less than that due to the different models or specific endpoint considered.
Discussion
The unique physical properties of protons can be exploited to improve the treatment of BC reducing normal tissue doses without compromising target coverage. This is not a novelty and it has been discussed and demonstrated in silico since the first studies in the early 2000s [Citation28–30]. However, the actual clinical use of PT for the treatment of the whole breast is gaining momentum thanks to the recent gradual spread of proton therapy centers and to the increasing use of PBS techniques and optimized IMPT [Citation31–33]. There are at least eight clinical trials currently investigating the use of proton therapy for the treatment of the whole breast with or without nodal irradiation [Citation34]. However, the risks of a potential increase in skin toxicity have long been considered as a potential limiting factor in the clinical use of protons. A recently published review by Verma et al. [Citation35] showed that, according to the limited number of available clinical data, the risk of acute skin toxicities for proton therapy might be similar to that observed after conventional RT or even lower. Those reported clinical data were mainly obtained by means of passive scattering. Nowadays with proton PBS becoming more accessible, a reduction can be expected in the dose released to the skin as a consequence of higher flexibility and enhanced modulation capability in treatment planning. Such potential has been only recently investigated for skin sparing in whole breast irradiation without nodal involvement [Citation14].
In the present study, we extended the analysis to investigate the potential of IMPT in skin sparing when also regional nodes were included in the target volume. Three different IMPT plans were implemented on purpose. A first plan was generated without including the skin in the optimization (IMPT1), a second plan with the skin-like structure added in the cost function (IMPT2), and a third one (IMPT3), which used as benchmark the IMXT plan.
Our results confirmed that the dose to the skin could be modulated in PBS at the same time achieving adequate target coverage. Furthermore, thanks to IMPT flexibility, we were able to achieve two scenarios in terms of skin sparing and target coverage: IMPT3 plans allow to achieve the same level of skin toxicity as IMXT, with an improved target coverage; IMPT2 plans resulted into a reduction of acute skin toxicity risk compared with IMXT with a minimal loss in terms of CI and HI when compared to IMPT3. The RR reduction of radiation-induced skin toxicity obtained with IMPT2 ranges from 0.3 to 0.9 depending on the analyzed toxicity endpoint and applied model.
Severe acute skin reactions may be prodromal of consequential skin late effects [Citation36] and prevention of these acute reactions may also avoid late effects which can impact on esthetic outcome and quality of life. Recently Kindts et al. [Citation37] developed a prediction model for late unfavorable esthetic outcome for breast-conserving therapy taking into account clinicopathological and dosimetric variables. The authors identified seroma, axillary lymphadenectomy, and breast volume receiving more than 55 Gy as predictive factors. However, in our in silico dosimetric analysis, the target volume receiving more than 55 Gy was negligible and consequently the model was not applicable.
Concerning cardiac toxicity, patients successfully treated with RT for BC have still an increased risk of developing subsequent heart disease, although lower than in earlier eras [Citation38]. The risk of heart disease is higher for women who received RT for left-sided BC compared with women who received right-sided RT [Citation39] and even higher if the internal mammary chain is irradiated [Citation10]. By applying different models on radiation-associated cardiovascular toxicity that have been published since 2009, i.e., in the post QUANTEC era, we obtained different risk estimates ( ) with different potential gains in RR reduction by IMPT plans, according to the specific clinical endpoint. Of note, all simulated plans fulfilled the predefined acceptance criteria and doses to heart substructures, such as ventricles, were not included per protocol as constraints.
Interestingly, if we compare the risk estimates for lung and heart of the present analysis on breast and RNI with those previously reported [Citation14] for breast irradiation, values generally increase for both IMXT and IMPT, being in any case significantly lower in the IMPT plan. This is a consequence of the more complicated target geometry. Specifically, RNI results into an increased risk of valvular defects for both photon and proton irradiation. On the contrary, the risk of major coronary events with RNI is almost doubled for photons, while a minor increase is obtained with protons. A similar trend is also observed for the induction of lung fibrosis.
The results obtained in our study hold when we compare IMPT with IMXT without the use of breath-control techniques. The recent in silico study on BC with nodal involvement by Rangers et al. [Citation6] confirmed that the mean dose to heart and lung in photon RT could be reduced by the use of DIBH, especially when combined with VMAT techniques. This reduces the dosimetric advantages offered by IMPT which nevertheless remains the technique that allows to minimize the dose to distal OARs for such patients. Interestingly, the advantages offered by IMPT seem not to be affected by the use of DIBH [Citation6]. These results clearly indicate that the NTCP reduction expected with IMPT should also be reconsidered when comparing it to different IMXT techniques. At the same time, they suggest that the combination of dosimetric and NTCP analysis with technical considerations could help identifying a well-defined subpopulation of BC patients for which IMPT might represent the ideal treatment. For instance, this might be the case for young patients (age < 40 years old) due to the reduced cardiac morbidity and secondary cancer risks (i.e., reduced dose to the contra-lateral breast), for patients not tolerating DIBH as well as for those patients for which anatomy is responsible for a larger dosimetric and NTCP gain.
Notably, even though motion management DIBH does not significantly impact on the sparing of distal OARs, it is important to properly take into account the motion management when modeling dose to the skin. Even a displacement of few millimeters could cause significant differences in dose when using a scanning technique. In this regard, surface imaging systems such as those recently proposed by Batin et al. [Citation40,Citation41] can be valuable for a more accurate patient positioning.
In conclusion, our study confirms that the dosimetric advantages offered by IMPT over IMXT for the treatment of BC with nodal involvement translates into a significant NTCP reduction of cardiac and pulmonary toxicities. This also holds for the risk of severe acute skin toxicity, which can be maintained at values comparable with IMXT, without compromising target coverage. These data contribute to quantify the clinical benefit offered by IMPT, providing valuable information for the setup of cost-effective patient selection criteria for BC patients.
Ethics approval and consent to participate
This is a retrospective in silico study. The study follows the principles of the Declaration of Helsinki. All included patients gave written informed consents for data analysis, all patients’ health information data were de-identified for analysis.
Supplemental Material
Download MS Word (22.3 KB)Disclosure statement
No potential conflict of interest was reported by the authors.
Additional information
Funding
References
- Whelan TJ, Olivotto IA, Parulekar WR, et al. Regional nodal irradiation in early-stage breast cancer. N Engl J Med. 2015;373:307–316.
- Poortmans PM, Collette S, Kirkove C, et al. Internal mammary and medial supraclavicular irradiation in breast cancer. N Engl J Med. 2015;373:317–327.
- Darby SC, Ewertz M, McGale P, et al. Risk of ischemic heart disease in women after radiotherapy for breast cancer. N Engl J Med. 2013;368:987–998.
- van den Bogaard VA, Ta BD, van der Schaaf A, et al. Validation and modification of a prediction model for acute cardiac events in patients with breast cancer treated with radiotherapy based on three-dimensional dose distributions to cardiac substructures. JCO. 2017;35:1171–1178.
- Ares C, Khan S, Macartain AM, et al. Postoperative proton radiotherapy for localized and locoregional breast cancer: potential for clinically relevant improvements? Int J Radiat Oncol Biol Phys. 2010;76:685–697.
- Ranger A, Dunlop A, Hutchinson K, et al. A dosimetric comparison of breast radiotherapy techniques to treat locoregional lymph nodes including the internal mammary chain. Clin Oncol. (R Coll Radiol) 2018;30:346–353.
- Tyran M, Mailleux H, Tallet A, et al. Volumetric-modulated arc therapy for left-sided breast cancer and all regional nodes improves target volumes coverage and reduces treatment time and doses to the heart and left coronary artery, compared with a field-in-field technique. J Radiat Res. 2015;56:927–937.
- Lancellotta V, Iacco M, Perrucci E, et al. Comparing four radiotherapy techniques for treating the chest wall plus levels III-IV draining nodes after breast reconstruction. Br J Radiol. 2018;91:20160874.
- Braunstein LZ, Cahlon O. Potential morbidity reduction with proton radiation therapy for breast cancer. Semin Radiat Oncol. 2018;28:138–149.
- Kammerer E, Guevelou JL, Chaikh A, et al. Proton therapy for locally advanced breast cancer: a systematic review of the literature. Cancer Treat Rev. 2018;63:19–27.
- Bradley JA, Dagan R, Ho MW, et al. Initial report of a prospective dosimetric and clinical feasibility trial demonstrates the potential of protons to increase the therapeutic ratio in breast cancer compared with photons. Int J Radiat Oncol Biol Phys. 2016;95:411–421.
- Liang X, Bradley JA, Zheng D, et al. Prognostic factors of radiation dermatitis following passive-scattering proton therapy for breast cancer. Radiat Oncol. 2018;13:72.
- Verma V, Iftekaruddin Z, Badar N, et al. Proton beam radiotherapy as part of comprehensive regional nodal irradiation for locally advanced breast cancer. Radiother Oncol. 2017;123:294–298.
- Tommasino F, Durante M, D'Avino V, et al. Model-based approach for quantitative estimates of skin, heart, and lung toxicity risk for left-side photon and proton irradiation after breast-conserving surgery. Acta Oncol. 2017;56:730–736.
- Borm KJ, Loos M, Oechsner M, et al. Acute radiodermatitis in modern adjuvant 3D conformal radiotherapy for breast cancer – the impact of dose distribution and patient related factors. Radiat Oncol. 2018;13:218.
- Pastore F, Conson M, D’Avino V, et al. Dose-surface analysis for prediction of severe acute radio-induced skin toxicity in breast cancer patients. Acta Oncol. 2016;55:466–473.
- Langendijk JA, Lambin P, De Ruysscher D, et al. Selection of patients for radiotherapy with protons aiming at reduction of side effects: the model-based approach. Radiother Oncol. 2013;107:267–273.
- Widder J, van der Schaaf A, Lambin P, et al. The quest for evidence for proton therapy: model-based approach and precision medicine. Int J Radiat Oncol Biol Phys. 2016;95:30–36.
- Marvaso G, Vischioni B, Jereczek-Fossa BA, et al. Hadrontherapy from the Italian Radiation Oncologist point of view: face the reality. The Italian Society of Oncological Radiotherapy (AIRO) survey. Radiol Med. 2017;122:140–145.
- Moreno AC, Shaitelman SF, Buchholz TA. A clinical perspective on regional nodal irradiation for breast cancer. Breast. 2017;34(Suppl 1):S85–S90.
- AIRO. La Radioterapia dei Tumori della Mammella: Indicazioni e Criteri Guida [cited 2013]. Available from: http://vecchio.radioterapiaitalia.it/cont__169.phtml
- Feng M, Moran JM, Koelling T, et al. Development and validation of a heart atlas to study cardiac exposure to radiation following treatment for breast cancer. Int J Radiat Oncol Biol Phys. 2011;79:10–18.
- Deasy JO, Blanco AI, Clark VH. CERR: a computational environment for radiotherapy research. Med Phys. 2003;30:979–985.
- Palma G, Cella L. A new formalism of dose surface histograms for robust modeling of skin toxicity in radiation therapy. Phys Med. 2019;59:75–78.
- Cella L, D’Avino V, Palma G, et al. Modeling the risk of radiation-induced lung fibrosis: irradiated heart tissue is as important as irradiated lung. Radiother Oncol. 2015;117:36–43.
- Cella L, Liuzzi R, Conson M, et al. Multivariate normal tissue complication probability modeling of heart valve dysfunction in Hodgkin lymphoma survivors. Int J Radiat Oncol Biol Phys. 2013;87:304. 10.
- Cella L, Liuzzi R, Conson M, et al. Development of multivariate NTCP models for radiation-induced hypothyroidism: a comparative analysis. Radiat Oncol. 2012;7:224.
- Johansson J, Isacsson U, Lindman H, et al. Node-positive left-sided breast cancer patients after breast-conserving surgery: potential outcomes of radiotherapy modalities and techniques. Radiother Oncol. 2002;65:89–98.
- Fogliata A, Bolsi A, Cozzi L. Critical appraisal of treatment techniques based on conventional photon beams, intensity modulated photon beams and proton beams for therapy of intact breast. Radiother Oncol. 2002;62:137–145.
- Lomax AJ, Cella L, Weber D, et al. Potential role of intensity-modulated photons and protons in the treatment of the breast and regional nodes. Int J Radiat Oncol Biol Phys. 2003;55:785–792.
- Lomax A. Intensity modulation methods for proton radiotherapy. Phys Med Biol. 1999;44:185–205.
- Cella L, Lomax A, Miralbell R. New techniques in hadrontherapy: intensity modulated proton beams. Phys Med. 2001;17:100–102.
- Mohan R, Grosshans D. Proton therapy – present and future. Adv Drug Deliv Rev. 2017;109:26–44.
- Verma V, Simone CB II, Mishra MV. Quality of life and patient-reported outcomes following proton radiation therapy: a systematic review. J Natl Cancer Inst. 2018;110:341–353.
- Verma V, Shah C, Mehta MP. Clinical outcomes and toxicity of proton radiotherapy for breast cancer. Clin Breast Cancer. 2016;16:145–154.
- Dorr W, Hendry JH. Consequential late effects in normal tissues. Radiother Oncol. 2001;61:223–231.
- Kindts I, Defraene G, Laenen A, et al. Development of a normal tissue complication probability model for late unfavourable aesthetic outcome after breast-conserving therapy. Acta Oncol. 2018;57:916–923.
- Brenner DJ, Shuryak I, Jozsef G, et al. Risk and risk reduction of major coronary events associated with contemporary breast radiotherapy. JAMA Intern Med. 2014;174:158–160.
- Rehammar JC, Jensen MB, McGale P, et al. Risk of heart disease in relation to radiotherapy and chemotherapy with anthracyclines among 19,464 breast cancer patients in Denmark, 1977–2005. Radiother Oncol. 2017;123:299–305.
- Batin E, Depauw N, Jimenez RB, et al. Reducing X-ray imaging for proton postmastectomy chest wall patients. Pract Radiat Oncol. 2018;8:e266–e274.
- Batin E, Depauw N, MacDonald S, et al. Can surface imaging improve the patient setup for proton postmastectomy chest wall irradiation? Pract Radiat Oncol. 2016;6:e235–e241.